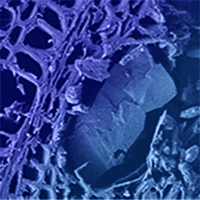
Improving dimensional stability of Populus cathayana wood by suberin monomers with heat treatment
iForest - Biogeosciences and Forestry, Volume 14, Issue 4, Pages 313-319 (2021)
doi: https://doi.org/10.3832/ifor3684-014
Published: Jul 01, 2021 - Copyright © 2021 SISEF
Research Articles
Abstract
This paper presents a wood modification method using renewable and non-toxic suberin monomers (SMs) under heat treatment to improve dimensional stability of wood from fast-growing species. Specimens of poplar (Populus cathayana) wood were impregnated with SMs and then subjected to heat treatment at 180°C for two hours. The untreated wood (Control), suberin monomers impregnated wood (Sub) and suberin monomers impregnated wood with heat treatment (Sub 180°C) were analyzed by scanning electron microscopy (SEM), confocal laser scanning microscopy (CLSM), and Fourier transform infrared spectroscopy (FTIR). The hygroscopicity and dimensional stability of modified wood were evaluated. The results showed that SMs in the treated wood were located in the cell lumen of fibers and vessels, as well as in the cell wall which was bulked. The dimensional stability of SMs modified wood was improved, and this enhancement became more pronounced by a combination with heat treatment. The anti-swelling efficiency of Sub and Sub 180°C treatments were 30.0% and 49.6%, respectively. The presented results showed potential of SMs treatment to develop an effective modification approach and improve dimensional stability of wood of fast-growing species, as well as to promote the reuse of suberin from the bark.
Keywords
Poplar, Wood Modification, Suberin, Dimensional Stability, Heat Treatment
Introduction
As a biocomposite, the unique characteristics of wood, such as esthetic grain, great workability, high ratio of strength-to-weight, thermal insulation as well as environment-conditioning ability, make it a natural material for decoration, furniture, and construction. However, wood is a natural hygroscopic material. The main chemical components of wood are cellulose, hemicelluloses, and lignin, which have a large number of hydroxyl groups leading to dimensional instability, especially from polysaccharides ([22]). The dimensional changes of wood are different in the main anatomical directions due to its anisotropy. Therefore, warping, cracking, and other defects occur in practice frequently. The dimensional instability of wood will adversely affect its performances, especially when wood is used in furniture ([6]). Wooden material can be modified by various reagents, which improved its performances in service ([43], [37], [36]). Polyethylene glycol (PEG) is a wood modifier commonly used to improve the dimensional stability of wood by bulking the cell wall. However, its raw materials come from petroleum, failing to cater the requirements of sustainable development. Further, toxic reagents, such as phenolic resin, urea-formaldehyde resins, are not desirable in practical application, although they provide excellent modification effects. Health is the basic requirement of family life, and the release of formaldehyde from wooden materials is an endangering factor for people’s health ([42]). The International Agency for Research on Cancer announced in 2004 that formaldehyde is a recognized human carcinogen, which can cause human nasopharyngeal carcinoma ([7]). Formaldehyde can irritate eyes, mucous membranes and skin at certain concentrations ([11]), and the maximum allowable air concentration of formaldehyde indoor is 0.08 mg m-3 in China ([15]).
Today, many chemicals from forest and agriculture feedstocks are potentially available in large amounts ([13]). Among them, suberin is a natural aromatic-aliphatic crosslinked polyester, playing the role of protective barrier between plants and environment ([3]). Suberin prevents water loss during transport and protects plants from invasion by pathogens ([12]). Suberin is ubiquitous throughout the plant, particularly abundant in the cork oak (Quercus suber L.), where almost half of the total bark weight is made up of suberin ([33], [32]). In 2017, the cork production was 201.000 tons worldwide, and Portugal accounted for 55% ([1]). It has been reported that 40% of cork productions were wine stoppers, and 66% of used wine stoppers went to landfill ([28]). On the other hand, the cork stopper industry in Portugal and the birch pulp mills in Nordic countries generate considerable amounts of suberin-rich residues, around 40.000 tons per year ([40]). Specifically, a single pulp mill processing could produce approximate 28.000 tons per year of suberin-rich residues ([25]). Compared with phenolic resin or other urea resins, suberin does not release toxic substances which are harmful to humans and the environment during its use. Suberin is a natural polymer which have a wide range of sources, as it can be extracted from waste bark, cork processing residue, etc. A reasonable reuse of cork residues can reduce the waste of resources and realize their high-value utilization. Therefore, the exploitation of these industrial by-products to modify fast-growing wood has vital practical significance for the reuse of bioresources and recycle of modified timber.
