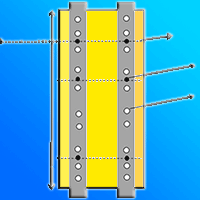
Effects on soil physicochemical properties and seedling growth in mixed high forests caused by cable skidder traffic
iForest - Biogeosciences and Forestry, Volume 16, Issue 2, Pages 127-135 (2023)
doi: https://doi.org/10.3832/ifor4103-016
Published: Apr 23, 2023 - Copyright © 2023 SISEF
Research Articles
Abstract
The use of wheel skidders for timber extraction from tree stump to roadside landing has become more and more widespread. Although the use of wheel skidders has the advantages of high production and reduced extraction costs, it also damages the soil and impedes forest regeneration. The main purpose of this study was to investigate the effect of machine traffic using the Timberjack 450C (two, six and 15 passes) on two slope classes (SC) of skid trails. A low slope is considered to be <20% and a high slope is at >20%. The effects on soil physicochemical properties and seedling growth (alder, Alnus subcordata C.A. Mey. and maple, Acer velutinum Boiss.) in natural mixed beech stands in the Hyrcanian forests in Northern Iran were observed and studied. The results showed that the different factors of traffic intensity (TI) and SC had a significant impact on soil physicochemical properties and subsequent seedling growth. After two machine passes in a low TI on both low and high slopes, soil bulk density (BD) increased by 49.3% and 59.2% and penetration resistance increased by 30.5% and 38.5%, while total porosity decreased by 19.5% and 23.5%. The forest floor decreased by 30.9% and 42%, organic carbon decreased by 25.6% and 39.4%, nitrogen decreased by 18.5% and 26.3%, phosphorus decreased by 14.1% and 23%, and potassium decreased by 10.7% and 24.2%, respectively as compared with the control area. Our results indicated additional BD increments after two, six and 15 machine passes of 49.3%, 17.9% and 8.3% in the low slope, respectively, and 59.2%, 16.5% and 7.1% in the high slope, respectively. The mean of the germination rate (GR) of alder and maple seedlings in the control area was 58.3% and 46.1%, respectively, while after two, six and 15 passes, the GR of alder seedlings reduced to 50%, 46.4% and 37.5%, respectively, while that of maple seedlings reduced to 36.1%, 28.6% and 25.6%, respectively. Additionally, after two machine passes, stem length, main root length, and total dry biomass decreased by 28.7%, 34.9% and 34% in alder seedlings, respectively, and 27.9%, 27.6% and 33.3% in maple seedlings, respectively. Comparison of the response of the two seedling species to soil compaction showed that although alder had a higher GR than maple, the root growth of maple was higher than that of alder.
Keywords
Forest Regeneration, Soil Compaction, Trail Gradient, Wheel Skidder
Introduction
Forest soils are sensitive entities of high chemical, physical and biological complexity that sustain the productivity of forest ecosystems ([40]). Forest soils have fundamental environmental functions: they provide nutrients and water for plant growth, play an important role in carbon stocking and the water cycle. Therefore, soil preservation is a critical issue that is gaining attention with respect to global environmental policy ([4]). Undisturbed forest soils have high macroporosity (MP) and low soil bulk density (BD) and are easily compacted by logging machinery ([36], [53], [33]). The use of heavy machinery in forest operations may seriously affect the soil ecosystem by inducing rutting, compaction and topsoil mixing, especially within the upper layers ([1]). Soil disturbances are unavoidable consequences of forest logging, especially ground-based skidding, and their severity, extent, and distribution depend on the interaction among forest site characteristics at the time of harvest ([45], [22]), silvicultural treatment ([47]), forest logging methods and quality ([21], [28]) and machine characteristics ([47]). Moreover, the magnitude of soil compaction depends on several factors, such as traffic intensity (TI), slope gradient, site characteristics, harvesting machine types and characteristics, planning of skid trails, and harvesting season ([15], [11]).
