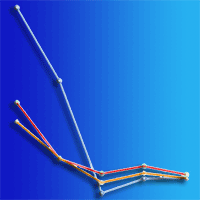
Wildfire and harvesting effects on carbon dynamics in an oak-pine mixed forest
iForest - Biogeosciences and Forestry, Volume 13, Issue 5, Pages 435-440 (2020)
doi: https://doi.org/10.3832/ifor3312-013
Published: Sep 16, 2020 - Copyright © 2020 SISEF
Research Articles
Abstract
CO2 emission to the atmosphere is the main cause of global warming. The impacts of land-use changes for agriculture and urbanisation, deforestation, and fire disturbance are attributed to the increase in CO2 emissions. Soil respiration, largely due to microbial activity, is one of the CO2 sources being released to the atmosphere. In this regard, several soil parameters related with carbon cycle, including organic matter, total N, C/N ratio, CO2 efflux, microbial biomass C (Cmic), the Cmic/Corg ratio, the metabolic quotient qCO2, and β-D glucosidase activity, were determined in a burned (harvested, H; non-harvested, NH), and its adjacent unburned (UB), mixed oak-pine forest to estimate the effects of burning and removal of residual woods. The Cmic increased gradually with burning and harvesting after Month 9, and sharp increases were observed in all areas, likely due to the abundant rainfall after Month 12. CO2 efflux decreased in the burned areas at Months 4 and 6; however, this reversed in Month 9. In spite of non-significant differences, we detected higher CO2 efflux values in the unburned areas compared to the burned ones, probably as a result of the drought effect observed in the burned areas up to Months 9 and 12 due to the increased soil heat. There was no significant difference between the H and NH burned areas, while both areas were different from the unburned areas in all soil parameters, except CO2 efflux and qCO2. The harvesting effect was not significant compared to the fire effect with regard to the considered soil variables, likely due to the management and protection of the burned area which allowed a fast vegetation recover. The abundance of the microbial biomass was independent of the changes in CO2 efflux and showed a negative correlation with β-D glucosidase activity. This might be related to the variation in substrate quality, microbial composition and abundance after burning and harvesting.
Keywords
CO2 Evolution, β-D Glucosidase Activity, qCO2, Soil Microbial Biomass Carbon, Wildfire
Introduction
The increase in C stocks in forest ecosystems depends on the preservation of C in tree biomass and soil against degradation. Wildfire and harvesting both affect the soil organic carbon storage by combusting organic matter, volatilization, changing soil structure, vegetation canopy, microclimatic conditions, erosion, litter layer composition, microbial population and their activity, and decomposition ([49], [29], [35], [42], [33], [1]). Fire may accelerate the decomposition of organic matter (OM), promoting CO2 emission. Logging and removal of burned trees increase the CO2 emissions (about 120 gr C m-2 in burned pine forests) and reduce the renovation capacity of Mediterranean ecosystems after the wildfire ([46]). On the other hand, though no significant effect on total ecosystem carbon stocks was observed, a decrease was seen in carbon stored in snags and down woody material after the salvage logging in burned sub-boreal jack pine forests ([10]) The soil microflora contributes to soil C storage, soil respiration and ecosystem productivity, despite representing only a small portion of the soil ([6]). Microbial biomass C (Cmic) has been suggested as an indicator of changes in the soil ([6]) as a consequence of forest management ([5], [41], [40]). Fire may alter the microbial abundance both directly by killing microbes soil surface due to heating and indirectly by modifying the community composition and environmental conditions ([13], [20], [56]). Additionally, harvesting may affect these conditions by compacting the soil and causing increases in runoff rates and erosion ([36], [15]). Decreases were found in microbial biomass carbon after harvest (18%), burn (74%), and burn-salvage (53%) treatments ([49]).
