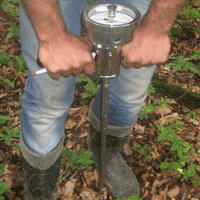
Influence of slope on physical soil disturbance due to farm tractor forwarding in a Hyrcanian forest of northern Iran
iForest - Biogeosciences and Forestry, Volume 7, Issue 5, Pages 342-348 (2014)
doi: https://doi.org/10.3832/ifor1141-007
Published: Apr 17, 2014 - Copyright © 2014 SISEF
Research Articles
Abstract
Tractor-based systems are the most common type of small-scale timber harvesting equipment in the Hyrcanian forest, where farm tractors equipped with a 2-wheel trailer typically perform forwarding operations for pulpwood and fuelwood. The objective of this study was to evaluate the effects of different frequencies of machine passes on soil bulk density (BD), penetration resistance (PR) and total porosity (TP) in different slope gradient and forwarding direction (i.e., uphill vs. downhill). The study, performed on a brown soil with a silt loam to loamy texture, was designed as a factorial experiment with three slope gradient/forwarding direction classes (0-10% downhill forwarding slope, 10-20% downhill forwarding slope and 0-10% uphill forwarding slope) and four traffic intensity classes (0 [undisturbed control area], 1-4, 5-10 and >10 machine passes). Responses were assessed at four soil profile depth classes (5, 10, 15 and 20 cm). BD and PR significantly increased and TP significantly decreased with increasing machine traffic, with magnitudes of change that were not consistently greater in the upper 5 cm compared to the 20 cm soil profile depth class. Whereas the majority of changes in BD and TP occurred after fewer than 5 passes, large increases in PR occurred even after 10 passes. Changes of BD, PR and TP in response to machine traffic differed significantly among slope gradient/forwarding direction classes. Increases in BD and PR and decreases in TP were largest on the 0-10% uphill forwarding slope, followed by the 10-20% downhill and 0-10% downhill forwarding slope. We conclude that the application of farm tractors in forwarding operations should be limited to gentle slopes and uphill forwarding with these machines should be avoided.
Keywords
Farm Tractor, Forwarding Operation, Soil Compaction, Penetration Resistance, Total Porosity
Introduction
The entry of forestry machineries into forest has resulted in some cases in levels of soil disturbance that may have adverse effects on forest ecosystems and subsequently on long-term tree growth ([27], [25], [21], [29]). Numerous studies have documented changes in physical soil properties following harvesting machine traffic, such as increased soil compaction, soil displacement, rutting and soil profile disturbances ([1], [44], [32], [34], [12], [40], [13], [20], [42], [46], [4], [3], [31]). For instance, soil compaction, in which soil pores (especially macropores) are destroyed and surface aggregates are broken down ([4]), depend on the soil texture and moisture at the time of harvest ([26], [6]), but also on the number of machines passing on a specific point. Generally, soil compaction occurs in the first ten passes of a vehicle, but most of the compaction occurs in the first three trips; subsequent passes generally have little additional effect ([16], [12], [13], [4]). So it would be appropriate to concentrate machine traffic on carefully designed skid trails in such a way to minimize the affected area and leave most trees undisturbed ([45], [4]).
It is unclear, however, to what extent the slope gradient (i.e., longitudinal and transversal slope) of the skid trail and the direction of machine traffic (i.e., uphill vs. downhill) could modify the impacts of vehicle traffic on the soil. Whereas some studies have failed to document an effect of slope on bulk density following harvesting traffic (e.g., [38], [22]), others have shown that soil disturbances increase with slope gradient ([14]), possibly because machines slip more and remain in a given place for a longer time on steep terrain, causing soil pulling and dragging ([16]). Thus, steepness may have an even stronger effect on soil disturbance than that of the soil moisture ([24]), but possibly less so than that of the traffic intensity ([14]).
Soil disturbance is also strongly affected by the type of harvesting equipment used. Small-scale harvesting technology, such as farm tractors or better small skidder or other specific machines (such as ATV), is thought to result in lower levels of residual stand and soil damage because the equipment is typically smaller and lighter than regular skidders ([41], [36], [39]). A recent study dealing with soil compaction has found that the highest relative increase in bulk density was associated with the use of bulldozers, the lowest with the use of a forwarder, and intermediate levels with the use of a farm tractor and a rubber-tired cable skidder ([9]). However, farm tractors have been designed primarily for use in an agricultural rather than a forest environment and must be modified for use as wheeled skidders, such as through the addition of a logging winch, a roll-over canopy, a blade, belly pan protection, valve stem protection, wheel chains and wheel weights ([10], [33], [37], [11], [19], [35]).
