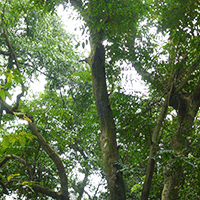
Seasonal dynamics of soil respiration and nitrification in three subtropical plantations in southern China
iForest - Biogeosciences and Forestry, Volume 9, Issue 5, Pages 813-821 (2016)
doi: https://doi.org/10.3832/ifor1828-009
Published: May 29, 2016 - Copyright © 2016 SISEF
Research Articles
Abstract
Numerous studies have documented that soil respiration and nitrogen cycling show a distinct seasonal dependence regulated by environmental factors (e.g., soil temperature and soil water content). The mechanisms controlling the seasonal dependence of these two key ecosystem processes have rarely been linked to both soil microbial community and soil environmental factors. Here, we present results on the seasonal patterns of soil respiration and gross nitrification rates in three subtropical plantations of Pinus massoniana, Castanopsis hystrix and Erythrophleum fordii over a period of 11 months. Turnover rates were measured with the Barometric Process Separation technique (BaPS). We elucidated how soil respiration and gross nitrification are controlled by the soil microbial community and by soil environmental factors. Soil respiration and gross nitrification showed strong seasonal dynamics, although no significant differences were observed among plantations. The turnover rates were the highest during the wet season and the lowest during the dry season. Microbial biomass, total phospholipid fatty acids (PLFAs), fungal PLFAs and bacterial PLFAs peaked during the dry season. Both soil respiration and gross nitrification rates were positively correlated with soil temperature and soil water content. Microbial biomass decreased with increasing turnover rates. Our findings highlight that carbon and nitrogen turnover rates were mostly controlled by soil temperature and soil water content.
Keywords
Soil Respiration, Nitrification, PLFA, Soil Microbial Community, N-fixing Tree Species, Subtropical China
Introduction
Forests are important carbon (C) pools in terrestrial ecosystems and play an important role in the global C and nitrogen (N) cycle. Soil CO2 efflux (soil respiration) is sensitive to climate, vegetation type and soil properties ([41]). Soil temperature and soil water content are recognized as the main factors controlling soil respiration ([24]), whereas the soil biophysical environment and substrate availability (e.g., aboveground and belowground litter fall, soil organic C) are the main factors controlling heterotrophic respiration ([45], [32]).
The N cycle in forests is a complex system with close linkages and interactions between soil, plants and microbes. The natural N supply for plants and microorganisms is derived from the mineralization of organic N compounds ([16]). This process occurs in two steps, ammonification and nitrification, which play a key role by making inorganic N compounds available for plants and microbes. The process is influenced by a number of factors such as composition and diversity of the soil microbial community, substrate quality and quantity, and environmental conditions (temperature and water content - [55], [18]). These factors are influenced by tree species and plantations. In fact, tree species are known to affect the physicochemical and biological characteristics of soils ([7], [49]). Soil microbial biomass (MB), activity, and community structure might thus be tree species dependent ([19]). Tree species also differ in the quality of leaf litter (e.g., C/N), which directly influences the quality and quantity of organic matter input ([55], [12]). Moreover, root litter is also species dependent and directly affects root exudation ([13]). Accordingly, microbes receive organic matter of varying quality from different tree species ([55]). This, in turn, may lead to changes in soil microbial communities and MB ([5]), which subsequently influence soil N transformations ([42], [53]). Most studies on microbial N cycling have concentrated on determining net nitrification rates ([38], [40], [62]) rather than gross rates. Direct comparisons of net and gross nitrification rates, however, reveal that the former can be one order of magnitude lower than the latter. Thus changes in the NO3- pool do not necessarily reflect the total turnover from NH4+ to NO3- ([52], [39], [22]). These findings highlight the importance of measuring gross nitrification rates to understand N transformation in forest ecosystems.
Only few studies have investigated gross nitrification in soil under different tree species/forest types. Brüggemann et al. ([12]) studied soil respiration, gross N mineralization and gross nitrification in pure stands of different temperate tree species. Matejek et al. ([34]) and Rosenkranz et al. ([44]) investigated ammonification and gross nitrification in forest soil layers in southern Germany (temperate climate). Comparable comprehensive studies are missing for subtropical climate regions.
Southern China, which is located mostly in the subtropical region, has 25 million hectares of plantations ([59]). Plantations are becoming a key component of the world’s forest resources and play an important role in the context of sustainable forest management ([60]). This calls for studies on soil C and N transformation under the main tree species used for afforestation to better understand the C and N cycle of subtropical plantation ecosystems.
Accordingly, the objective of this study was to determine the seasonal dynamics of soil respiration and nitrification in the top soil layer in subtropical plantations and to elucidate the relationships of these two key turnover processes with the composition of the soil microbial community (bacteria vs. fungi) and the two environmental factors soil temperature and soil water content.
Materials and methods
Study area
The study was conducted at the Experimental Center of Tropical Forestry, the Chinese Academy of Forestry (22° 06′ N, 106° 46′ E), Pingxiang City, Guangxi Zhuang Autonomous Region, China. Annual rainfall is about 1400 mm and occurs primarily from April to September. The annual mean temperature is 21 °C, the mean monthly minimum is 12.1 °C, and the mean monthly maximum is 26.3 °C. The soil was formed on granite, classified as red soil in Chinese soil classification, equivalent to Oxisol in USDA Soil Taxonomy ([27], [59], [21]).