The metabolic quotient (qCO2), which is the microbial respiration/microbial biomass C ratio, decreases with increasing the quality of substrate, and increases under unfavourable conditions ([3], [54], [6], [32]). Microbial parameters, including Cmic, qCO2 and qC, have been used in several studies ([37]) in order to understand the impacts of soil management or disturbance.
Soil microorganisms apply different strategies to use available C, such as biotic (e.g., release of extracellular enzymes) and abiotic (e.g., redox and metal complexation) mechanisms ([22]). β-D glucosidase which takes part in the degradation of cellulose, decreased in burned soils, as reported by Hernandez et al. ([21]), but little is known about the harvesting effect of burned residues on this enzyme activity.
The purpose of this study was to understand the relationship between the C dynamics and the microbial activity after a wildfire, and after harvesting of the residues, by using several microbial parameters, including CO2 evolution, Cmic, qCO2, and β-D glucosidase enzyme activity. It was hypothesized that burning alters the microbial activity related to C dynamics, and harvesting contributes to these changes.
Materials and methods
Study area and soil sampling
We conducted this study in a mixed pine (Pinus nigra Arnld. subsp. pallasiana Lamb.) and oak (Quercus pubescens Wild.) forest in Safranbolu, Turkey (41° 16′ 11″ N, 32° 37′ 41″ E) following a wildfire occurred on the 9th and 10th of October, 2011. The fire was caused by a picnic fire, and affected an area of 5 hectares. Fire severity was estimated based on the litter consumption ([44]) and whether the ashes whiten or not ([29]). The severity of the fire ranged from low to medium due to black ash and bare soil surface dominated the majority of the site. Additionally, this determination also complies with the criteria of Ryan & Noste ([44]) modified from Ryan ([45]) and Turner et al. ([52]) ([30]). We did not measure the percentage of vegetatition cover, but based on our observation the understory was mainly composed of Phillyrea latifolia L., Cistus creticus L., Juniperus oxycedrus L., Colutea cilicica Boiss. & Balansa, and Rubus sp. The annual rainfall was between 630 and 650 mm in the region during the study period. The elevation of the area was 720-760 m a.s.l., and the slope 30-45%. The soil with typical A-C horizons belongs to the order intrazonal and suborder calsimorfic and was classified as a rendzina, according to FAO/UNESCO ([25], [50]). The main characteristics of the soils in the study area are given in Tab. 1. The area was covered with residues, consisting of twigs, for the protection of the soil and the new generation. In several parts of the burnt area the residual trees were removed (harvested).
Tab. 1 - Main soil characteristics in the study area. (EC): Electrical conductivity.
- | Variable | Profile horizons | |||
---|---|---|---|---|---|
Ah | C1 | C2 | C3 | ||
Physical analysis | Depth (cm) | 0-2 | 2-36 | 36-46 | 46-83 |
Total weight (g) | 1614.48 | 1946.15 | 1740.84 | 2080.9 | |
Gravel weight (g) | 211.48 | 389.51 | 377.71 | 546.70 | |
Gravel volume (ml) | 87.50 | 158.33 | 155.00 | 240.00 | |
Sand (%) | 29.41 | 37.07 | 36.97 | 42.36 | |
Silt (%) | 29.63 | 26.95 | 21.47 | 26.42 | |
Clay (%) | 40.97 | 35.99 | 41.56 | 31.22 | |
Soil texture | Silty clay loam | Silty clay loam | Clay loam | Clay loam | |
Chemical analysis | pH 1:2.5 | 7.2 | 7.5 | 7.6 | 7.7 |
Total lime (%) | 2.915 | 14.84 | 28.09 | 24.11 | |
Organic Matter, OM (%) | 6.88 | 1.65 | 1.07 | 0.87 | |
Total Nitrogen, Nt (%) | 0.39 | 0.09 | 0.06 | 0.04 | |
P2O5 (mg kg-1) | 9.73 | 16.8 | 11.2 | 9.05 | |
EC (×103, mS cm-1) | 0.70 | 0.59 | 0.41 | 0.71 |
Soil sampling was conducted in Months 4, 6, 9, 12 and 24 after the fire. We selected 22 plots (10 × 10 m2) including eight burned-non harvested (NH), eight burned-harvested (H) and three adjacent, unburned areas for each species. Three soil samples per plot were collected at depths of 0-7.5 cm, following removal of the surface residues, including oak leaves and pine needles. We collected the samples from low- and medium-severity burned areas in order to achieve homogeneity. Soil samples were transferred to the laboratory, sieved through a 2-mm mesh and stored at +4 °C.