Suberin monomers (SMs) can be isolated from cork by depolymerizing suberin using alkaline hydrolysis ([16], [14], [32]). The main components of SMs are long-chain aliphatic acids, which is about 80-90% in mass of all the monomers obtained ([17]). The typical representatives in long-chain aliphatic acids are ω-hydroxyacids and α,ω-diacids, with chain length from C16 to C26 ([16]). In some long-chain aliphatic acids, the chain length can even reach C30 ([38]). On the other hand, there are a few polyaromatics monomers in SMs, such as ferulic acid, tyramine, etc., which make up from 1% to 10% of all suberin depolymerized products that have been found ([5], [17], [16]). The long-chain aliphatic acids and polyaromatic monomers are believed to have potential for improving wood properties. Long-chain aliphatic acids can improve dimensional stability of wood for its hydrophobicity ([16]), and polyaromatic monomers improve wood performance in anti-weathering due to the benzene ring in the structure ([34]).
In this study, SMs were extracted from the bark of cork (Quercus suber L.) to enhance the dimensional stability of wood from fast-growing poplar (Populus cathayana), a typical species in Northern China. To improve wood dimensional stability, we used heat treatment which is expected to redistribute SMs and partly degrade hemicelluloses. The morphological alteration and chemical characteristics of treatments were analyzed by scanning electron microscopy (SEM), confocal laser scanning microscopy (CLSM), and Fourier transform infrared (FTIR) spectroscopy. The dimensional changes of the modified wood under five relative humidity and liquid water conditions were investigated, and anti-swelling efficiency (ASE) of the specimens were calculated. Further, ASE’ was used to investigate the modification mechanism of SMs treated wood ([31], [21]). The results from this study could expand the application of wood from fast-growing species, as well as prompt a high-valued reuse of suberin.
Materials and methods
Materials
Cork samples of Algerian oak (Quercus suber L.) trees were provided by Hebei Belin Cork Co, Ltd., and were used to extract SMs. Wood specimens (20×20×20 mm3) were cut from the sapwood of fast-growing poplar (Populus cathayana) harvested from the Greater Khingan Mountains in China. Dichloromethane, ethanol, deionized water, methanol, sodium methoxide were obtained from the market.
Modification process
Preparation of modifier
First, the extract of cork was removed according to Sen et al. ([39]). The cork samples were ground and sieved to 40-60 mesh. Extractives of samples were obtained by sequential Soxhlet extractions with dichloromethane, ethanol, and deionized water by 6 h with each solvent to completely remove the extract.
SMs were prepared with the extractive-free material by alkaline methanolysis ([35], [30]). Extractive-free material was refluxed with a 3% methanolic solution of NaOCH3 in CH3OH during 3h. The sample was filtrated and washed with methanol. The residue was refluxed with CH3OH for 15 min and filtrated again. The combined filtrates were acidified to pH 6 with 2 M H2SO4 and evaporated to dryness. The solid was dissolved in deionized water, and a modifier with a mass fraction of 8% was obtained.
Specimens treatment
Wood specimens were impregnated with SMs solution using a vacuum-pressure process. Firstly, the specimens were oven-dried at 103 °C, and their weights were recorded. They were then subjected to vacuum of -0.1 MPa for 30 min and immersed in SMs at 0.5 MPa for 1 h. After impregnation, the excess liquid on the surface of wood was wiped with tissue paper. Then the specimens were oven-dried at 103 °C (labelled as “Sub”). Finally, the Sub specimens were treated at 180 °C for 2 h (labelled as “Sub 180 °C”). The weight percent gain (WPG) and bulking coefficient (BC) for the impregnation treatment and mass loss (ML) for the heat treatment were evaluated according to the following equations (eqn. 1, eqn. 2,eqn. 3):
where m0 and m1 are the oven-dried weights of each wood specimen before and after impregnation (g); m2 is the oven-dried weights of each wood specimen after heat treatment (g); v0 and v1 are the oven-dried volumes of each wood specimen before and after impregnation (mm3).