We studied three of the most dominant plantations: one conifer plantation (Pinus massoniana Lamb.) and two broadleaved plantations (Castanopsis hystrix Miq. and Erythrophleum fordii Oliv.). E. fordii is a N-fixing species. These monoculture plantations were selected based on their similar topography, soil texture, stand age and management history. The three plantations were established after a clear-cut harvest of P. massoniana plantation in 1978, at an elevation of 350 m, over areas ranging from 2.2 to 4.8 hectares. Stem density varied from 410 to 415 trees per hectare, the diameter at breast height (DBH) ranged from 22.4 to 26.4 cm. In each plantation, five sampling squared plots (20 × 20m) were randomly selected and delineated.
Soil sampling
Soil was sampled monthly over a period of 11 months (from August 2011 to July 2012; except January, the date of sampling was the same every month, i.e., 15th), covering the entire season, i.e., wet, dry and intermediate conditions. Five intact soil cores were randomly taken from the top soil layer using a soil corer (5.6 cm diameter, 4.1 cm height) at each plot after removing the litter ([25], [60], [61]). The intact soil cores were analyzed for gross nitrification and soil respiration rates using the BaPS technique (UMS GmbH Inc., Germany - [22], [11], [26]).
Bulk soil samples for chemical analysis were collected from the top soil layer (0-5 cm) in late February 2012 (dry season) and July 2012 (wet season). A total of six soil cores per plot were randomly collected using a 5.0-cm-diameter stainless steel core and bulked to one composite sample. After collection, samples were immediately delivered to the laboratory for further analysis. In the lab, each composite sample was passed through a sieve (2 mm mesh size), and plant material was manually removed from the sieved soil. The sieved soil was divided into three subsamples. The first subsample was used to determine soil organic carbon (SOC), total nitrogen (TN) and soil pH. The second subsample was kept at 4 °C for analysis of nitrate (NO3--N), ammonium (NH4+-N), microbial biomass carbon (MBC) and microbial biomass nitrogen (MBN). The third subsample was used for total PLFAs, fungal and bacterial PLFAs determinations, and was stored at -20 °C.
Physicochemical analyses
Soil samples collected for physicochemical analyses were ground to pass through a 0.25 mm sieve. SOC was measured using the potassium dichromate vitriol oxidation method ([28]) and TN concentration was measured after semimicro-Kjeldahl digestion using a flow injection auto-analyzer (FIA®, Lachat Instruments, USA). Soil C/N values were calculated as the ratio of SOC to TN. Soil pH was determined using a 1:2.5 soil/water suspension. Inorganic N (ammonium and nitrate) was extracted with 2 M KCl solution. Ammonium and nitrate in extract were measured using the above flow injection auto-analyzer. Soil MBC and MBN were determined using the fumigation-extraction method ([58], [54]). Specifically, soil samples from each experimental site were divided into paired subsamples of 20 g. One subsample was immediately extracted with 60 ml 0.5 M K2SO4. The second subsample was fumigated with chloroform vapor for 48h in a desiccator followed by 10 vacuum/purge cycles, and then extracted as described above. Soil extractable organic C and TN in the K2SO4 extracts before and after the fumigation were quantified using a total C/N analyzer (Multi-N/C 2100®, Analytik Jena AG, Germany). The released C and N were converted to MBC and MBN, respectively, using the conversion factors Kec=0.45 and Ken=0.45. Soil temperatures at 5 cm depth (T5) were determined by a digital thermometer concomitantly with the soil samplings. All results were expressed per unit of oven-dried soil weight.
Phospholipid fatty acid extraction
The soil microbial community was characterized using PLFA analysis as described by Bossio & Scow ([10]). Lipids were extracted from 8 g of dry-weight-equivalent fresh soil with a one-phase mixture of chloroform, methanol and phosphate buffer (1:2:0.8). The separated fatty acid methyl-esters were re-dissolved in 200 µl hexane containing 19:0 as an internal standard and were analyzed using a Hewlett-Packard 6890 Gas Chromatograph equipped with an Ultra 2-methyl polysiloxane column. Concentrations of each PLFA were calculated based on the 19:0 internal standard concentrations. The abundance of individual fatty acids was determined as nmol per g of dry soil and standard nomenclature was used ([57]).
Bacteria were considered to be represented by 12 PLFAs (i14:0, i15:0, a15:0, 15:0, i16:0, 16:1ω7c, i17:0, a17:0, 17:0, cy17:0, cy19:0, 18:1ω7c), and gram-positive bacteria were identified by the PLFAs i14:0, i15:0, a15:0, i16:0, i17:0, a17:0, gram-negative bacteria by the PLFAs 16:1ω7c, cy17:0, cy19:0. The fungi were considered to be represented by the PLFAs 18:2ω6.9c and 18:1ω9c ([2], [20]). A ratio of the gram-positive bacteria to the gram-negative bacteria PLFAs (G+/G-) was used as an indicator of changes in the relative abundance of these two microbial groups; the ratio of 18:2ω6.9c and 18:1ω9c to total bacterial PLFAs was used to estimate the ratio of fungal to bacterial biomass (F/B) in soils ([4]). Several other PLFAs such as 16:0, 16:1 2OH, 16:1ω5c, 10Me16:0 and 10Me17:0 were detected and also used to analyze the composition of microbial community.