The functionality of forest soils depends on a structure characterised by pore systems that simultaneously provide water and air to tree roots and soil organisms ([40]). Compaction and rutting may have negative physical, chemical, and biological effects on forest soil. Machinery-induced soil compaction greatly reduces porosity and pore connectivity and increases soil density, soil shear resistance, and soil penetration resistance (PR - [17], [39], [31], [55], [30], [37], [14]). Reduction of coarse porosity and loss of pore continuity negatively affect soil aeration ([20], [18], [23], [2]), water infiltration, and soil water balance and may therefore have a significant impact on soil organisms and forest growth and productivity ([31], [54], [7]). Dedecek & Gava ([9]) analysed the influence of soil compaction on eucalyptus plantations and highlighted that soil compaction decreased stand productivity by two-thirds. On the other hand, high yields occur in soil with high MP and low PR ([41]).
Maintaining the growth and quality of natural seedlings is an important goal of forest management in uneven-aged high stands ([53]). Compaction reduces the penetrability of the soil by roots ([6], [53]). When PR increases to more than 2.00-2.50 MPa, the root growth of many trees is limited ([19]). Previous studies have revealed that root elongation and branching patterns can be negatively influenced by increasing soil BD ([6], [35], [7], [34], [53]). For example, Tavankar et al. ([53]) studied the response of beech seedlings to soil compaction and reported that the ratio of main root to stem length (RRS) and the ratio of root penetration to main root length (RPL) were significantly higher in the uncompacted area (control) than on skid trails. Jourgholami ([27]) demonstrated that the root mass to shoot mass ratio (R/S) of maple seedlings was highest in the undisturbed (control) area and lowest on skid trails with high TI.
Compaction and rutting can also negatively affect seedling establishment and growth with regard to both root and shoot variables ([44], [8], [28], [45]). Cambi et al. ([7]) concluded that the shoot/root ratio and main root length (MRL) of Quercus robur L. seedlings decreased as soil BD increased. Furthermore, Jourgholami et al. ([26]) reported that soil compaction adversely impacted the size and biomass of Quercus castaneifolia seedlings. Blouin et al. ([5]) also stated that growth parameters of lodgepole pine (Pinus contorta Dougl. Ex. Loud. var. latifolia Engelm.) seedling such as new root mass and stem basal diameter decreased as BD increased.
A review of the literature shows that the impact of skidding operations on the physical and chemical properties of forest soils and their effects on the subsequent seedling growth in pioneer tree species (alder and maple) of the Hyrcanian forests have not been well studied. The main aims of this research were to evaluate: (i) the effect of machine traffic on the physical and chemical properties of soil; (ii) the effect of soil compaction on the growth, architecture and quality of seedlings; and (iii) the relationship between seedling growth characteristics and soil properties.
Materials and methods
Study area
The study was conducted in the Hyrcanian forests in northern Iran. The study site is located in Sorkhekolah forest, Mazandaran province (latitude: 36° 21′ to 36° 25′ N; longitude: 53° 50′ to 53° 60′ E). The elevation of the study site is approximately 1520 m a.s.l., and it is oriented towards the north. The mean annual precipitation is approximately 1300 mm and the mean annual temperature is 15 °C. The forest type comprises uneven-aged mixed hardwood species dominated by beech (Fagus orientalis Lipsky) and hornbeam (Carpinus betulus L.). Other accompanying tree species include Caucasian alder (Alnus subcordata C.A. Mey.), maple (Acer velutinum Boiss.), Cappadocian maple (Acer cappadocicum Gled.), Norway maple (Acer platanoides L.), velvet maple (Acer insigne Boiss.), wych elm (Ulmus glabra Huds.), lime tree (Tilia begonifolia Stev.), Caucasian elm (Zelkova caprinifolia Diopp), chestnut-leaved oak (Quercus castaneifolia Gled.) and common ash (Fraxinus excelsior L.). The canopy cover was 80%, stand density was 220 trees ha-1, average diameter at breast height (DBH, 1.3 m above the ground) was 34 cm and average tree height was 22.3 m before the harvesting operation ([43]).
A combination of the group selection and single-tree selection silvicultural systems was applied in the study area, and the harvesting intensity was 9.5 m3 ha-1. Harvesting operations were performed by motor-manual felling and processing, followed by timber extraction from the forest stand to the roadside using a rubber-tired “Timberjack 450C” cable skidder. The density of the skid trail in the study area was 25.2 m ha-1. The weight of the skidder was 9.8 Mg (55% on the front and 45% on the rear axle), and its width and length were 3.8 m and 6.4 m, respectively. The skidder had an engine power of 177 hp (132 kW). It was equipped with a blade for lightly pushing obstacles and stacking logs. The skidder was fitted with size 24.5-32.0 tires inflated to 220 kPa on both front and rear axles, no chains or tracks were installed on the skidder during skidding.
