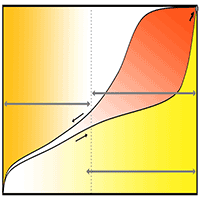
Moisture in modified wood and its relevance for fungal decay
iForest - Biogeosciences and Forestry, Volume 11, Issue 3, Pages 418-422 (2018)
doi: https://doi.org/10.3832/ifor2406-011
Published: Jun 05, 2018 - Copyright © 2018 SISEF
Review Papers
Collection/Special Issue: COST action FP1407
Understanding wood modification through an integrated scientific and environmental impact approach
Guest Editors: Giacomo Goli, Andreja Kutnar, Dennis Jones, Dick Sandberg
Abstract
Water plays an essential role in fungal decay of wood, and limiting the cell wall moisture content by chemical modification can effectively improve the durability of the material. Investigating the wood-water relations of modified material under climatic conditions relevant for fungal decay are, however, experimentally challenging. Most studies in literature therefore focus on moisture sorption under conditions outside those of importance for fungal decay. This review discusses the validity of such data for characterising the wood-water relations at very humid climatic conditions, relevant for fungal decay. Moreover, the review attempts to cover the basics of fungal decay, the important role of water, and how controlling water content by modification can improve durability.
Keywords
Introduction
Some of the most widespread, economically important, and devastating wood-decaying organisms are basidiomycetes fungi ([74], [22], [1], [65]). A lot of research is therefore dedicated to understand their degradation mechanisms and the basic conditions necessary for decay ([2], [70], [71], [61], [62], [63], [87], [47], [55]), in order to prevent fungal attack of wood. Protection of wood structures has traditionally been accomplished by using toxic preservative systems, i.e., fungicides, but environmental concerns and restrictions of their use have increased the focus on non-toxic alternatives such as chemical modification ([36]). A large number of physicochemical modification processes exist aimed at improving various aspects of the performance of wood materials. In this review, the focus is on modifications targeting an improved durability, and how their performance is linked to wood-water relations of the modified material. Recent years have seen several new findings regarding the protection mechanism, and this reviews attempts to cover both the basics of fungal decay, the important role of water, and how controlling water content by modification can improve durability.
Fungal decay mechanisms and the importance of water
Fungi employ enzymes for the conversion of cell wall polymers into smaller fragments which can be consumed. However, as cell wall pores, even in the water-saturated state, are too small for enzymes to enter ([67], [19], [20], [21]), fungi break up cell walls by oxidative action ([18]). While fungi classified as white-rot fungi rely on enzymes for this task ([73], [40], [60]), another class of fungi termed brown-rot fungi have evolved a non-enzymatic strategy based on Fenton chemistry to disrupt cell walls in the initial stage of attack ([30], [81], [34], [8], [35], [64], [61], [89]). It is speculated that through transportation of chelated iron ions into wood cell walls and reaction of these with hydrogen peroxide, brown-rot fungi create highly reactive free radicals which disrupt chemical bonds of the cell wall constituents. This mechanism can work at a distance of several microns from the fungi to create pathways within cell walls, through which the lignocellulolytic enzymes can penetrate ([32], [31], [80], [8], [9], [61], [43]). That such a combination of non-enzymatic and enzymatic degradation machinery is effective is apparent from the high relative occurrence (73-85 % of cases) of brown-rot in decaying wooden structures compared with white-rot decay ([22], [74], [1], [65]).
A prerequisite for fungal decay is sufficient ambient temperature and moisture conditions of the wood. Being a hygroscopic material, wood can absorb/desorb and exchange water molecules with the surrounding air. Water in wood, often termed moisture, can be found both within cell walls as bound water and outside cell walls in the wood void structure (pits, lumens, vessels, etc.) as capillary water or vapour ([24]). The moisture distribution between cell walls and voids depends on the climatic conditions. Thus, for relative humidity (RH) levels up to about 97-98 %, the moisture content in equilibrium with the ambient RH is dominated by bound water ([23]). However, as the RH approaches saturation (100% RH) the contribution from capillary water held in the void structure becomes significant and eventually dominates the moisture content at very high (> 99 %) RH ([68], [33], [27], [17], [3], [4], [29], [28]). In line with common practice within building materials research, the RH-range from 0% to 97-98 % is in this review referred to as “hygroscopic” and the range above as “over-hygroscopic” (see Fig. 1).