Determination of gross nitrification and soil respiration
The BaPS technique enables simultaneous determination of gross nitrification and soil respiration ([22], [23]). Previous studies testing the BaPS method against the 15N pool dilution technique revealed that using the default value of the respiration quotient (RQ) of unity tends to overestimate gross nitrification rates ([37], [43]). Müller et al. ([37]) thus suggested using the 15N pool dilution technique for determining the RQ value needed in the BaPS method. For acid forest soils, Rosenkranz et al. ([43]) and Matejek et al. ([35]) found average RQ values of 0.90 ± 0.01 and 0.89 ± 0.02, respectively. We therefore used a RQ of 0.9 in the present study. The autotrophic-to-heterotrophic nitrification ratio was kept at the default ratio of 3:1 ([22], [23]).
Gross nitrification and soil respiration rates were determined by incubating intact soil cores (5.6 cm diameter, 4.1 cm height), which were taken from the top soil from each sampling spot. Five replicates per experimental plantation were taken at each sampling date. Immediately after sampling, the undisturbed soil cores were sealed with parafilm and transported to the laboratory, where they were stored in the dark at in situ soil temperature as determined by use of Pt100 probes at the field site at 2 pm. Soil samples were incubated in the BaPS incubation chamber for 24h at stable in situ soil temperatures. At the end of the incubation, soil water content and bulk density were determined gravimetrically by drying soil samples at 105 °C for 24h. Water-filled pore space (WFPS
) was calculated using the following formula ([56] - eqn. 1):
where SWC
is the volumetric water content (cm3 cm-3), BD
is the soil bulk density (g cm-3), and PD
denotes the soil particle density, which was assumed to be 2.65 g cm-3 ([56], [11]).
Statistical analysis and data evaluation
Statistical analyses to determine significant differences between plantations and sampling dates were performed using SPSS® 16.0 and SigmaPlot® 10.0 (SPSS Inc., Chicago, USA). To test for normal distribution of data we used the normal probability plot. For the analyses of variance we used a one-way analysis of variance (ANOVA) with a least significant differences (LSD) post hoc test. Correlations between gross nitrification and soil respiration were also analyzed by bivariate linear regressions.
We used an exponential equation ([31]) to describe the response of the two turnover processes to temperature (eqn. 2):
where k
is the turnover rate of soil respiration or gross nitrification, T
is soil temperature measured at 5 cm depth, and a
and b
are regression coefficients. The temperature sensitivity (Q
10) was calculated as ([31] - eqn. 3):
The effect of WFPS
on the two turnover processes was evaluated with a simple linear regression ([30]) as follows (eqn. 4):
where c
and d
are the slope and the intercept, respectively.
We incubated the soil samples under in situ conditions, i.e., for incubation we used the temperature and the WFPS
measured at the field site. To disentangle the effect of differing temperature and WFPS
between the sites from other factors such as vegetation type or soil physicochemical properties, the rates were normalized for each month to the mean temperature and mean WFPS
used in the incubations (eqn. 5):
where k
is the measured rate, and k
* denotes the normalized rate, T
and {bar}T
are the site and mean temperature (N=3), respectively. The same notation was used for WFPS
.
The composition of the soil microbial community was summarized using principal component analysis (PCA) on the 19 individual PLFAs (nmol g-1 dry soil) from the PLFA analysis of soil samples after standardization for equal unit variance. To test for normal distribution of data we used the normal probability plot. Differences in individual soil PLFA among plantations and seasons were tested with one-way analysis of variances (ANOVA).
Results
Soil carbon and nitrogen pools
Soil properties including SOC and TN varied significantly among the plantations (Tab. 1). In particular, the TN content of the top soil in the plantation of the N-fixing species E. fordii was substantially higher than in the other two plantations. SOC in the E. fordii plantation was significantly higher than that in P. massoniana, although it was not significantly different from C. hystrix. There were no significant seasonal differences of the topsoil TN content among the plantations, while the SOC difference between dry and wet seasons was significant in the E. fordii and the C. hystrix plantations.
Tab. 1 - Soil pH and carbon and nitrogen pools of three subtropical plantations in Southern China. Data are means ± standard error (n=5). A one-way analysis of variance (ANOVA) with a least significant differences (LSD) post-hoc test was used. Different letters indicate significant differences (P<0.05) among plantations (lower case letters) or between different seasons (upper case letters).