Scanning electron microscope analysis
The microstructure of specimens was observed by scanning electron microscope (JSM-6700F®, Jeol Ltd., Tokyo, Japan). The transverse sections of the specimens were sputter-coated with gold and observed by SEM with a voltage of 15KV.
Confocal laser scanning microscopy analysis
Suberin and lignin are naturally fluorescent substances in plant cells for its phenolic structure ([23], [24]). The microscopic distribution of SMs in specimens was detected by a Leica TCS SP8 confocal microscope (Leica Microsystems Inc., Wetzlar, Germany) with a krypton/argon laser emitting at wavelengths of 488 nm. The transverse sections of the specimens with a thickness of 10-20 μm was obtained using a microtome, and the gain setting was 115. The fluorescence intensity on the cell cavity and cell wall was measured and compared to the difference before and after treatment with the average value of 5 points.
Fourier transform infrared analysis
Some 200 mg of KBr powder and 1-2 mg were taken and ground in an agate mortar. The sample was pressed using a tablet press and put into the cuvette for testing. The change of functional groups in the specimens after treatment was tested by Fourier transform infrared spectroscopy (Spectrum Two™, Perkin-Elmer Inc., Waltham, MS, USA) with a resolution of 4 cm-1 and 32 scans per specimen in the 400-4000 cm-1 interval.
Hygroscopicity and dimensional stability
Oven-dried specimens were separately exposed to 5 different relative humidities (RHs) of 16%, 36%, 60% and 83%. The 5 RH conditions were developed by saturated salt solutions of LiCl, MgCl, NaBr, KBr, and KNO3 at 25 °C ([18]). Each treatment collectives have 4 wood replicates for all the RHs. Six additional oven-dried specimens were placed in deionized water at approximately 25 °C for liquid water uptake in each group. Weights and dimensions along and across the grain during the process were measured at specific time intervals, by analytical balance (0.0001 g) and vernier caliper (0.02 mm) at the same positions marked in the specimens, respectively.
Moisture content (MC), tangential swelling rate (TSR), and volumetric swelling rate (VSR) of the specimens were calculated as follows (eqn. 4 to eqn. 8):
where the subscript ad represents the water vapor uptake process, ab refers to the liquid water uptake process; M0 and Mi are the weights of oven-dried treated specimens and specimens after n hours of water vapor uptake (g), respectively; L0, Lad, and Lab are the tangential dimensions of oven-dried treated specimens and specimens after n hours of water vapor uptake and liquid water uptake (mm), respectively; V0, Vad, and Vab are volumes of oven-dried treated samples and specimens after n hours of water vapor uptake and n hours water immersion (mm3).
Anti-swelling efficiency (ASE) of the specimens was calculated according to eqn. 9. To further investigate the modification mechanism at the cellular level, ASE′ was evaluated using eqn. 10 ([31], [21]):
where VSRu and VSRs are the volumetric swelling rates of untreated wood and treated wood based on the oven-dried dimension after treatment, while VSRs′ is the volumetric swelling coefficient of treated wood calculated by using the oven-dried dimension before treatment.
According to Hill ([21]), there are two mechanisms in the improvement of wood dimensional stability. When reagent is not cross-linked with wood cell wall, the achievement of dimensional stability is attributed to cell wall swelling (bulking) caused by the reagent; otherwise it could be due to the restrained movement of cell wall polymer. The dimensional stability calculated by ASE cannot differentiate the effect of bulking from crosslinking, whereas ASE′ could eliminate the influence of reagent filling by changing the calculation method for volumetric swelling rate of the treated wood. In the calculation of ASE′, the oven-dried dimension before treatment is used in the VSRs′, then the effect of swell by reagent filling is removed. Therefore, it can further determine whether the cross-linking reaction has occurred from the results of ASE′.
The effects of modifiers on wood cell wall could have 3 cases: (i) when the improved dimensional stability of the modified wood is caused by a bulking phenomenon, then ASE′ will be zero (Fig. S1 in Supplementary material); (ii) when the cross-linking occurs, then the liquid water uptake volume of the modified wood will be lower than bulking only, so ASE′ takes a positive value; (iii) if the modification results in cell wall degradation, which leads to an increase in volume, then a negative value of ASE′ will be obtained ([31], [21]).