Fig. 1 - Schematic illustration of sorption isotherms for wood in absorption from absolute dry state (lower black line) and desorption from fully water-saturated state (upper black line) in the entire relative humidity (RH) range.
It is often assumed that liquid water, e.g., capillary water in the wood void structure, should be available for fungal decay to be possible ([46], [65]). While it is evident that fungal decay is only relevant at high RH (Fig. 1) and that in general wood moisture contents of 40-70 % are most favourable for decay ([74]), the essential presence of capillary water is questionable. For instance, the lowest RH capable of supporting brown-rot decay is around 92-97 % RH ([33], [15], [75], [65]), the exact threshold depending on temperature and fungal species ([75]). Wood in equilibrium with this level of RH only contains minuscule amounts of capillary water that is found in pores smaller than 70 nm. For Norway spruce, this amount of water outside cell walls is of the order of 10 µg water per gram wood ([23]). The question of whether capillary water is essential for fungal decay is, however, obscured by experimental limitations. It is difficult to maintain a stable climate above 97 % RH ([33]), and studies of moisture in wood in the over-hygroscopic regime therefore requires special techniques, e.g., pressure plate. For this reason, studies on the effect of initial wood moisture conditions on fungal decay often employ wood specimens exposed to liquid water and perhaps partially dried to specific wood moisture contents ([58]). This inevitably causes moisture gradients in the specimens, but further complications arise with controlling the moisture conditions during decay tests ([6]). During such tests both the hygroscopicity of the material changes ([13], [5], [66], [79], [7]), and the amount of water increases due to fungal respiration ([53], [39], [51], [76], [71]). These issues of lack of climate control and changes in the substrate during the tests seriously complicate the interpretation of the limiting moisture conditions for decay.
Improving durability by chemical modification
Since sufficient moisture conditions are central to fungal decay, it has long been recognised that durability can be obtained by keeping wood dry ([52], [46], [16]). However, for some applications keeping wood dry is impossible, e.g., under direct exposure to rain or in very humid environments. In these cases, alternative strategies are needed to avoid fungal decay. While traditional wood protection depends on toxic preservatives, chemical modification improves the durability the modified material by non-toxic means. The exact mechanisms behind the increased resistance to fungal decay observed for several kinds of chemical modification is not fully clear, but it is undoubtedly linked with reductions in the moisture content of cell walls ([70]). Thus, even at high moisture contents where fungal decay of untreated wood is possible, modified wood can be decay resistant ([14], [26], [25], [78], [37], [71]). This illustrates that the total amount of water available to fungi is not a predictor of the potential for wood decay as sufficient cell wall moisture content needs to be present as already speculated a century ago ([88]).
As described previously, investigating the wood-water relations in the over-hygroscopic regime, relevant for fungal decay, is challenging. Therefore, the vast majority of studies of wood-water relations for modified wood focus on the hygroscopic range, while the over-hygroscopic range is only covered in two studies on modified wood ([72], [82]) and few more for unmodified wood ([68], [33], [27], [17], [3], [4], [29], [28], [86]). This raises the important question of whether observations about wood-water relations in the hygroscopic range are valid for the over-hygroscopic range as well. For instance, Thybring ([70]) found a common moisture threshold for decay in several different types of modified wood, where a reduction of about 40 % in moisture content at similar hygroscopic RH conditions (in the range 30-90 %) was found to correlate with resistance to fungal decay across widely different modifications. One exception is thermally modified wood which is not fully decay resistant ([45], [77], [50]), despite a 40 % reduction in moisture content under hygroscopic conditions. This illustrates the need for determining wood-water relations at moisture conditions relevant for fungal decay (Fig. 1).