Variable | Season | Erythrophleum fordii | Castanopsis hystrix | Pinus massoniana |
---|---|---|---|---|
pH | dry | 3.93 ± 0.04 Aa | 4.26 ± 0.01 Ab | 5.18 ± 0.04 Ac |
wet | 3.86 ± 0.03 Aa | 4.15 ± 0.05 Bb | 4.96 ± 0.04 Bc | |
SOC (g kg-1) | dry | 35.11 ± 3.93 Aa | 32.55 ± 0.36 Aab | 26.46 ± 1.29 Ab |
wet | 30.25 ± 1.86 Ba | 28.58 ± 1.08 Ba | 23.94 ± 1.07 Ab | |
TN (g kg-1) | dry | 2.86 ± 0.31 Aa | 2.11 ± 0.14 Ab | 1.59 ± 0.09 Ab |
wet | 2.50 ± 0.13 Aa | 2.03 ± 0.20 Ab | 1.60 ± 0.04 Ab | |
C:N ratio | dry | 12.28 ± 0.34 Aa | 15.63 ± 1.05 Ab | 16.69 ± 0.16 Ab |
wet | 12.07 ± 0.31 Aa | 14.33 ± 0.97 Ab | 14.88 ± 0.44 Bb | |
Nitrate (mg N kg-1) | dry | 9.73 ± 0.91 Aa | 2.07 ± 0.19 Ab | 3.07 ± 0.10 Ab |
wet | 7.54 ± 0.15 Ba | 3.30 ± 0.19 Bb | 2.91 ± 0.20 Ab | |
Ammonium (mg N kg-1) | dry | 18.26 ± 1.71 Aa | 17.26 ± 1.68 Aab | 13.38 ± 0.83 Ab |
wet | 18.83 ± 0.61 Aa | 16.28 ± 1.21 Aa | 15.74 ± 1.38 Aa |
Soil C to N ratios and nitrate concentrations varied significantly between the N-fixing vs. the non-N-fixing plantations (P < 0.05). Soil ammonium concentrations were the highest in the E. fordii plantation, where the soil pH values were the lowest.
Soil respiration rates
Soil respiration rates were variable over the two seasons in all the plantations. The minimum respiration rates occurred during the dry season, when soil water content decreased to values below 45.0% WFPS
. The maximum respiration rates were measured during the wet season when soil water content increased from 50.0% to 78.5% WFPS
(Fig. 1). Soil respiration rates were not significantly different among the plantations (P>0.05). In the dry season, the rates were 3-fold to 5-fold lower than in the wet season (Fig. 1).
Fig. 1 - Means (± SE) of soil temperature, WFPS, soil respiration and gross nitrification in the three plantations at different sampling dates over 2011-2012. A one-way analysis of variance (ANOVA) with a least significant differences (LSD) post-hoc test was used. Different letters indicate significant differences (P<0.05) among the plantations for a given sampling date. All rates were normalized to the mean temperature and WFPS of each month.
In all the three plantations, soil respiration increased with increasing temperature and WFPS
(Fig. 2). The response to temperature was well described by the exponential function (eqn. 2). The coefficient of determination ranged from 0.76 to 0.86 (Fig. 2a). Based on the regression coefficient b
of eqn. 2, Q
10 values of respiration were 2.87, 3.98 and 2.70 in the E. fordii, C. hystrix and P. massoniana plantations, respectively. Moreover, we found a linear relation between soil respiration and WFPS (R2 = 0.31-0.56, P < 0.0001 - Fig. 2b).
Fig. 2 - Relation between soil respiration rate and (a) soil temperature, or (b) water-filled pore space (WFPS) for the three plantations. Soil temperature was measured at 5 cm depth and soil water content was measured at 0-5 cm depth.
Gross nitrification rates
Gross nitrification rates showed a seasonal pattern similar to that of soil respiration (Fig. 1). The highest rates were recorded during the wet season and the lowest rates during the dry season. In the wet season, rates were up to 11-fold higher than in the dry season in the P. massoniana plantation (Fig. 1). Over the entire observation period, mean gross nitrification ranged between 0.11 and 2.06 mg N kg-1 SDW d-1. The differences among the plantations were not significant (Fig. 1).
The response of gross nitrification to temperature was well described with the exponential approach (R2 between 0.54 and 0.79, P < 0.0001 - Fig. 3a). The data, however, scattered much more strongly around the fit compared with the soil respiration data. The calculated Q
10 values were 3.39, 4.85 and 8.25 for E. fordii, C. hystrix and P. massoniana, respectively. Gross nitrification showed a weak linear relationship to WFPS (R2 = 0.14-0.32, P < 0.001 - Fig. 3b).
Fig. 3 - Relation between gross nitrification rate and (a) soil temperature, or (b) water-filled pore space (WFPS) for the three plantations. Soil temperature was measured at 5 cm depth and soil water content was measured at 0-5 cm depth.
Significant linear correlations between soil respiration and gross nitrification were found in all three plantations and the strongest correlation was in the E. fordii plantation (Fig. 4).
Fig. 4 - Linear correlation between gross nitrification rate and soil respiration rate for the three plantations.
Soil microbial community composition
Total PLFAs did not differ among the plantations, while the abundance of individual PLFAs did (Tab. 2). In the wet season, the abundance of gram-positive bacteria (i15:0, a15:0, i16:0, i17:0, a17:0), gram-negative bacteria (16:1ω7c, cy17:0, cy19:0) and ectomycorrhizal fungi (18:1ω9c) was higher in the C. hystrix plantation than in the E. fordii plantation (P < 0.05). Saprophytic fungi (18:2ω6.9c), arbuscular mycorrhizae fungi (16:1ω5c) and the ratio of Gram+ to Gram- differed significantly among the three plantations for two seasons; saprophytic fungi (18:2ω6.9c) and arbuscular mycorrhizae fungi (16:1ω5c) were higher in the dry season for all the plantations (Tab. 2).