Laboratory analyses
The CO2 evolution was determined according to the method of Isermeyer ([24]), modified by Alef ([2]). Following incubation of the samples with NaOH traps for 24 hours at 25 °C in sealed containers, the remaining NaOH was titrated with HCl after adding BaCl2 solution and phenolphthalein. The CO2 flux was calculated from the amount of consumption of HCl through titration given by the following formula (eqn. 1):
where SW is the amount of soil dry weight, t is the incubation time, V0 is the HCl used for titration, V is the HCl used for soil sample, dwt is the dry weight of 1 g moist soil, and 1.1 is the conversion factor (i.e., 1 ml 0.05 M NaOH equals 1.1 mg CO2).
The Cmic was determined according to the chloroform extraction method described by Vance et al. ([53]). Half of each sample was fumigated with CHCl3 and then incubated at 25 °C for 24 h. The fumigated and non-fumigated samples were extracted using 0.5 M K2SO4 at a soil/extract ratio of 1:4, and then kept at -20 °C until they were analysed using a TOC-L® analyser (Shimadzu Corp., Kyoto, Japan). The Cmic was calculated following Wu et al. ([55]) using the following equation (eqn. 2):
where Ec is the difference between the amount of Corg extracted from the fumigated and non-fumigated soils.
The qCO2 (metabolic quotient) is a specific respiration rate of CO2 C evolved per unit of Cmic, which is calculated from CO2 evolution and Cmic C.
The β-D glucosidase activity was measured according to the method of Naseby & Lynch ([38]). A 1.5 g aliquot of soil was extracted using an acetate buffer at pH 5.5, agitated on an orbital rotary shaker with a slope angle of 60° to the horizontal for 1 h, and then centrifuged at 4000 rpm for 15 min. Following incubation at 37 °C for 24 h with buffered substrate p-nitrophenyl-β-D glucoside, 1 ml NaOHCO3 was added to the extracts, which were then quantified at 400 nm in a spectrophotometer.
Statistical analyses
Differences between the burned (H and NH) and unburned soil samples were determined by ANOVA for each period and in total. Data was transformed when it was not normally distributed as log (1+ √qCO2), or alternatively a non-parametric test (Mann-Whitney U) was used. Correlations between the parameters were estimated. All statistical analyses were performed using the software SPSS® v. 21 (IBM, Armonk, NY, USA).
Results
We observed significant differences in most of the soil samples in terms of burning, while the harvested and non-harvested samples were similar for most of the variables analysed. A significant decrease was determined in Corg in burned samples but the results were relatively higher in burned-H samples. No difference in total nitrogen (Nt) was found between the unburned and burned soils but the Month 9. The C/N ratios were slightly different in favor of the unburned samples (p = 0.05 - Fig. 1a, Tab. 2). There was no significant difference in CO2 evolution (p = 0.956); however, the maximum values were observed in the unburned soils, and the minimum values were found in the burned-NH soils (Tab. 2). The Cmic values were different at Month 12 (p = 0.036) while it was comparatively high in the burned areas at all months (Fig. 1b, Tab. 2). We observed significant differences (p = 0.029) in Cmic/Corg at Month 12 similarly (Fig. 1c, Tab. 2). An increase in the total amount of Cmic in all areas was observed through the time, but the increase was higher in the burned areas between Months 12 and 24.