Potential mechanisms for improved durability of modified wood towards brown-rot decay
It is apparent that the durability of wood can be improved by reducing the cell wall moisture content through modification, but the actual mechanism of protection has not yet been resolved. In a recent review, Zelinka et al. ([87]) discuss the potential mechanisms behind decay resistance from modification based on the observation by Zelinka et al. ([85]) that ions within cell walls have a threshold moisture content below which they cannot diffuse. Ion transport in wood has been linked with the formation of a continuous network of cell wall water (a percolation threshold - [84], [83], [44]), and limiting the cell wall moisture might prevent the formation of such a network, hereby disrupting the physical pathways for transport of solutes. As the initial stage of brown-rot attack involves the transport of ions into cell walls ([47]), it seems reasonable that the formation of a continuous water-swollen porosity of sufficient pore size is a prerequisite for this transport. Hosseinpourpia & Mai ([41], [42], [43]) have conducted a series of experiments where modified wood veneers are exposed sequentially to solutions of iron ions and hydrogen peroxide, mimicking the oxidative Fenton chemistry of brown-rot fungi. Their results show that for acetylated and phenol-formaldehyde modified wood, hydrogen peroxide is not consumed in a solution with iron ions and modified wood after 48 hours of exposure given that the modification intensity (WPG) is high enough ([41], [42]). Moreover, controls of modified wood of adequately high WPG exposed only to the iron ion solution did not take up iron during the 48 hour experiment. The threshold WPG in both cases was consistent with the 17-20 % WPG reported for acetylated exposed in laboratory and field tests ([59], [48], [69], [10], [12], [56], [54], [37], [57], [11], [38]). For thermally modified wood, the uptake of iron ions and consumption of hydrogen peroxide was markedly reduced during the 48 hour exposure time for wood of high modification intensity ([43]), but no threshold was found. This indicates that solute transport is slowed but not hindered in thermally modified wood, since fungal agents presumably can be transported in the micro-porosity created as cell wall material is lost during modification ([49], [50]). If the pore size is sufficiently large, enzymes may even be transported into the cell walls. This is seen for pretreated wood material where the water-swollen volume in cell walls accessible to 5.1 nm probe molecules correlates linearly with hydrolysis yield ([32], [31], [80]). Perhaps the durability of thermally modified wood could be optimised further if the created micro-porosity could be tuned. This would require detailed investigations of the cell wall micro-porosity in thermally modified wood, and how processing conditions potentially affect it.
Conclusion
Water plays an essential role in fungal decay of wood, and limiting the cell wall moisture content by chemical modification can effectively improve the durability of the material. Investigations of the wood-water relations under climatic conditions relevant for fungal decay are, however, difficult, and thus many studies focus on the relative humidity (RH) range below 95 %. While the cell wall moisture content in the over-hygroscopic range (> 98 % RH) is underestimated by extrapolation of data obtained below 95 % RH, the relative reductions in cell wall moisture content appear similar for acetylated wood. This might not be the case for other types of modification.
Reductions in cell wall moisture content are thought to prevent fungal decay by hindering transport of fungal agents into the cell walls, presumably from a disruption of the continuous water network within cell walls otherwise found in untreated wood at high moisture contents.
Acknowledgements
EET gratefully acknowledges financial support from the VILLUM FONDEN Postdoc programme. KM and LR gratefully acknowledges financial support from European Regional Development Fund through South Savo Regional Council from Finland and industrial partners. The authors acknowledge COST Action FP1407 “Understanding wood modification through an integrated scientific and environmental impact approach (ModWoodLife)”.