Tab. 2 - Abundance of soil indicator lipids in the dry and wet season in the three plantations. Data are means ± standard error (n=5). A one-way analysis of variance (ANOVA) with a least significant differences (LSD) post-hoc test was used. Different letters indicate significant differences (P<0.05) among plantations (lower case letters) or between different seasons (upper case letters).
Group | Lipid abundance (nmol g-1) |
Season | Erythrophleum fordii | Castanopsis hystrix | Pinus massoniana |
---|---|---|---|---|---|
Overall | Total PLFA | dry | 18.46 ± 2.23 Aa | 20.89 ± 1.65 Aa | 16.19 ± 0.42 Aa |
wet | 10.65 ± 0.20 Ba | 14.41 ± 0.92 Bb | 9.73 ± 0.44 Ba | ||
Gram+ | i14:0 | dry | 0.09 ± 0.02 Aa | 0.15 ± 0.02 Ab | 0.12 ± 0.02 Aab |
wet | 0.10 ± 0.00 Aa | 0.13 ± 0.01 Aa | 0.12 ± 0.01 Aa | ||
i15:0 | dry | 2.16 ± 0.37 Aa | 3.32 ± 0.36 Ab | 2.27 ± 0.21 Aa | |
wet | 1.12 ± 0.06 Ba | 1.88 ± 0.19 Bb | 1.45 ± 0.12 Ba | ||
a15:0 | dry | 0.63 ± 0.10 Aa | 1.12 ± 0.12 Ab | 1.25 ± 0.14 Ab | |
wet | 0.39 ± 0.01 Ba | 0.75 ± 0.06 Bb | 0.81 ± 0.07 Bb | ||
i16:0 | dry | 1.89 ± 0.35 Aab | 1.92 ± 0.11 Aa | 1.16 ± 0.05 Ab | |
wet | 1.19 ± 0.06 Ba | 1.51 ± 0.14 Bb | 0.72 ± 0.06 Bc | ||
i17:0 | dry | 0.59 ± 0.07 Aa | 0.71 ± 0.06 Aa | 0.63 ± 0.05 Aa | |
wet | 0.44 ± 0.01 Ba | 0.52 ± 0.04 Ba | 0.43 ± 0.04 Ba | ||
a17:0 | dry | 0.35 ± 0.05 Aa | 0.53 ± 0.04 Ab | 0.49 ± 0.04 Ab | |
wet | 0.22 ± 0.00 Ba | 0.36 ± 0.03 Bb | 0.30 ± 0.03 Bb | ||
Gram- | 16:1w7c | dry | 0.44 ± 0.10 Aa | 0.79 ± 0.09 Ab | 0.72 ± 0.08 Aab |
wet | 0.22 ± 0.02 Ba | 0.42 ± 0.03 Bb | 0.38 ± 0.04 Bb | ||
cy17:0 | dry | 0.22 ± 0.05 Aa | 0.34 ± 0.03 Aac | 0.36 ± 0.05 Abc | |
wet | 0.10 ± 0.01 Ba | 0.21 ± 0.01 Bb | 0.18 ± 0.02 Bc | ||
cy19:0 | dry | 1.37 ± 0.22 Aa | 2.04 ± 0.21 Ab | 1.60 ± 0.13 Aab | |
wet | 0.82 ± 0.05 Ba | 1.48 ± 0.14 Ab | 1.30 ± 0.10 Ab | ||
Saprophytic fungi | 18:2w6.9c | dry | 0.50 ± 0.08 Aab | 0.75 ± 0.04 Aa | 0.48 ± 0.01 Ab |
wet | 0.30 ± 0.02 Ba | 0.42 ± 0.02 Bb | 0.20 ± 0.02 Bc | ||
Ectomycorrhizal fungi | 18:1ω9c | dry | 0.90 ± 0.18 Aa | 1.37 ± 0.14 Ab | 1.38 ± 0.09 Ab |
wet | 0.66 ± 0.05 Aa | 1.09 ± 0.08 Ab | 0.88 ± 0.08 Ba | ||
Arbuscular mycorrhizal fungi | 16:1 w5c | dry | 0.32 ± 0.07 Aa | 0.50 ± 0.07 Aab | 0.66 ± 0.10 Ab |
wet | 0.15 ± 0.01 Ba | 0.30 ± 0.03 Bb | 0.43 ± 0.05 Bc | ||
Gram + / Gram - | - | dry | 2.84 ± 0.07 Aa | 2.44 ± 0.09 Ab | 2.23 ± 0.05 Ab |
wet | 3.07 ± 0.08 Aa | 2.45 ± 0.11 Ab | 2.06 ± 0.02 Bc |
Principal Components Analysis of the microbial community composition, defined by the 19 PLFAs, showed that the first principal component (PC1) accounted for 65.8% and the second component (PC2) for 20.6% of the total variation in microbial communities (Fig. 5). The C. hystrix plantation was clearly separated from the other two on the PC1 axis (Fig. 5). This difference was mainly caused by the relatively higher abundances of Gram-positive bacteria, Gram-negative bacteria, saprophytic fungi and ectomycorrhizal fungi in the C. hystrix plantation. The E. fordii plantation was clearly separated from the P. massoniana plantation on the PC2 axis (Fig. 5) because the E. fordii plantation showed lower abundances of ectomycorrhizal fungi, arbuscular mycorrhizae fungi and Gram-negative bacteria (Tab. 2).