In the Hyrcanian forest of northern Iran, where rough topography and use of the sheltercut system favor small-scale harvesting operations, farm tractors often pull an additional two-wheeled trailer to forward the wood raised completely off the ground. The felling and on-site processing of felled trees with large diameters are typically done by chainsaw ([23]). Mechanization is largely limited to the transport function, whereby logs of 5-15 meter length are extracted with rubber-tired skidders to roadside landings and farm tractors and mules are the most common timber extraction systems for pulpwood and fuelwood. The advantages of using relatively light farm tractors with large-dimension tires in the rear axle is the large contact area of the tire with the soil and the reduced ground pressure. Although the addition of a trailer increases the payload per trip, thereby reducing the number of equipment passes with its associated soil disturbances, the trailer also reduces tractor maneuverability, potentially creating deep ruts in steep terrain and in uphill forwarding.
We hypothesized that the extent of the soil degradation from farm tractor forwarding operations along the upper 20 cm of the soil profile would increase with traffic frequency, but that these increases would vary with slope gradient and direction of forwarding (uphill or downhill). To test this hypothesis, we quantified soil bulk density, penetration resistance and total porosity in skid trails on different slope gradients with varying forwarding direction and traffic intensities.
Materials and methods
Study sites
The research was carried out in Compartments 311 and 319 of the Gorazbon district in the Kheyrud Forest in the Hyrcanian forest region of northern Iran. The studied forests range in elevation from 1050-1270 m a.s.l. with a prevailing southern aspect. Average rainfall ranges from 1150 to 1260 mm year-1, with peaks in summer and autumn. Average daily temperatures range from a few degrees below 0 °C in December, January, and February to +25 °C during the summer. Soils are classified as well-drained forest brown soils (Alfisols) with a soil texture ranging from silt loam to loamy. The relief of the study area is characterized by carbonate rocks (limestone and dolomite) and their numerous karst forms: crests, carks in limestone, under-ground flows and sink hole structure. The study area is dominated by natural forests with native mixed deciduous tree species, including Fagus orientalis Lipsky, Carpinus betulus L., Alnus subcordata C.A.Mey, and Quercus castaneifolia C.A. Mey. The prevalent silvicultural treatment is a combination of the group selection and single tree selection methods, resulting in uneven-aged stands. Based on field data collected in October 2012, the average growing stock in compartments 311 and 319 was 289.1 m3 ha-1 and 555.2 m3 ha-1, respectively. A total of 240 trees (9 trees ha-1) with a total volume of 940.2 m3 (33.8 m3 ha-1) were marked in compartment no. 311 and a total of 210 trees (5 trees ha-1) with a total volume of 1177.3 m3 (27.5 m3 ha-1) were marked in compartment no. 319.
Experimental design and data collection
A farm tractor equipped with a 2-wheel trailer (2-driving wheel) was used for forwarding pulp- and fuelwood. Technical specifications of the farm tractor were: UTB/ Universal 650 Engine UTB, diesel, 4-cylinder; Displacement: 4.8 l; Fuel capacity: 98.0 l; Mass: 3999 kg; Engine Power: 46.2 kW; Length: 414 cm; Width: 199 cm; Height: 260 cm; 4WD; and trailer Mass: 1700 kg. At the time of forwarding, weather conditions had been very dry and warm, and these conditions remained constant during the extraction operation. The soil moisture was 20-65% and the organic matter content was 11%. The slope of the skid trail ranged from 0 to 20% and extraction distances to the landing area near the forest roadside were about 220 m. A Suunto inclinometer was used for measuring slope in the skid trails. Calculation of slope was weighted as follows ([28] - eqn. 1):
where S
is the average slope along the skid trail (percent), d
is the distance between two points in the sample (m), s
is the slope between two points in the sample (percent), i
is the sample number, and n
is the number of samples.