Results and discussion
General description of treated wood
As shown in Tab. 1, the WPG and BC of wood specimens subjected to the SMs treatment were 14.27% and 3.32%, respectively. A part of SMs was introduced into the wood cell and it bulked after vacuum/pressurize treatment. The WPG and BC of Sub 180 °C were 10.14% and 1.89%, respectively, and the average mass loss for specimens of this treatment was 2.28%, due to the degradation of hemicellulose and volatilization of low molecular mass monomers in SMs.
Tab. 1 - Mean (± standard deviation) weight percent gain, bulking coefficient, and mass loss of the treated specimens (based on 21 replicates).
Treatment | Weight percent gain (%) |
Bulking (%) |
Mass loss (%) |
---|---|---|---|
Sub | 14.27 ± 0.01 | 3.32 ± 0.01 | - |
Sub 180°C | 10.14 ± 0.03 | 1.89 ± 0.01 | 2.28 ± 0.00 |
Microstructure of the specimens
Fig. 1 shows the SEM images of honeycomb cell structure in the cross-sections of specimens. SMs entered the wood cells by vacuum/pressurize treatment at room temperature, and deposited as particles (Fig. 1e-h). Compared with the Control, a part of the cell lumen of wood vessels and fibers were totally or partially filled with SMs after impregnation (Fig. 1e, Fig. 1f), which blocked the water channels within wood. SMs in granular or membranous form created a barrier reducing the contact between wood and water. After heat treatment at 180 °C, the cell structure remained intact, implying the heat treatment temperature and time were moderate. Comparing Fig. 1c with Fig. 1g, the cell wall of Sub 180 °C was significantly thicker than that of Control 180 °C, due to the uniform attachment of SMs to the inner cell wall after heat treatment. The SMs particles in Fig. 1h was smoother than Fig. 1f, which proved that the SMs particles had fluidity with heat treatment again ([40]).
Fig. 1 - SEM images of wood cross-sections for the Control (a, b), Control 180 °C (c, d), Sub (e, f) and Sub 180 °C (g, h) specimens. Magnification of 500× for (a), (c), (e) and (g), and 4000× for (b), (d), (f) and (h).
SMs distribution in the specimens
Fig. 2 illustrates the CLSM images of wood specimens from Control, Sub, and Sub 180 °C treatments. The aromatic compounds have a fluorescent effect under CLSM, and brighter areas represent higher aromatic concentration ([26]). In Fig. 2a, the green light fluorescence in the compound middle lamella was brighter than cell wall, suggesting the presence of more lignin in the compound middle lamella. Fig. 2also shows the average fluorescence intensity estimated by five random points from the cell wall or cell lumen. In the Control, the average intensity was 48.23 for the cell wall, and 1.21 for cell lumen. For the treatments Sub and Sub 180 °C, both the brightness of the image and fluorescence signal intensity significantly increased compared with the Control. The intensity was 118.96 for cell wall and 7.08 for cell lumen in Sub, which indicated that SMs was not only deposited in the cell lumen but also entered into cell wall. Further compared to the Sub, the signal intensity curve demonstrates that the fluorescence intensity distribution was more homogeneous for Sub 180 °C, suggesting a uniform distribution of SMs after heat treatment, which agrees with the result from SEM. The results of the CLSM were consistent with previous studies. Kitin et al. ([24]) also reported the fluorescence of lignin and suberin in plant cells under CLSM. Heinämäki et al. ([20]) confirmed the melting behavior of suberin monomers. Therefore, the fluorescence of cell wall was brighter and SMs have a uniform distribution after heat treatment.
Fig. 2 - CLSM images showing the cross-sections of wood specimens for Control, Sub, and Sub 180 °C treatments and the corresponding intensity of fluorescence signal. (a): Average values (standard deviation in parenthesis) from five replicates.