Fig. 1 - (a) C/N ratio, (b) Cmic values, (c) Cmic/Corg ratio, (d) β-D glucosidase activity, and (e) qCO2 ratios in burned (H: harvested; NH: non-harvested) and unburned areas.
Tab. 2 - Means (± standard error) of the soil parameters analysed for different periods after fire (Month) and level of disturbance (Dist). Different letters indicate significant differences (p<0.05) between unburned (UB), burned non-harvested (B-NH) and burned harvested (B-H) areas. (OM): organic matter (%); (Nt): total nitrogen; (Cmic): microbial carbon (µg C g-1); (Corg): organic carbon; (β-DG): β-D glucosidase (mg pNP h-1 g-1); (N): sample size.
Month | Dist | OM (%) |
Nt (%) |
C/N ratio |
CO2 efflux (mg CO2 g-1 h-1) |
Cmic (µg C g-1) |
Cmic/Corg ratio |
qCO2 (mg CO2 g-1 Cmic h-1) |
β-DG (mg pNP h-1 g-1) |
N |
---|---|---|---|---|---|---|---|---|---|---|
4 | UB | 6.09 ± 1.48 a | 0.26 ± 0.07 a | 14 ± 1 b | 19.81 ± 6.11 a | 3760 ± 2239 a | 0.07 ± 0.04 a | 7.12 ± 5.23 b | 1.49 ± 0.32 a | 6 |
B-NH | 5.49 ± 1.72 a | 0.27 ± 0.09 a | 12 ± 1 a | 13.45 ± 2.88 a | 3862 ± 1200 a | 0.07 ± 0.02 a | 3.84 ± 1.52 a | 1.22 ± 0.97 a | 8 | |
B-H | 6.50 ± 1.60 a | 0.29 ± 0.09 a | 13 ± 2 ab | 14.36 ± 9.89 a | 4251 ± 1489 a | 0.07 ± 0.02 a | 3.18 ± 0.96 a | 1.12 ± 0.84 a | 8 | |
Total | 6.02 ± 1.60 | 0.28 ± 0.08 | 13 ± 1 | 15.51 ± 7.19 | 3976 ± 1568 | 0.07 ± 0.02 | 4.50 ± 3.22 | 1.26 ± 0.77 | 22 | |
6 | UB | 6.57 ± 1.22 b | 0.25 ± 0.08 a | 17 ± 8 a | 8.88 ± 2.15 b | 3703 ± 2533 a | 0.05 ± 0.03 a | 4.60 ± 4.45 a | 3.08 ± 3.32 a | 6 |
B-NH | 4.14 ± 0.84 a | 0.19 ± 0.04 a | 13 ± 4 a | 5.52 ± 1.06 a | 3555 ± 1777 a | 0.09 ± 0.04 a | 1.91 ± 1.05 a | 1.84 ± 1.94 a | 8 | |
B-H | 5.15 ± 2.18 ab | 0.24 ± 0.06 a | 12 ± 3 a | 6.53 ± 2.20 ab | 4393 ± 3056 a | 0.10 ± 0.07 a | 1.95 ± 0.85 a | 1.17 ± 0.85 a | 8 | |
Total | 5.17 ± 1.77 | 0.23 ± 0.06 | 14 ± 5 | 6.80 ± 2.23 | 3900 ± 2417 | 0.08 ± 0.05 | 2.66 ± 2.61 | 1.93 ± 2.17 | 22 | |
9 | UB | 8.37 ± 3.16 b | 0.43 ± 0.17 b | 11 ± 0 a | 1.96 ± 0.93 a | 3276 ± 1872 a | 0.04 ± 0.01 a | 0.73 ± 0.41 a | 3.01 ± 1.36 b | 6 |
B-NH | 5.37 ± 1.40 a | 0.27 ± 0.07 a | 11 ± 0 a | 3.70 ± 1.58 a | 3357 ± 1428 a | 0.06 ± 0.02 a | 1.40 ± 1.10 a | 1.22 ± 1.06 ab | 8 | |