Fig. 5 - Principal component analysis of phospholipid fatty acid (PLFA) structures in the soils of the three plantations.
Microbial community and its relation to C and N turnover
Soil MB, total PLFAs, fungal PLFAs, bacterial PLFAs, soil respiration and gross nitrification rates were significantly different between the dry and wet season, while the latter two showed a seasonal pattern opposite to that of soil MB, total PLFAs, fungal PLFAs and bacterial PLFAs (Tab. 3). While soil respiration and gross nitrification rates were higher during the wet season, soil MB, total PLFAs, fungal PLFAs and bacterial PLFAs reached their lowest values during the same period.
Tab. 3 - Microbial, fungal and bacterial biomass along with soil respiration and gross nitrification rates of the top soil in the three plantations. Data are means ± standard error (n=5). A one-way analysis of variance (ANOVA) with a least significant differences (LSD) post-hoc test was used. Different letters indicate significant differences (P<0.05) among plantations (lower case letters) or between different seasons (upper case letters). Soil respiration and gross nitrification rates were normalized to the mean temperature and WFPS.
Variables | Season | Erythrophleum fordii | Castanopsis hystrix | Pinus massoniana |
---|---|---|---|---|
Microbial biomass C(mg kg-1) | dry | 412.01 ± 41.98 Aab | 516.01 ± 29.62 Aa | 368.99 ± 11.07 Ab |
wet | 205.44 ± 8.40 Ba | 464.61 ± 50.90 Ab | 197.36 ± 6.81 Ba | |
Microbial biomass N (mg kg-1) | dry | 106.66 ± 8.96 Aa | 89.57 ± 9.41 Aac | 69.40 ± 7.76 Abc |
wet | 58.10 ± 4.69 Ba | 55.82 ± 2.59 Ba | 53.67 ± 3.79 Aa | |
Microbial C:N ratio | dry | 3.87 ± 0.26 Aa | 6.11 ± 0.94 Aa | 5.71 ± 0.88 Aa |
wet | 3.60 ± 0.24 Aa | 8.48 ± 1.11 Ab | 3.79 ± 0.37 Aa | |
Total PLFAs (nmol g-1) | dry | 18.46 ± 2.23 Aa | 20.89 ± 1.64 Aa | 16.19 ± 0.42 Aa |
wet | 10.65 ± 0.20 Ba | 14.42 ± 0.92 Bb | 9.73 ± 0.44 Ba | |
Fungi (nmol g-1) | dry | 1.41 ± 0.25 Aa | 2.12 ± 0.18 Ab | 1.86 ± 0.09 Aab |
wet | 0.97 ± 0.07 Aa | 1.52 ± 0.09 Bb | 1.07 ± 0.10 Ba | |
Bacteria (nmol g-1) | dry | 12.16 ± 1.43 Aa | 14.67 ± 1.17 Aa | 11.84 ± 1.05 Aa |
wet | 6.48 ± 0.18 Ba | 9.59 ± 0.67 Bb | 6.29 ± 0.32 Ba | |
F:B ratio | dry | 0.12 ± 0.02 Aa | 0.15 ± 0.01 Aa | 0.16 ± 0.01 Aa |
wet | 0.15 ± 0.01 Aa | 0.16 ± 0.01 Aa | 0.17 ± 0.02 Aa | |
Soil respiration(mg C kg-1 sdw d-1) | dry | 4.80 ± 1.67 Aa | 6.76 ± 1.11 Aa | 6.21 ± 0.46 Aa |
wet | 25.26 ± 1.72 Ba | 20.96 ± 1.13 Ba | 22.04 ± 1.97 Ba | |
Gross nitrification(mg N kg-1 sdw d-1) | dry | 0.13 ± 0.05 Aa | 0.11 ± 0.02 Aa | 0.11 ± 0.03 Aa |
wet | 1.45 ± 0.10 Ba | 0.77 ± 0.11 Bb | 1.36 ± 0.19 Ba |
The highest MBC values were in C. hystrix, whereas the highest MBN values were in E. fordii for both dry and wet seasons. The P. massoniana plantation showed the lowest MBC and MBN contents (Tab. 3). Two PLFAs (18:2ω6.9c and 18:1ω9c) were used as indicators of fungal biomass. Both PLFAs varied among the plantations. The highest fungal PLFAs were recorded in the C. hystrix plantation, followed by P. massoniana and E. fordii. Moreover, the highest amount of bacterial PLFAs was in the C. hystrix plantation, and the lowest was in the P. massoniana plantation. PLFA-derived F/B ratios did not differ significantly among the plantations (Tab. 3).
Discussion
Soil respiration and gross nitrification in all the three plantations showed a pronounced seasonal pattern with significantly higher rates during the wet versus the dry season (Fig. 1 and Tab. 3). These findings are consistent with previous reports on the significant seasonal variation of soil respiration and gross nitrification rates in different geographic regions and different tree species ([12], [25], [64], [44], [29]).