References
Gscholar
Gscholar
Online | Gscholar
Gscholar
CrossRef | Gscholar
Gscholar
Gscholar
CrossRef | Gscholar
Gscholar
Gscholar
Gscholar
Gscholar
Gscholar
Gscholar
Gscholar
CrossRef | Gscholar
Gscholar
Gscholar
Gscholar
Gscholar
Gscholar
CrossRef | Gscholar
Gscholar
CrossRef | Gscholar
Gscholar
Gscholar
Gscholar
Gscholar
Gscholar
Authors’ Info
Authors’ Affiliation
University of Copenhagen, Department of Geosciences and Natural Resource Management, Rolighedsvej 23, DK-1958 Frederiksberg C (Denmark)
Lauri Rautkari
Aalto University, Department of Bioproducts and Biosystems, PO BOX 6300, F-00076 Aalto (Finland)
Corresponding author
Paper Info
Citation
Thybring EE, Kymäläinen M, Rautkari L (2018). Moisture in modified wood and its relevance for fungal decay. iForest 11: 418-422. - doi: 10.3832/ifor2406-011
Academic Editor
Giacomo Goli
Paper history
Received: Feb 13, 2017
Accepted: Mar 18, 2018
First online: Jun 05, 2018
Publication Date: Jun 30, 2018
Publication Time: 2.63 months
Copyright Information
© SISEF - The Italian Society of Silviculture and Forest Ecology 2018
Open Access
This article is distributed under the terms of the Creative Commons Attribution-Non Commercial 4.0 International (https://creativecommons.org/licenses/by-nc/4.0/), which permits unrestricted use, distribution, and reproduction in any medium, provided you give appropriate credit to the original author(s) and the source, provide a link to the Creative Commons license, and indicate if changes were made.
Web Metrics
Breakdown by View Type
Article Usage
Total Article Views: 44777
(from publication date up to now)
Breakdown by View Type
HTML Page Views: 36212
Abstract Page Views: 2993
PDF Downloads: 4799
Citation/Reference Downloads: 12
XML Downloads: 761
Web Metrics
Days since publication: 2361
Overall contacts: 44777
Avg. contacts per week: 132.76
Article Citations
Article citations are based on data periodically collected from the Clarivate Web of Science web site
(last update: Feb 2023)
Total number of cites (since 2018): 23
Average cites per year: 3.83
Publication Metrics
by Dimensions ©
Articles citing this article
List of the papers citing this article based on CrossRef Cited-by.
Related Contents
iForest Similar Articles
Research Articles
Examining the evolution and convergence of wood modification and environmental impact assessment in research
vol. 10, pp. 879-885 (online: 06 November 2017)
Research Articles
Kinetic analysis of poplar wood properties by thermal modification in conventional oven
vol. 11, pp. 131-139 (online: 07 February 2018)
Research Articles
Characterization of VOC emission profile of different wood species during moisture cycles
vol. 10, pp. 576-584 (online: 08 May 2017)
Research Articles
Changes in moisture exclusion efficiency and crystallinity of thermally modified wood with aging
vol. 12, pp. 92-97 (online: 24 January 2019)
Research Articles
Improving dimensional stability of Populus cathayana wood by suberin monomers with heat treatment
vol. 14, pp. 313-319 (online: 01 July 2021)
Research Articles
Thermo-modified native black poplar (Populus nigra L.) wood as an insulation material
vol. 14, pp. 268-273 (online: 29 May 2021)
Review Papers
Wood modification technologies - a review
vol. 10, pp. 895-908 (online: 01 December 2017)
Research Articles
NIR-based models for estimating selected physical and chemical wood properties from fast-growing plantations
vol. 15, pp. 372-380 (online: 05 October 2022)
Research Articles
Identification of wood from the Amazon by characteristics of Haralick and Neural Network: image segmentation and polishing of the surface
vol. 15, pp. 234-239 (online: 14 July 2022)
Research Articles
Study on the chemical composition of teak wood extracts in different organic solvents
vol. 14, pp. 329-336 (online: 09 July 2021)
iForest Database Search
Search By Author
Search By Keyword
Google Scholar Search
Citing Articles
Search By Author
Search By Keywords
PubMed Search
Search By Author
Search By Keyword