The average forwarded volume in each trip was 2280 kg. Sampling transects were placed on different slope gradient classes along the designated skid trail (Fig. 1a). To ensure that traffic was restricted to the same tracks, painted sticks were implanted in the center of the skid trail as a guide for machinery operators. Before the forwarding operation with the farm tractor began, sampling plots were established in different skid trail segments on three slope gradients (i.e., 0-10% downhill, 10-20% downhill, and 0-10% uphill forwarding) that would be exposed to different levels of machine traffic, i.e., 0 (undisturbed), 1-4, 5-10 and >10 machine passes. A machine pass implies a drive both back and forth (i.e., with and without a wood load) on the selected trail segment. Each combination of slope by traffic frequency was replicated three times. Prior to and after the forwarding operations, soil response measurements (i.e., bulk density, resistance to penetration and total porosity) were obtained in each replicate at soil depths of 5, 10, 15 and 20 cm in the wheel rut as well as in control sample points adjacent to the skid trail (Fig. 1a). Organic horizons were removed from the soil surface prior to bulk density and penetration resistance measurements, so that measurements were referenced to the mineral soil surface. Soil sample cores were taken from the top mineral soil using a thin walled steel cylinder, 40 mm long and 56 mm in diameter, driven into the soil by a hammer-driven device. After extracting the steel cylinder, soil cores were trimmed flush with the cylinder end and extruded into a plastic bag for transport to the laboratory. Samples were weighed on the day they were collected and again after oven-drying at 105 °C for 24 h to determine water content and bulk density. Soil penetration resistance (PR
) was measured using a hand-held soil penetrometer (Fig. 1b). The study yielded 144 PR
readings (samples) before and after treatment. When the penetrometer had hit a buried rock or tree roots, the readings were ignored.
Fig. 1 - Experimental design of the field trail for the BD and PR sampling (a) and measurement of PR using a hand-held penetrometer (b).
Soil porosity (TP
) was determined by the following equation (eqn. 2):
where PD
is the particle density measured by a pycnometer on the same soil samples used to determine the bulk density (BD
). A pycnometer is a device used to determine the density of a liquid. Such instrument is usually made of glass, with a close-fitting ground glass stopper with a capillary tube through it, so that air bubbles may escape from the apparatus. This device enables a liquid’s density to be measured accurately by reference to an appropriate working fluid, such as water or mercury, using an analytical balance. Particle density (PD) was calculated as follows (eqn. 3):
where dw
is the density of water (g cm-3) at the temperature observed, Ws
is the weight of soil sample (oven dry), Wsw
is the weight of pycnometer, soil and water, and Ww
is the weight of pycnometer and water.
Statistical analysis
The experimental design was a factorial arrangement of treatments conducted in a completely randomized design. We applied general linear modeling (GLM) to relate bulk density, penetration resistance and total porosity to the slope gradient (three classes), machine passes (four classes), soil depth (four classes) and their interactions. Since no departure from normal distribution of the data was observed after Kolmogorov-Smirnov test (α = 0.05), standard parametric analyses were carried out. Homogeneity of variance among treatments was verified by the Levene’s test (α = 0.01). Post-hoc comparison of group means was performed using the Duncan’s multiple range test with a 99% confidence level. Treatment effects were considered significant when P<0.01.
All statistical analyses were carried using the SPSS (release 15.0) statistical package.
Results
Bulk density
Slope gradient (SG), traffic intensity (T) and soil profile depth (D - depth readings were referenced to the mineral soil surface), as well as the interaction effects of SG×T, SG×D, SG×D and SG×T×D significantly affected BD
(Tab. 1 and Tab. 2). Average BD
varied significantly among slope gradients and ranged from 1.20 g cm-3 on the 0-10% downhill forwarding slope, 1.35 g cm-3 on the 10-20% downhill forwarding slope and 1.46 g cm-3 on the 0-10% uphill forwarding slope. Average BD
increased significantly with increasing frequency of machine passes and reached the highest level of 1.45 g cm-3 after 10 passes. Average BD
also increased significantly with increasing soil profile depth. However, the major increases following forwarding occurred at the soil surface (0-5 cm), particularly on the 0-10% uphill forwarding slope and the 10-20% downhill forwarding slope.
Tab. 1 - Means ± standard deviation of different physical soil properties on skid trails after forwarding with a farm tractor. (BD
): bulk density; (TP
): total porosity; (PR
): penetration resistance. Different letters indicate statistically significant differences within columns for each slope class separately (P<0.01), based on analysis of variance (GLM).