In all the three plantations, soil respiration rate was significantly related to soil temperature and was well described by an exponential function within the temperature range observed in the field (Fig. 2a). This relation has been often described in the literature ([11], [44], [36]). Regression analysis showed that the observed variance of soil respiration was largely explained by soil temperature (R2=0.76-0.86; Fig. 2a). These values correspond very well to data of Luan et al. ([31]) in a warm-temperate forest ecosystem (R2 = 0.83-0.93). Our results also showed that the gross nitrification rate was positively correlated with soil temperature (Fig. 3a). Ingwersen et al. ([22]) found the highest gross nitrification at 25 °C, with a Q
10 value of 4.13 for the temperature range between 15 and 25 °C for coniferous forest in Germany. In the present study, the highest gross nitrification rate was recorded at 27.7 °C, and a Q
10 value of 5.49 was calculated within the temperature range from 16 to 26 °C. This is somewhat higher than the value obtained by Breuer et al. ([11]) for a rainforest ecosystem (Q
10 = 3.60, temperature range: 14-24 °C) and the values derived from the data set by Ingwersen et al. ([22]) for a temperate forest ecosystem. In this context, Q
10 values derived under field conditions merely indicate the “apparent” temperature sensitivity ([50]), because other factors such as WFPS
or N availability are not constant in time and may superimpose with the temperature response. This may explain the quite high Q
10 values, which are usually not found in lab incubation experiments.
Soil moisture conditions can markedly impact the microbial processes and ecological interactions involved in nutrient cycling, such as soil C and N turnover rates ([6], [8], [15], [29]). In this study, both soil respiration and gross nitrification rates were positively correlated with soil WFPS
(Fig. 2b and Fig. 3b). Some authors reported that soil water content has significant positive effects on soil respiration rates ([36], [44]). In addition, Bengtson et al. ([6]) and Chen et al. ([15]) found that both respiration and gross nitrification rates in forest ecosystems increase with increasing moisture under field conditions. Our study demonstrates that those results are applicable under field conditions in subtropical plantations.
Soil C and N turnover are controlled by microbial processes. In general, a warm and humid season is expected to be more favorable for mineralization, whereas the dry season is typically more favorable for immobilization. We found lowest MBC, MBN, total PLFAs, fungal PLFAs and bacterial PLFAs during the wet season, when temperature and soil moisture conditions were favorable for the microbial community (Tab. 3). During the period of the lowest MB, however, we observed the highest soil respiration and gross nitrification rates (Tab. 3). This finding agrees very well with the results of Maithani et al. ([33]) and Arunachalam et al. ([1]). They found that, in regrowth of a disturbed subtropical humid forest in north-east India, the periods of high mineralization coincided with minimum MB, whereas periods of immobilization corresponded with times of highest MB. In the subtropical forest of Meghalaya, India, Das et al. ([16]) also reported highest N-mineralization rates in the rainy season, while MBN was low in the rainy season and high in the dry winter season. As discussed by Maithani et al. ([33]) and Barbhuiya et al. ([3]), lower MB values during the rainy season, when temperature and soil moisture conditions were favorable for the microbes, indicated a period of rapid mineralization in soil. Sarathchandra et al. ([46]) and Singh et al. ([48]) reported that the relatively greater nutrient demand by plants during the wet season (the peak vegetative growth period) limited the availability of nutrients to soil microbes and thereby reduced their immobilization in MB. Moreover, when soil dries out during the dry season, substrate supply might become limiting. Then, microbes may experience resource limitation that can slow down biogeochemical processes and force microbes into a dormant state ([51], [47]). During the dry season, low water content can inhibit microbial activity by lowering intracellular water potential. This causes microbes to acclimate to decreasing water potentials by altering their allocation of resources ([47]). We therefore expect that microorganisms use most of the available resources to synthesize biomass during the transition between wet and dry season. Chen et al. ([14]) also found that a high level of MBC and MBN in late winter in a hoop pine plantation coincided with low temperatures and low microbial activity.
Soil MB and microbial community composition have been shown to affect soil C and N cycling ([9], [63], [29]). Soil in the E. fordii plantation had higher concentrations of SOC, TN, inorganic N and MBN than soil in the two other plantations (Tab. 1 and Tab. 3). Moreover, the highest C and N turnover rates coincided with the lowest fungal biomass and highest N availability (Tab. 1 and Tab. 3). This agrees with other studies that found fungal biomass to be negatively correlated with soil fertility and N availability ([17], [20], [9]). This may be caused by microbial communities affecting C and N processes or, more likely, by N availability affecting the microbial communities ([17]).
Conclusions
The present study revealed no significant differences in soil respiration and gross nitrification among the three plantations, but seasonal variation in the C and N processes was highly significant. The seasonal variation of soil respiration and gross nitrification was mostly controlled by environmental factors such as soil temperature and soil water content. The role of the microbial community in this context was less clear, while respiration and nitrification were related to MBN and fungal biomass. Moreover, MB and total PLFA content were negatively correlated with C and N turnover, showing that these two measures alone are poor indicators for microbial activity in soils that experience environmental stress such as drought.