B-H | 5.73 ± 2.55 ab | 0.29 ± 0.13 ab | 11 ± 0 a | 3.25 ± 2.03 a | 3522 ± 1590 a | 0.07 ± 0.04 a | 1.09 ± 0.82 a | 1.05 ± 0.64 a | 8 | |
Total | 6.32 ± 2.62 | 0.32 ± 0.14 | 11 ± 0 | 3.06 ± 1.71 | 3395 ± 1539 | 0.06 ± 0.03 | 1.11 ± 0.86 | 1.65 ± 1.30 | 22 | |
12 | UB | 6.18 ± 1.11 a | 0.24 ± 0.05 a | 15 ± 2 a | 4.21 ± 1.30 a | 3575 ± 7880 a | 0.06 ± 0.02 a | 1.23 ± 0.51 a | 1.40 ± 0.36 a | 6 |
B-NH | 4.40 ± 1.99 a | 0.19 ± 0.06 a | 14 ± 5 a | 6.95 ± 2.56 a | 4415 ± 1023 ab | 0.12 ± 0.06 b | 1.66 ± 0.66 a | 1.29 ± 1.34 a | 8 | |
B-H | 4.88 ± 1.28 a | 0.22 ± 0.06 a | 13 ± 3 a | 6.59 ± 2.86 a | 5009 ± 1359 b | 0.11 ± 0.03 ab | 1.42 ± 0.75 a | 1.49 ± 1.19 a | 8 | |
Total | 5.06 ± 1.64 | 0.21 ± 0.06 | 14 ± 4 | 6.07 ± 2.59 | 4402 ± 1203 | 0.10 ± 0.05 | 1.45 ± 0.65 | 1.39 ± 1.05 | 22 | |
24 | UB | 4.52 ± 2.08 a | 0.21 ± 0.12 a | 13 ± 2 a | 9.47 ± 2.57 a | 14417 ± 15842 a | 0.43 ± 0.52 a | 1.24 ± 0.87 a | 0.42 ± 0.24 a | 6 |
B-NH | 3.57 ± 1.43 a | 0.16 ± 0.05 a | 13 ± 3 a | 8.86 ± 1.92 a | 20805 ± 20733 a | 0.88 ± 1.07 a | 1.36 ± 1.25 a | 0.21 ± 0.17 a | 8 | |
B-H | 4.00 ± 0.84 a | 0.21 ± 0.05 a | 11 ± 3 a | 9.13 ± 1.99 a | 30156 ± 28705 a | 0.83 ± 0.82 a | 1.24 ± 1.39 a | 0.22 ± 0.15 a | 8 | |
Total | 3.99 ± 1.45 | 0.19 ± 0.08 | 12 ± 3 | 9.12 ± 2.04 | 22463 ± 22799 | 0.74 ± 0.84 | 1.29 ± 1.16 | 0.27 ± 0.20 | 22 | |
Total | UB | 6.35 ± 2.21 b | 0.28 ± 0.13 a | 14 ± 4 b | 8.87 ± 6.93 a | 5746 ± 8088 a | 0.13 ± 0.26 a | 2.98 ± 3.84 a | 1.88 ± 1.83 b | 30 |
B-NH | 4.59 ± 1.62 a | 0.22 ± 0.08 a | 13 ± 3 a | 7.69 ± 3.93 a | 7199 ± 11231 b | 0.24 ± 0.56 b | 2.03 ± 1.44 a | 1.16 ± 1.29 a | 40 | |
B-H | 5.25 ± 1.90 a | 0.25 ± 0.09 a | 12 ± 2 a | 7.97 ± 5.95 a | 9466 ± 16148 b | 0.23 ± 0.46 b | 1.78 ± 1.20 a | 1.01 ± 0.88 a | 40 | |
Total | 5.31 ± 2.01 | 0.25 ± 0.1 | 13 ± 3 | 8.11 ± 5.58 | 7627 ± 12574 | 0.21 ± 0.46 | 2.20 ± 2.33 | 1.30 ± 1.38 | 110 |
Significant differences were observed between the burned and unburned areas (p = 0.023) in favor of unburned areas in regard to β-D glucosidase enzyme activity (Fig. 1d, Tab. 2). The qCO2 values did not vary significantly with burning (p = 0.786) except for Month 4 (Fig. 1e, Tab. 2).