Functional group changes of the specimens
Fig. S2 (Supplementary material) gives the FTIR spectra from 400 to 4000 cm-1 of the specimens. The signals from SMs were observed at 1425 cm-1 (C-H) and 1461 cm-1 (C-H) in Sub and Sub 180 °C ([33]). In addition, compared with the Control, three new signals appeared in Sub and Sub 180 °C, namely the peaks at 1116 cm-1 (C-O-C), 1507 cm-1 (aromatic ring skeleton), and 1598 cm-1 (COO), which proves that SMs entered into wood cells. In the research of Cordeiro et al. ([8]), the peak of C-H in 1463 cm-1 was also found. Besides, the peak at 1739 cm-1 (C=O) in the Control 180 °C and Sub 180 °C was reduced, which indicated that hemicellulose was degraded. Labbé et al. ([27]) reported the reduction of acetyl groups on hemicelluloses in 1736 cm-1 after heat treatment as well. The new bond was not find in spectra of Sub 180 °C, thus supporting the evidence that cross-linking occurred between SMs and wood.
Water vapor uptake of specimens
Fig. 3 presents the water vapor uptake processes at four RH conditions for the specimens. In low RH (16% and 36% RH), the moisture content of Sub was lower than Control. However, under high RH (60% and 83% RH), this relation was reversed.
Fig. 3 - Moisture water uptake processes under different RH conditions (at 25 °C) for the wood specimens subjected to different treatment.
The results of water vapor uptake for the SMs treated wood are similar to those of PEG modified wood. Bjurhager et al. ([4]) investigated the equilibrium moisture content (EMC) of PEG 600 modified oak wood under different RH, finding that it was lower than expected when RH was below 55%, while it was the opposite when RH was above 55%. The findings of Meints et al. ([29]) showed similar EMC results for PEG 400/PEG 1000 modified oak wood under high RH.
Both SMs and PEG have a large number of hydroxyl groups. As suggested by Bjurhager et al. ([4]), hydrogen bonding takes place between PEG and hydroxyl groups in the cell wall of PEG modified wood. Under different RH, the “PEG-wood” interface reacted differently to moisture. The mechanism could be deduced from PEG modified wood for their similar functional groups. When the RH was low, the water molecules cannot cut down the hydrogen bonds between the SMs and wood (Fig. 4b). In addition, SMs filled the cell wall pores, thereby reducing the contact of moisture with wood substance ([19]), and the hydrophobic long-chain aliphatic acids prevented water from entering the vessels ([16]); thus the SMs treated wood had a lower moisture content. Whereas under high RH conditions, the hydrogen bonds between the SMs and wood were broken (Fig. 4c), as water molecules bonded with the SMs and the hydroxyl groups in the wood cell wall; therefore, the moisture content of Sub treated wood was higher than the Control.
Fig. 4 - Schematic diagram of the interaction among SMs, wood, and water. (a): Hydrogen bonding between SMs and wood; (b): interactions among water molecules, SMs, and wood in low RH; (c): interactions among water molecules, SMs, and wood in high RH.
The moisture content of the Control 180 °C specimens was reduced during the water vapor uptake process due to the degradation of hemicellulose (Fig. 3). At the same time, the moisture content of Sub 180 °C wood was also reduced. Compared with Control, the moisture content of Sub 180 °C specimens was reduced by 35.6%, 46.76%, 15.56%, and 5.74% under RH of 16%, 36%, 60%, 83% at 528 h, respectively. Regarding the moisture content, the relationship between Sub 180 °C and Control 180 °C treatments was similar to that between Sub and Control treatments. The moisture content of impregnated specimens was lower than that of unimpregnated ones at 36% RH, and the phenomenon was the opposite at high RH. This indicates that the “SMs-wood” interface existed in Sub 180 °C wood, thus the hydroxyl groups in SMs were still present after heat treatment. The moisture content of Control 180 °C specimens was lower than that of Sub 180 °C specimens under RH of 60%, but this relation was reversed for the Control and Sub specimens. This support the hypothesis that the interface of “SMs-wood” in Sub 180 °C samples was weaker than that of Sub samples, due to the degradation of hemicellulose after heat treatment.