Slope (%) |
Traffic (# of passes) |
Depth (cm) |
BD (g cm-3) |
PR (kPa) |
TP (%) |
---|---|---|---|---|---|
0-10 % (down) |
0 | 0-5 | 1.02 ± 0.01d | 1367.7 ± 35.7b | 55.3 ± 1.1a |
5-10 | 1.09 ± 0.02c | 1366.0 ± 24.2b | 53.6 ± 4a | ||
10-15 | 1.18 ± 0.01b | 1382.7 ± 16.2ab | 51.1 ± 2.7a | ||
15-20 | 1.23 ± 0.01a | 1429.3 ± 19a | 45.3 ± 1.7b | ||
<5 | 0-5 | 1.09 ± 0.01d | 1487.3 ± 2.9a | 53.5 ± 2.5a | |
5-10 | 1.17 ± 0.01c | 1470.3 ± 44.7a | 49.4 ± 1.6ab | ||
10-15 | 1.21 ± 0.02b | 1496.7 ± 86.6a | 43.7 ± 6.5b | ||
15-20 | 1.28 ± 0.01a | 1562.3 ± 48.1a | 44.8 ± 3.3b | ||
5-10 | 0-5 | 1.07 ± 0.01d | 1614.0 ± 45.6a | 55.0 ± 1.6a | |
5-10 | 1.21 ± 0.02c | 1553.7 ± 18.3ab | 45.6 ± 0.7b | ||
10-15 | 1.25 ± 0.01b | 1541.3 ± 43b | 44.4 ± 1.7b | ||
15-20 | 1.33 ±0.01a | 1498.0 ± 18.2b | 40.0 ± 2.9c | ||
>10 | 0-5 | 1.15 ± 0.02c | 1595.7 ± 27.4b | 48.8 ± 1.7a | |
5-10 | 1.25 ± 0.01b | 1643.3 ± 20.8ab | 47.1 ± 1.9a | ||
10-15 | 1.34 ± 0.03a | 1690.0 ± 45.8a | 43.3 ± 2.2a | ||
15-20 | 1.37 ± 0.04a | 1695.3 ± 32.3a | 44.3 ± 6.4a | ||
10-20 % (down) |
0 | 0-5 | 1.03 ± 0.01d | 1343.3 ± 32.1c | 56.2 ± 2.4a |
5-10 | 1.09 ± 0.01c | 1366.7 ± 30.6c | 53.8 ± 3.7ab | ||
10-15 | 1.19 ± 0.01b | 1433.3 ± 28.9b | 50.7 ± 2.3bc | ||
15-20 | 1.24 ± 0.01a | 1486.7 ± 5.8a | 47.2 ± 2.4c | ||
<5 | 0-5 | 1.23 ± 0.02d | 1672.0 ± 41.1b | 47.6 ± 2.4a | |
5-10 | 1.37 ± 0.01c | 1676.7 ± 11.6b | 41.2 ± 1.7b | ||
10-15 | 1.43 ± 0.02b | 1717.7 ± 42.4ab | 37.5 ± 1.8b | ||
15-20 | 1.47 ± 0.03a | 1778.7 ± 51.4a | 38.2 ± 4.6b | ||
5-10 | 0-5 | 1.29 ± 0.02d | 1763.3 ± 36.1a | 45.8 ± 6.4a | |
5-10 | 1.41 ± 0.02c | 1776.7 ± 38.3a | 43.3 ± 1.1a | ||
10-15 | 1.45 ± 0.01b | 1752.0 ± 18.4a | 40.4 ± 1.3ab | ||
15-20 | 1.52 ± 0.01a | 1807.7 ± 51.9a | 33.6 ± 3.9b | ||
>10 | 0-5 | 1.36 ± 0.03c | 1895.3 ± 49.5a | 41.2 ± 3.0a | |
5-10 | 1.45 ± 0.01b | 1943.7 ± 37.6a | 39.1 ± 5.4a | ||
10-15 | 1.51 ± 0.02a | 1916.7 ± 30.6a | 34.0 ± 7.8a | ||
15-20 | 1.52 ± 0.01a | 1953.3 ± 15.3a | 35.5 ± 3.0a | ||
0-10 % (up) |
0 | 0-5 | 1.04 ± 0.02d | 1376.7 ± 20.8b | 57.8 ± 0.9a |
5-10 | 1.10 ± 0.01c | 1356.7 ± 32.1ab | 50.8 ± 1.0b | ||
10-15 | 1.17 ± 0.02b | 1380.0 ± 17.3ab | 48.7 ± 1.6bc | ||
15-20 | 1.24 ± 0.01a | 1423.3 ± 25.2a | 47.1 ± 3.0c | ||
<5 | 0-5 | 1.41 ± 0.02a | 1577.0 ± 28.6c | 38.1 ± 5.5a | |
5-10 | 1.50 ± 0.02b | 1674.0 ± 50.5b | 30.8 ± 4.4ab | ||
10-15 | 1.57 ± 0.01a | 1727.3 ± 34.1b | 35.8 ± 2.5ab | ||
15-20 | 1.58 ± 0.02a | 1826.7 ± 23.1a | 29.9 ± 2.7b | ||
5-10 | 0-5 | 1.49 ± 0.02c | 1953.3 ± 15.3c | 34.0 ± 1.2a | |
5-10 | 1.58 ± 0.01b | 2067.3 ± 50.0b | 30.1 ± 2.2a | ||
10-15 | 1.61 ± 0.03ab | 2078.3 ± 44.1b | 26.6 ± 2.7ab | ||
15-20 | 1.64 ± 0.02a | 2166.7 ± 54.6a | 30.7 ± 1.8b | ||
>10 | 0-5 | 1.55 ± 0.02b | 2197.7 ± 81.0a | 34.1 ± 1.8a | |
5-10 | 1.63 ± 0.02a | 2152.7 ± 10.5a | 34.8 ± 4.7a | ||
10-15 | 1.63 ± 0.02a | 2196.7 ± 70.1a | 30.8 ± 3.9a | ||
15-20 | 1.65 ± 0.02a | 2193.0 ± 37.4a | 30.2 ± 4.5a |
Tab. 2 - Analysis of variance (ANOVA) for the effects of slope gradient (SG), traffic intensity (T), soil profile depth (D) classes and their interactions on BD
after forwarding with a farm tractor.