There were significant differences between the different periods, in terms of the soil properties, with p < 0.05 for C/N and β-D glucosidase, and p < 0.001 for the others. The interaction effect of period and burning for qCO2 was significant (p < 0.05), while it was unclear for CO2 evolution (p = 0.052).
Cmic and Cmic/Corg were well correlated with each other, while negative relations between Corg, TN, qCO2 and β-D glucosidase were recorded (Tab. 3).
Tab. 3 - Correlation coefficients among the analyzed soil parameters (n=110). (Nt): total nitrogen; (Cmic): microbial carbon; (Corg): organic carbon; (β-DG): β-D glucosidase; (*): p<0.05; (**): p<0.001; (ns): non-significant.
- | Corg | Nt | C/N | CO2 efflux | Cmic | Cmic/Corg | qCO2 | β-DG |
---|---|---|---|---|---|---|---|---|
Corg | 1 | 0.873** | 0.207* | 0.128 ns | -0.277** | -0.384** | 0.135 ns | 0.181 ns |
Nt | - | 1 | -0.274** | 0.078 ns | -0.266** | -0.341** | 0.106 ns | 0.143 ns |
C/N | - | - | 1 | 0.055 ns | -0.001 ns | -0.076 ns | 0.000 ns | 0.097 ns |
CO2 efflux | - | - | - | 1 | 0.046 ns | 0.011 ns | 0.592** | -0.154 ns |
Cmic | - | - | - | - | 1 | 0.925** | -0.298** | -0.241* |
Cmic/Corg | - | - | - | - | - | 1 | -0.274** | -0.241* |
qCO2 | - | - | - | - | - | - | 1 | -0.024 ns |
β-DG | - | - | - | - | - | - | - | 1 |
Discussion
Although the Corg, C/N, Cmic, Cmic/Corg and β-D glucosidase values were different between the burned and unburned plots, there were no significant differences between the H (burned, harvested) and NH (burned, non-harvested) areas, in terms of the soil parameters.
Organic carbon content decreases as a result of fire as observed in this study, and changes may occur in organic matter fractions. As a matter of fact, loss of Corg and Nt has been reported due to the fire severity reaching 220-460 °C ([16], [12]). Similarly, the lower levels of OM were significant at Month 6 and 9 according to our results. Relatively high OM content in burned-harvested samples was likely due to mixing of the forest floor with the mineral soil during harvesting ([42]). Nt content in burned samples were low as well as non-significant in general. Higher Nt concentration was observed at Month 9 likely due to the warmer conditions in more moist unburned samples ([26], [27], [57]). Slight changes in C/N ratio may be related with the formation of new recalcitrant N and volatisation of C compounds, as suggested by previous studies conducted in burned pine forests ([43], [18]).
A general increase in Cmic was probably due to the increase in the concentration of oxidisable C and nutrients in burned soils as previously reported ([18], [17]); immediate increases have been found in soils after fire ([51], [14]). Likely because of the relatively late sampling in our study, we did not detect significant changes in the above variables in burned (especially burned and harvested) areas in Month 4 and Month 6. Bárcenas-Moreno et al. ([9]) categorised the process of microbial recovery after a wildfire as initial depletion of microorganisms in soil and the proliferation of fast-growing bacteria after a few months due to rapid and short increase of available nutrients. Cmic increase was reported after 8 months in a burned pine (Pinus halepensis) and oak (Quercus coccifera) mixed forest by Bárcenas-Moreno et al. ([9]) which was maintained for the rest of their study and reversed after 32 months. We assessed a significant decrease at Month 12 and non-significant decrease at Month 24 in Cmic values of the unburned areas, in comparison with those at Months 4 and 6. An increase in the total amount of Cmic was observed in all areas, more in the burned areas, likely due to the higher amount of rainfall between Months 12 and 24.