Dimensional stability of the specimens
Fig. S3 (Supplementary material) presents the tangential and volumetric swelling rate of specimens under different RHs. After heat treatment, the tangential and volumetric swelling rate of Control 180°C wood was significantly lower than Control wood, and the tangential and volumetric swelling rate of Sub 180 °C was lower than the Control. Specifically, the tangential swelling rate was reduced by 13.18% to 24.63% at 528 h, and the volumetric swelling rate was reduced by 12.24% to 34.00% at 528 h. With the heat treatment, the SMs were more evenly distributed in the wood cells (Fig. 1), and this reduced the contact between water and wood. Compared with the Control 180°C samples, the Sub 180°C sample wood had a lower volumetric swelling rate at 16% RH, 36% RH, and 60% RH. However, under 83% RH, the volumetric swelling rate of Sub 180°C specimens was similar to the of Control 180°C specimens. Cui et al. ([9]) studied the dimensional changes of ZnO composite heat treatment wood under 65% RH, finding that it had 1.6% tangential and 2.2% volumetric swelling. In this study, the swelling rate of Sub 180 °C wood under 60% RH was lower than the aforementioned ZnO composite heat treatment modified wood. Therefore, SMs compound after heat treatment can better prevent wood from absorbing water vapor.
Fig. 5 presents the tangential and volumetric swelling rate of specimens through liquid water uptake. The swelling rate of Sub samples was reduced by 29.98% compared to Control samples, because SMs filled in cell lumen and cell wall. Concerning the Sub 180 °C treatment, the dimensional stability was further enhanced compared with Control, as the tangential and volumetric swelling rate of Sub 180 °C specimens was reduced by 21.37% and 32.85% at 192h. Bulking of cell wall by SMs, better distribution of SMs within wood cell wall, and the degradation of hemicellulose after heat treatment, are supposed to be the reasons accounting for the most favorable dimensional stability of the Sub 180 °C treated wood. Baar et al. ([2]) reported a hemp oil composite heat-treated wood, which tangential and volumetric swelling rate were about 8.5% and 15.0% through liquid water uptake. In this study, Sub 180°C wood had a lower tangential and volumetric swelling rate, which were 6.9% and 8.9%, respectively.
Fig. 5 - Tangential (a) and volumetric (b) swelling rate of the specimens through liquid water uptake.
Tab. 2 lists the ASE and ASE′ of the specimens subject to different treatments. At WPG of 13.2%, the ASE of the Sub specimens was 30.0%, and that of the Sub 180 °C specimens was 49.6%, which once again indicate higher dimensional stability. The ASE′ of glutaraldehyde modified wood was 18% with 14% WPG ([41]). Compared with glutaraldehyde modified wood, that of Control 180 °C, Sub and Sub 180 °C modified wood could be considered as 0, suggesting the SMs just impregnated the cell wall and coated the inner surface of cell lumen without cross-linking with the cell wall substance in the Sub and Sub 180 °C wood (Fig. 2). Thermosetting resin modified wood was reported by many researchers ([10], [31]). An average ASE value of 45.6% was achieved for phenol formaldehyde (PF) modified wood with 13.8% WPG ([31]). This study proved that SMs and PF have similar effects on wood ASE. However, PF modified wood have a different mechanism underlying the dimensional stability in respect to Sub 180 °C modified wood (0.2% ASE′ for Sub 180 °C, 21.5% ASE′ for PE modified wood).
Tab. 2 - ASE and ASE′ of specimens in 192h during liquid water uptake from 6 replicates.
Parameter | Treatment | ||
---|---|---|---|
Control 180°C | Sub | Sub 180°C | |
ASE (%) | 18.1 ± 6.61 | 30.0 ± 4.28 | 49.6 ± 4.12 |
ASE′ (%) | 0.2 ± 0.06 | -0.0 ± 0.07 | 0.2 ± 0.07 |
Conclusions
To improve the dimensional stability of wood from fast-growing species, an effective and sustainable modification approach was reported here. Poplar specimens were impregnated with SMs and then subjected to heat treatment. Compared with Control, the volumetric swelling rate of Sub and Sub 180 °C modified wood was significantly reduced. SMs filled in cell lumen, which not only blocked the vessels within wood but also reduced the contact between wood and water. In addition, SMs could enter into the wood cell wall and bulked it, so as to reduce its swelling. After heat treatment, the distribution of SMs became much more uniform and part of hemicelluloses was degraded. The dimensional stability of modified wood was further enhanced by the heat treatment.