Source | Sum of Squares |
df | Mean Square |
F-value | P-value |
---|---|---|---|---|---|
SG | 1.61 | 2 | 0.81 | 3224.34 | < 0.001 |
T | 2.11 | 3 | 0.7 | 2811.7 | < 0.001 |
D | 0.79 | 3 | 0.26 | 1049.24 | < 0.001 |
SG × T | 0.51 | 6 | 0.08 | 339.78 | < 0.001 |
SG × D | 0.02 | 6 | 0 | 12.31 | < 0.001 |
T × D | 0.02 | 9 | 0 | 8.2 | < 0.001 |
SG × T × D | 0.02 | 18 | 0 | 3.92 | < 0.001 |
The effects of significant interactions of slope gradient with traffic intensity and soil profile depth on BD
are shown in Fig. 2. Average BD
increased with increasing traffic intensity, regardless of slope gradient, but throughout the profile BD
increase was particularly strong in plots with fewer than 5 passes on the 0-10% uphill and 10-20% downhill forwarding slope compared with control plots. The highest average BD
values were observed on the 0-10% uphill forwarding slope with >10 machine passes (Tab. 1), which also experienced the greatest amount of relative change (Fig. 3). Further, average BD
increased with increasing soil profile depth in each slope gradient class (Tab. 1), but relative changes were greatest in the upper 10 cm than of the soil profile (Fig. 2). The highest average BD
values were observed on the 0-10% uphill forwarding slope at a soil depth of 15-20 cm (Tab. 1).
Fig. 2 - Change in BD
(%) after forwarding by slope gradient class, traffic intensity class and soil profile depth class. Changes are relative to measurements from the same profile depth class in untrafficked control plots.
Fig. 3 - Change in PR
(%) after forwarding by slope gradient class, traffic intensity class and soil profile depth class. Changes are relative to measurements from the same profile depth class in untrafficked control plots.
Penetration resistance
Slope gradient (SG), traffic intensity (T) and soil profile depth (D), as well as the interaction effects of SG×T, SG×D, SG×D and SG×T×D significantly affected PR
(Tab. 3). Average PR
by slope gradient was 1525 kPa on the 0-10% downhill, 1705 kPa on the 10-20% downhill and 1834 kPa on the 0-10% uphill forwarding slope. Average PR
increased significantly with increasing the frequency of machine passes and reached the highest level of 1922 kPa after 10 passes. Average PR
also increased significantly with increasing soil profile depth.
Tab. 3 - Analysis of variance (ANOVA) for the effects of slope gradient (SG), traffic intensity (T), soil profile depth (D) classes and their interactions on PR
after forwarding with a farm tractor.
Source | Sum of Squares |
df | Mean Square |
F-value | P-value |
---|---|---|---|---|---|
SG | 2321852 | 2 | 1160926 | 860.29 | < 0.001 |
T | 5643747 | 3 | 1881249 | 1394.08 | < 0.001 |
D | 134033.1 | 3 | 44677.71 | 33.11 | < 0.001 |
SG × T | 1286932 | 6 | 214488.7 | 158.95 | < 0.001 |
SG × D | 29403.57 | 6 | 4900.59 | 3.63 | < 0.001 |
T × D | 38066.81 | 9 | 4229.65 | 3.13 | < 0.001 |
SG × T × D | 110708.2 | 18 | 6150.45 | 4.56 | < 0.001 |
Average PR
increased significantly with increasing traffic intensity, regardless of slope gradient, with particularly strong PR
increases on the 10-20% downhill and the 0-10% uphill forwarding slope compared to control plots; the increase in PR
on the trafficked 0-10% downhill forwarding slope was lower at all traffic intensities and soil depths (Fig. 3). Compared to undisturbed control areas, which had PR
values of 1386 kPa on the 0-10% downhill, 1408 kPa on the 10-20% downhill and 1384 kPa on the 0-10% uphill forwarding slope, average PR
values after >10 machine passes increased to 1656 kPa on the 0-10% downhill, 1927 kPa on the 10-20% downhill and 2185 kPa on the 0-10% uphill forwarding slope. Soil penetration resistance continually increased with traffic frequency in each soil depth and was still increasing at >10 passes, particularly on the 0-10% uphill and 10-20% downhill forwarding slope (Fig. 3). Although the highest average PR
values were found on the 0-10% uphill forwarding slope at a soil depth of 10-20 cm, PR
did not increase significantly with soil depth in any of the slope gradients.