We found a negative correlation between Cmic and qCO2 that is inconsistent with the results from previous studies ([54], [8]), whereas a positive correlation between Cmic and CO2 evolution was detected. A high qCO2 indicates the need for restoring the C demand as a result of the microbial biomass depending on the renewal of C lost through respiration ([4], [8]). After a low-intensity prescribed fire in a Picea abies forest, basal respiration diminished while there was a disproportionate decrease in Cmic ([41]), which clearly led to higher values of qCO2 in the burned areas compared to unburned ones ([11]). This indicator predicts soil fertility ([3], [54], [6], [32], [7]). The lower levels of qCO2 in the burned soils in the first and second sampling dates indicate an improvement in soil conditions for the new vegetation due to the warmer soils in burned areas. Similar values in the burned and unburned areas after Month 9 may have resulted from vegetation succession ([23]).
β-D glucosidase enzyme activity plays an important role in energy availability in the soil, as it is directly related to the labile C content and its ability to stabilise the soil organic matter independently of seasonal variability ([34]). Our results indicate that this enzyme activity decreased with burning up to the last sampling date, two years after the wildfire, and was significantly different among H, NH, and UB plots at Month 9. The decrease in enzyme activity related with the lack of C entry to the system via plant inputs of Corg and Nt ([47], [48]) is consistent with our results. There was a negative correlation between enzyme activity and Cmic. The decrease in enzyme activity compared to Cmic likely resulted from the enzymes being retained in the stabilised fraction of the soil rather than that part associated with the viable microbial population ([31]).
Decomposition increased due to harvesting, possibly resulting in changes to the C budget ([39]). The harvesting of burned tree residues did not affect the measured soil parameters significantly in this study, probably because of the sensitive protection precautions used after the wildfire (such as covering the soil surface with residual brushwood material) and the rapid regeneration of forest ([19], [18]), which is characteristic of oak forests.
Conclusions
Fire affected the microbial biomass likely due to changes in the substrate quality, microbial composition, and abundance. However, this increase was not significant one year after burning. The correlation between qCO2 and β-D glucosidase activity showed that the former might be a more coherent indicator of microbial functionality than Cmic and CO2 evolution. In fact, enzymes might be independent of microbial proliferation, as it can attach to dead cells or cellular fragments instead of viable microorganisms.
The decrease in qCO2 after burning at low severity may indicate to the forest managers that the soil has relatively ideal conditions, simplifying the preparation of the area for new vegetation. The harvesting of burned trees in post-fire areas provides many advantages, in terms of economic benefits or protection of the forest against pests. Proper precautions for the soil, such as covering it after the fire with residues, comprising branches and small, felled burned trees, may be advantageous by allowing soil and nutrient loss to be avoided.
Acknowledgements
This study was supported by the General Directorate of Forestry of Turkey. All the analyses were conducted at the Research Institute for Forest Soil and Ecology in the context of project ESK-11 (6300)/2011-2015. Part of the data for some of the soil characteristics were used in the publication Kaptanoglu & Namli ([28]), for different periods and in a different context. We would like to thank Aydin Çömez, Oguz Can Turgay, Münevver Arslan, and Kürsad Özkan for their comments.