The SMs modified wood has excellent performances and is a fully green product, which can be used as an ecologically friendly material. Future research will focus on methods based on acids and biological enzymes aimed to polymerize SMs in the wood and reduce the water vapor uptake of SMs modified wood under high relative humidity. Besides, long-chain aliphatic acids and polyaromatic monomers of the SMs can be separated and wood can be modified with long-chain aliphatic acids or polyaromatic monomers, aiming at different purposes, to further realize an efficient and reasonable utilization of SMs.
Acknowledgments
This work was supported by the Fundamental Research Funds for the Central Universities of China (No. 2015ZCQ-CL-01). The authors would also like to thank Xingang Zhao, general manager of Heibei Belin Cork Co., Ltd., for providing cork samples.
References
Gscholar
Gscholar
Authors’ Info
Authors’ Affiliation
Erni Ma 0000-0001-9774-9779
MOE Key Laboratory of Wooden Material Science and Application, Beijing Forestry University, Qinghua Eastroad 35, Haidian 100083, Beijing (China)
Corresponding author
Paper Info
Citation
Zhang R, Ma E (2021). Improving dimensional stability of Populus cathayana wood by suberin monomers with heat treatment. iForest 14: 313-319. - doi: 10.3832/ifor3684-014
Academic Editor
Giacomo Goli
Paper history
Received: Oct 27, 2020
Accepted: Apr 19, 2021
First online: Jul 01, 2021
Publication Date: Aug 31, 2021
Publication Time: 2.43 months
Copyright Information
© SISEF - The Italian Society of Silviculture and Forest Ecology 2021
Open Access
This article is distributed under the terms of the Creative Commons Attribution-Non Commercial 4.0 International (https://creativecommons.org/licenses/by-nc/4.0/), which permits unrestricted use, distribution, and reproduction in any medium, provided you give appropriate credit to the original author(s) and the source, provide a link to the Creative Commons license, and indicate if changes were made.
Web Metrics
Breakdown by View Type
Article Usage
Total Article Views: 32447
(from publication date up to now)
Breakdown by View Type
HTML Page Views: 27955
Abstract Page Views: 2233
PDF Downloads: 1776
Citation/Reference Downloads: 4
XML Downloads: 479
Web Metrics
Days since publication: 1460
Overall contacts: 32447
Avg. contacts per week: 155.57
Article Citations
Article citations are based on data periodically collected from the Clarivate Web of Science web site
(last update: Mar 2025)
Total number of cites (since 2021): 3
Average cites per year: 0.60
Publication Metrics
by Dimensions ©
Articles citing this article
List of the papers citing this article based on CrossRef Cited-by.
Related Contents
iForest Similar Articles
Research Articles
Kinetic analysis of poplar wood properties by thermal modification in conventional oven
vol. 11, pp. 131-139 (online: 07 February 2018)
Research Articles
Heat treatment of poplar plywood: modifications in physical, mechanical and durability properties
vol. 16, pp. 1-9 (online: 09 January 2023)
Research Articles
Thermo-modified native black poplar (Populus nigra L.) wood as an insulation material
vol. 14, pp. 268-273 (online: 29 May 2021)
Research Articles
Physical-mechanical properties and bonding quality of heat treated poplar (I-214 clone) and ceiba plywood
vol. 8, pp. 687-692 (online: 17 December 2014)
Research Articles
Physical and mechanical characteristics of poor-quality wood after heat treatment
vol. 8, pp. 884-891 (online: 22 May 2015)
Research Articles
Examining the evolution and convergence of wood modification and environmental impact assessment in research
vol. 10, pp. 879-885 (online: 06 November 2017)
Short Communications
Influence of thermo-vacuum treatment on bending properties of poplar rotary-cut veneer
vol. 10, pp. 161-163 (online: 13 June 2016)
Research Articles
Hardness and contact angle of thermo-treated poplar plywood for bio-building
vol. 14, pp. 274-277 (online: 29 May 2021)
Review Papers
Moisture in modified wood and its relevance for fungal decay
vol. 11, pp. 418-422 (online: 05 June 2018)
Technical Notes
Improving impregnation properties of fir wood to acid copper chromate (ACC) with microwave pre-treatment
vol. 8, pp. 89-94 (online: 01 April 2014)
iForest Database Search
Search By Author
Search By Keyword
Google Scholar Search
Citing Articles
Search By Author
Search By Keywords
PubMed Search
Search By Author
Search By Keyword