Total porosity
Slope gradient (SG), traffic intensity (T) and soil profile depth (D) as well as the interaction of SG×T significantly affected TP
(Tab. 4). Average TP
by slope gradient was 47.8% on the 0-10% downhill, 42.8% on the 10-20% downhill and 36.9% on the 0-10% uphill forwarding slope (Fig. 4). Average TP
decreased significantly with increasing the traffic intensity and reached the lowest level of 38.6% after 10 passes. However, differences in TP
were small among trafficked plots. TP
also decreased significantly with increasing soil profile depth (Tab. 1).
Tab. 4 - Analysis of variance (ANOVA) for the effects of slope gradient (SG), traffic intensity (T), soil profile depth (D) classes and their interactions on TP
after forwarding with a farm tractor.
Source | Sum of Squares |
df | Mean Square |
F-value | P-value |
---|---|---|---|---|---|
SG | 2873.63 | 2 | 1436.81 | 126.25 | < 0.001 |
T | 3955.4 | 3 | 1318.47 | 115.85 | < 0.001 |
D | 1443.86 | 3 | 481.29 | 42.29 | < 0.001 |
SG × T | 1013.74 | 6 | 168.96 | 14.85 | < 0.001 |
SG × D | 61.63 | 6 | 10.27 | 0.9 | 0.50 |
T × D | 128.83 | 9 | 14.31 | 1.26 | 0.27 |
SG × T × D | 281.26 | 18 | 15.63 | 1.37 | 0.16 |
Fig. 4 - Change in TP
(%) after forwarding by slope gradient class, traffic intensity class and soil profile depth class. Changes are relative to measurements from the same profile depth class in untrafficked control plots.
Average TP
was highest in control plots and decreased with increasing traffic intensity, regardless of slope gradient (Tab. 1). Particularly strong TP
decreases were observed in plots with fewer than 5 passes on the 0-10% uphill and 10-20% downhill forwarding slope as compared to control plots. Generally, only small additional decreases in TP
were observed with additional passes (Fig. 4). The lowest average TP
values were observed on the 0-10% uphill forwarding slope after the first 5 passes (29.9%), where TP
was 17.5% lower compared to the controls (Tab. 1).
Discussion
In this study, the investigated physical soil properties exhibited consistent and largely predictable responses to different tractor traffic intensities throughout the upper 20 cm of the soil profile. The significant impact of traffic intensity on BD
, PR
and TP
on all slope gradients is consistent with findings from previous studies that have documented a marked increase of bulk density, soil strength and loss of macropores with increasing traffic intensity ([38], [16], [12], [40], [4], [31]). The large initial increase in compaction with fewer than 5 machine passes and the modest increase thereafter reflects the typical logarithmic relationship between the degree of soil compaction and the traffic intensity, where the greatest impacts occur during the first three passes and traffic intensities beyond 10 passes contribute very little to further compaction ([18], [16], [12], [13], [20], [22], [7], [4], [31]). One explanation for this logarithmic relationship is that the bearing capacity of the soil (maximum load without soil structure failure) grows with increasing BD
, protecting the surface layer against further compaction with increasing traffic ([7], [4], [31]). The increase in PR
beyond 10 passes observed in this study is in agreement with findings that PR
does not taper off after a few passes but continues to increase with traffic frequencies well beyond the traffic intensity seen in this study ([46]). PR
is a more sensitive measure of logging traffic intensity than bulk density, because particle rearrangement after soil disturbance can increase PR
but not bulk density ([15]) and greater increases in PR
than bulk density are commonly found for the same logging intensity ([2], [8]). The maximum PR
increase of up to 60% observed in this study is similar to the 50% increase reported by Picchio et al. ([31]), but maximum PR
values of 2.3 MPa did not reach levels where root growth of trees becomes restricted (e.g., 2.5-3 MPa; [18], [7]).