Abbreviations
The following abbreviations were used throughout the paper:
- BaPS: barometric process separation;
- PLFA: phospholipid fatty acid;
- MB: microbial biomass;
- SOC: soil organic carbon;
- TN: total nitrogen;
- MBC: microbial biomass carbon;
- MBN: microbial biomass nitrogen;
- T5: soil temperature at 5 cm soil depth;
- F/B: ratio of fungal to bacterial biomass;
- RQ: respiration quotient;
- WFPS: water-filled pore space;
- Q10: temperature sensitivity;
- SDW: soil dry weight.
Acknowledgements
We are grateful to Lihua Lu, Haolong Yu and Angang Ming for their help with field sampling. This study was funded by the Project in the National Science & Technology Pillar Program during the Twelfth Five-year Plan Period (No. 2012BAD22B0102), National Scientific Foundation of China (31290223) and the Project of the Special Program on Carbon of the Chinese Academy of Sciences (No. XDA05060100).
References
CrossRef | Gscholar
Gscholar
Gscholar
CrossRef | Gscholar
CrossRef | Gscholar
Authors’ Info
Authors’ Affiliation
College of Forestry and Horticulture, Xinjiang Agricultural University, Urumqi 830052 (China)
Ruimei Cheng
Zuomin Shi
Da Luo
Shirong Liu
Key Laboratory on Forest Ecology and Environmental Sciences of State Forestry Administration; Institute of Forest Ecology, Environment and Protection, Chinese Academy of Forestry, Beijing 100091 (China)
Zuomin Shi
Co-Innovation Center for Sustainable Forestry in Southern China, Nanjing Forestry University, Nanjing 210037 (China)
Institute of Soil Science and Land Evaluation, University of Hohenheim, Stuttgart D-70593 (Germany)
Corresponding author
Paper Info
Citation
Wang W, Cheng R, Shi Z, Ingwersen J, Luo D, Liu S (2016). Seasonal dynamics of soil respiration and nitrification in three subtropical plantations in southern China. iForest 9: 813-821. - doi: 10.3832/ifor1828-009
Academic Editor
Elena Paoletti
Paper history
Received: Aug 28, 2015
Accepted: Feb 02, 2016
First online: May 29, 2016
Publication Date: Oct 13, 2016
Publication Time: 3.90 months
Copyright Information
© SISEF - The Italian Society of Silviculture and Forest Ecology 2016
Open Access
This article is distributed under the terms of the Creative Commons Attribution-Non Commercial 4.0 International (https://creativecommons.org/licenses/by-nc/4.0/), which permits unrestricted use, distribution, and reproduction in any medium, provided you give appropriate credit to the original author(s) and the source, provide a link to the Creative Commons license, and indicate if changes were made.
Web Metrics
Breakdown by View Type
Article Usage
Total Article Views: 48607
(from publication date up to now)
Breakdown by View Type
HTML Page Views: 41109
Abstract Page Views: 2667
PDF Downloads: 3616
Citation/Reference Downloads: 60
XML Downloads: 1155
Web Metrics
Days since publication: 3098
Overall contacts: 48607
Avg. contacts per week: 109.83
Article Citations
Article citations are based on data periodically collected from the Clarivate Web of Science web site
(last update: Feb 2023)
Total number of cites (since 2016): 7
Average cites per year: 0.88
Publication Metrics
by Dimensions ©
Articles citing this article
List of the papers citing this article based on CrossRef Cited-by.
Related Contents
iForest Similar Articles
Research Articles
The manipulation of aboveground litter input affects soil CO2 efflux in a subtropical liquidambar forest in China
vol. 12, pp. 181-186 (online: 10 April 2019)
Research Articles
Soil respiration along an altitudinal gradient in a subalpine secondary forest in China
vol. 8, pp. 526-532 (online: 01 December 2014)
Research Articles
Soil respiration and carbon balance in a Moso bamboo (Phyllostachys heterocycla (Carr.) Mitford cv. Pubescens) forest in subtropical China
vol. 8, pp. 606-614 (online: 02 February 2015)
Research Articles
Wood-soil interactions in soil bioengineering slope stabilization works
vol. 2, pp. 187-191 (online: 15 October 2009)
Research Articles
Thinning effects on soil and microbial respiration in a coppice-originated Carpinus betulus L. stand in Turkey
vol. 9, pp. 783-790 (online: 29 May 2016)
Research Articles
Spatial heterogeneity of soil respiration in a seasonal rainforest with complex terrain
vol. 6, pp. 65-72 (online: 07 February 2013)
Research Articles
Effect of different dolomitic limestone dosages on soil respiration in a mid-altitudinal Norway spruce stand
vol. 12, pp. 357-365 (online: 05 July 2019)
Research Articles
Response of soil bacterial communities to nitrogen and phosphorus additions in an age-sequence of subtropical forests
vol. 14, pp. 71-79 (online: 11 February 2021)
Research Articles
Relationship between microbiological, physical, and chemical attributes of different soil types under Pinus taeda plantations in southern Brazil
vol. 17, pp. 29-35 (online: 28 February 2024)
Research Articles
Short-time effect of harvesting methods on soil respiration dynamics in a beech forest in southern Mediterranean Italy
vol. 10, pp. 645-651 (online: 20 June 2017)
iForest Database Search
Search By Author
Search By Keyword
Google Scholar Search
Citing Articles
Search By Author
Search By Keywords
PubMed Search
Search By Author
Search By Keyword