References
Gscholar
CrossRef | Gscholar
CrossRef | Gscholar
Gscholar
CrossRef | Gscholar
Gscholar
Gscholar
Gscholar
Online | Gscholar
CrossRef | Gscholar
Gscholar
CrossRef | Gscholar
Authors’ Info
Authors’ Affiliation
General Directorate of Forestry, Research Institute for Forest Soil and Ecology, 26160 Eskisehir (Turkey)
Corresponding author
Paper Info
Citation
Kaptanoglu AS, Namli A (2020). Wildfire and harvesting effects on carbon dynamics in an oak-pine mixed forest. iForest 13: 435-440. - doi: 10.3832/ifor3312-013
Academic Editor
Davide Ascoli
Paper history
Received: Dec 04, 2019
Accepted: Jul 08, 2020
First online: Sep 16, 2020
Publication Date: Oct 31, 2020
Publication Time: 2.33 months
Copyright Information
© SISEF - The Italian Society of Silviculture and Forest Ecology 2020
Open Access
This article is distributed under the terms of the Creative Commons Attribution-Non Commercial 4.0 International (https://creativecommons.org/licenses/by-nc/4.0/), which permits unrestricted use, distribution, and reproduction in any medium, provided you give appropriate credit to the original author(s) and the source, provide a link to the Creative Commons license, and indicate if changes were made.
Web Metrics
Breakdown by View Type
Article Usage
Total Article Views: 25838
(from publication date up to now)
Breakdown by View Type
HTML Page Views: 22974
Abstract Page Views: 1055
PDF Downloads: 1408
Citation/Reference Downloads: 2
XML Downloads: 399
Web Metrics
Days since publication: 1319
Overall contacts: 25838
Avg. contacts per week: 137.12
Article Citations
Article citations are based on data periodically collected from the Clarivate Web of Science web site
(last update: Feb 2023)
Total number of cites (since 2020): 1
Average cites per year: 0.25
Publication Metrics
by Dimensions ©
Articles citing this article
List of the papers citing this article based on CrossRef Cited-by.
Related Contents
iForest Similar Articles
Research Articles
Relationship between microbiological, physical, and chemical attributes of different soil types under Pinus taeda plantations in southern Brazil
vol. 17, pp. 29-35 (online: 28 February 2024)
Research Articles
Effects of altitudinal gradients on leaf area index, soil microbial biomass C and microbial activity in a temperate mixed forest ecosystem of Northwestern Turkey
vol. 10, pp. 334-340 (online: 15 December 2016)
Research Articles
Wood-soil interactions in soil bioengineering slope stabilization works
vol. 2, pp. 187-191 (online: 15 October 2009)
Research Articles
Soil fauna communities and microbial activities response to litter and soil properties under degraded and restored forests of Hyrcania
vol. 14, pp. 490-498 (online: 11 November 2021)
Short Communications
Is microbial biomass measurement by the chloroform fumigation extraction method biased by experimental addition of N and P?
vol. 14, pp. 408-412 (online: 04 September 2021)
Research Articles
Seasonal dynamics of soil respiration and nitrification in three subtropical plantations in southern China
vol. 9, pp. 813-821 (online: 29 May 2016)
Research Articles
Thinning effects on soil and microbial respiration in a coppice-originated Carpinus betulus L. stand in Turkey
vol. 9, pp. 783-790 (online: 29 May 2016)
Research Articles
Soil stoichiometry modulates effects of shrub encroachment on soil carbon concentration and stock in a subalpine grassland
vol. 13, pp. 65-72 (online: 07 February 2020)
Research Articles
Effects of arbuscular mycorrhizal fungi on microbial activity and nutrient release are sensitive to acid deposition during litter decomposition in a subtropical Cinnamomum camphora forest
vol. 16, pp. 314-324 (online: 13 November 2023)
Research Articles
Soil C:N stoichiometry controls carbon sink partitioning between above-ground tree biomass and soil organic matter in high fertility forests
vol. 8, pp. 195-206 (online: 26 August 2014)
iForest Database Search
Search By Author
Search By Keyword
Google Scholar Search
Citing Articles
Search By Author
Search By Keywords
PubMed Search
Search By Author
Search By Keyword