The reduction in TP
of up to 45% in this study is similar to that reported by previous analyses, and is likely due to a loss or substantial decrease (50-60%) of macropores in favor of smaller pores after the first few machine passes ([4], [31]). In the soil compaction process, soil pores are typically compressed or destroyed, surface aggregates are broken down, and macropores are transformed into meso- (0.2<d<50 µm) and micropores (d<0.2 µm). As substantial destruction of macropores can already occur after the first few passes, this would explain why additional machine traffic only minimally decreased TP
beyond the initial reduction.
Even though the tendency of increased BD
and PR
and decreased TP
with increased traffic intensity was observed in all slope classes, the observed changes were highest when the forwarding direction was uphill (even on moderate slopes and after just a few passes) or on steeper slopes when the forwarding direction was downhill. Differences in levels of compaction between uphill and downhill forwarding may be explained by an uneven load distribution between the downhill and uphill tires of the skidder ([22]) or increased wheel slippage and vibration in uphill compared to downhill forwarding. Because stresses applied at the soil surface are always transmitted three-dimensionally, they cause not only soil compaction but also shear effects ([20]) and exposure of mineral subsoil, which naturally has a higher BD
and PR
than the surface layer. Further, slope gradient has been found to be an important factor for the potential level of compaction ([38]), presumably because machines can slip continuously and remain longer in a given spot in steeper terrain, thus puddling and dragging the soil ([16]).
An increase in BD
and PR
and a decrease in TP
with soil depth, as observed in control plots on all slope classes in this study, is typical for soil profiles. In untrafficked forest soils, BD
and PR
are typically low and TP
is high in the surface horizons due to large amounts of organic matter; as the organic matter content decreases with soil depth, BD
and PR
increase and TP
decreases ([17], [13]). Further, biological activity of roots and animals can reduce BD
and PR
and increase TP
in the upper soil, while fine soil texture, gravel content and soil structure may increase BD
and PR
and lower TP
at lower soil depths ([18], [4], [31]). The pore volume of a medium- to fine-textured soil consists mainly of meso- and micropores that easily hold water against gravitational forces. The smaller the pore diameter, the more the adhesion of water molecules to soil colloids exceeds the force of gravitational capillarity. So in a saturated state, all pores are filled with water that cannot be compressed. Soil moisture content determines the proportion between soil compaction and plastic deformation (in the form of rutting) after the application of machine forces ([3]). Tractor traffic with its associated wheel or track slip can directly affect the soil structure and alter physical soil properties down to deeper depths. In this study, increased soil compaction occurred throughout the soil profile with associated increases in BD
and PR
and reduction of TP
, which has also been observed previously ([18], [38], [16], [25], [30], [5], [13]). However, relative to values from different soil depths in the control plots, changes in BD
, PR
and TP
following machine traffic were often (but not always) highest in the upper 5 cm layer and lowest in the 20 cm layer of the soil profile. Compaction effects are generally most distinct in the upper soil layer, since the exerted pressure is maximal at the soil surface and declines with increasing depth as the total pressure is spread out over a growing area ([18], [4], [31]). As soils are compacted and lose large pores after a few passes, compaction leads to higher soil strength in the upper soil layers, which prevents much further compaction of the surface layer with additional passes and shifts forces to deeper soil layers that are then increasingly compacted ([4], [31]). Overall, damage to the soil (including deeper soil layers) was modest in this study, which might be bacause the intensity of machine traffic was generally at the low end compared to other studies (e.g., [46]) and the very dry soil conditions on this site at the time of the forwarding operation were better able to resist to the mechanical soil compaction and rutting (e.g., [43], [7]).
Conclusion
This study clearly demonstrates that changes in physical soil properties in response to farm tractor traffic are significantly affected by slope gradient and forwarding direction (i.e., uphill vs. downhill forwarding). Uphill forwarding, even on gentle slopes below 10% inclination, consistently caused more soil damage throughout the upper 20 cm of the soil profile than downhill forwarding on even steeper slopes. The pattern of the largest negative impact for uphill forwarding was the same for all three soil response variables, bulk density, resistance to penetration and total porosity, and at all traffic intensities along the skid trail. As a consequence, we recommend that skid trails have to be designed to avoid uphill forwarding by this farm tractor-2-wheel-trailer system. If possible, the application of this forwarding system should be limited to gentle slopes and alternative means of forwarding should be sought on steeper slopes. Given that impacts would have been even more severe had soils not been dry during the forwarding operation, logging operations should be restricted to periods when soil conditions are dry in order to minimize compaction and rutting. Future designs of skid trails should not only take into account the gradient of the slope of a skid trail, but also the direction of forwarding used by the chosen harvesting system.
Acknowledgments
The authors acknowledge the financial support by the University of Tehran for this research under grant number 28514/1/3.
References
Gscholar
Gscholar
Gscholar
Gscholar
Gscholar
Gscholar
Gscholar
Gscholar
Gscholar
Gscholar
Gscholar
Authors’ Info
Authors’ Affiliation
Shoukuh Soltanpour
Maryam Etehadi Abari
Department of Forestry and Forest Economics, Faculty of Natural Resources, University of Tehran, Zob-e-Ahan Street, 315854314 Karaj (Iran)
College of Agricultural Sciences, Pennstate University, 305 Forest Resources Building, University Park, 16802 PA (United States of America)
Corresponding author
Paper Info
Citation
Jourgholami M, Soltanpour S, Etehadi Abari M, Zenner EK (2014). Influence of slope on physical soil disturbance due to farm tractor forwarding in a Hyrcanian forest of northern Iran. iForest 7: 342-348. - doi: 10.3832/ifor1141-007
Academic Editor
Enrico Marchi
Paper history
Received: Oct 06, 2013
Accepted: Nov 25, 2013
First online: Apr 17, 2014
Publication Date: Oct 01, 2014
Publication Time: 4.77 months
Copyright Information
© SISEF - The Italian Society of Silviculture and Forest Ecology 2014
Open Access
This article is distributed under the terms of the Creative Commons Attribution-Non Commercial 4.0 International (https://creativecommons.org/licenses/by-nc/4.0/), which permits unrestricted use, distribution, and reproduction in any medium, provided you give appropriate credit to the original author(s) and the source, provide a link to the Creative Commons license, and indicate if changes were made.
Web Metrics
Breakdown by View Type
Article Usage
Total Article Views: 49791
(from publication date up to now)
Breakdown by View Type
HTML Page Views: 41516
Abstract Page Views: 3389
PDF Downloads: 3724
Citation/Reference Downloads: 12
XML Downloads: 1150
Web Metrics
Days since publication: 3871
Overall contacts: 49791
Avg. contacts per week: 90.04
Article Citations
Article citations are based on data periodically collected from the Clarivate Web of Science web site
(last update: Feb 2023)
Total number of cites (since 2014): 29
Average cites per year: 2.90
Publication Metrics
by Dimensions ©
Articles citing this article
List of the papers citing this article based on CrossRef Cited-by.
Related Contents
iForest Similar Articles
Research Articles
Impact of wheeled and tracked tractors on soil physical properties in a mixed conifer stand
vol. 9, pp. 89-94 (online: 22 May 2015)
Research Articles
Effects of soil compaction on seedling morphology, growth, and architecture of chestnut-leaved oak (Quercus castaneifolia)
vol. 10, pp. 145-153 (online: 13 June 2016)
Research Articles
Estimating machine impact on strip roads via close-range photogrammetry and soil parameters: a case study in central Italy
vol. 11, pp. 148-154 (online: 07 February 2018)
Research Articles
Effects on soil physicochemical properties and seedling growth in mixed high forests caused by cable skidder traffic
vol. 16, pp. 127-135 (online: 23 April 2023)
Research Articles
Use of terrestrial laser scanning to evaluate the spatial distribution of soil disturbance by skidding operations
vol. 8, pp. 386-393 (online: 08 October 2014)
Research Articles
Effect of forwarder multipassing on forest soil parameters changes
vol. 15, pp. 476-483 (online: 24 November 2022)
Research Articles
Comparison of alternative harvesting systems for selective thinning in a Mediterranean pine afforestation (Pinus halepensis Mill.) for bioenergy use
vol. 14, pp. 465-472 (online: 16 October 2021)
Research Articles
Exploring the reliability of CAN-bus data in assessing forwarder rolling resistance under real working conditions
vol. 17, pp. 360-369 (online: 13 November 2024)
Research Articles
Deploying an early-stage Cyber-Physical System for the implementation of Forestry 4.0 in a New Zealand timber harvesting context
vol. 17, pp. 353-359 (online: 13 November 2024)
Research Articles
Density, extractives and decay resistance variabilities within branch wood from four agroforestry hardwood species
vol. 14, pp. 212-220 (online: 02 May 2021)
iForest Database Search
Search By Author
Search By Keyword
Google Scholar Search
Citing Articles
Search By Author
Search By Keywords
PubMed Search
Search By Author
Search By Keyword