At the time of skidding operations (March 2015), weather conditions were wet, with an average gravimetric soil moisture content of 28%. The soil texture was analysed using the Bouyoucos hydrometer method to a depth of 10 cm and was determined to be clay, with a particle size distribution of 53 ± 4.5% clay (< 0.002 mm), 25.5 ± 2.9% silt (0.002-0.05 mm) and 21.5 ± 2.5% sand (0.05-2 mm) along the machine operating trail. The soil had not been driven on before the experiment. The rubber-tired cable skidder was used to extract logs 3-4 m in length on trafficable terrain of a gradient of up to 30%. The skidder was loaded to the maximum load capacity (2.5 to 3.0 m3) in all experiments.
Data collection
The impacts of skidding operations at different trail gradients and traffic frequencies on the soil surface layer (0-10 cm depth) of the skid trail were quantified using dry BD, total porosity (TP) and litter mass (LM) and then compared to undisturbed control (UC) areas within the same forest stand. In addition, the influence of skidder traffic frequency and trail gradient on the germination rate (GR) and stem and root growth of alder (Alnus subcordata C.A. Mey.) and maple (Acer velutinum Boiss.) seedlings was also assessed. Alder and maple seedlings were chosen because these pioneer species have shade-intolerant seeds, and they are suitable for restoring compacted soils as well as being the preferred species for revegetating machine operating trails and landings in Iran ([44], [25], [45]).
Two skid trails with a range of longitudinal gradients and without any lateral gradient were selected for study, one for planting alder seeds (SKT-A) and one for planting maple seeds (SKT-M). Two longitudinal gradient classes were considered on each skid trail, i.e., < 20% (low slope, LS) and ≥ 20% (high slope, HS). The LS class contained trail segments with gradients ranging between 4% and 15%, whereas the HS class contained segments with gradients ranging between 23% and 30%. Three traffic frequencies (TIs) of the loaded skidder were considered for each slope class (SC) of the skid trail: (i) two passes for light traffic (2P); (ii) six passes for moderate traffic (6P); and (iii) 15 passes for heavy traffic segments (15P). Thus, treatment plots (PLs) included the combination of two levels of trail gradient (LS and HS) and three levels of traffic frequency (2P, 6P and 15P), resulting in six combinations of traffic frequency and trail gradient (LS2P, LS6P, LS15P, HS2P, HS6P and HS15P). Each treatment combination was replicated three times, totaling 18 PLs in each trail.
The PLs were 10 m long and 4 m wide (area of 40 m2 - Fig. 1), with a separation of 5 m between plots (PLs). They included three 4 m linear transects, which randomly placed across the wheel track perpendicular to the direction of travel, with a buffer zone of at least 2 m between transects ([50]). Forest floor (FF) samples, defined as the O layer from the litter surface to the mineral soil, were also collected over a 1 m2 subplot (OP) from two locations on each transect (PL), i.e., the left and right wheel track (LWT and RWT). Once the FF was removed, soil samples were collected from a depth of 0-10 cm (excluding the recently removed FF) at two different locations on each transect, i.e., the centre of the LWT and RWT. Thus, six soil core samples and six FF samples were collected from each of the 18 PLs. Additionally, one soil sample and one FF sample were collected from either side of the PLs at a distance of 50 m from the PL centre to constitute the control plots (CPs, zero passes). There was no direct skidding impact on the CPs. The soil was cleaned of FF material, sampled with a soil hammer and rings (diameter 5 cm, length 10 cm), placed in polyethylene bags, labelled and transported to the laboratory. On each plot, the soil PR was measured near the soil sampling points at six points on the PLs (three points each on the LWT and RWT) and six points on the CPs (three points each on the left and right CP) using a hand-held penetrometer (Eijkelkamp, Zevenaar, Netherlands) at depths of 0, 5 and 10 cm ([53]).
Fig. 1 - Sampling design for soil and seedlings, detail of a treatment plot (PL). On the left side of the figure at a distance of 50 m from the PL centre is possible to note the control plots (CPs, 0 passes).
Freshly matured alder and maple seeds were collected during the 2014 growing season from an uneven-aged stand inside the study area. In each PL (18 PLS) of the SKT-A, twenty alder seeds (10 seeds each on the LWT and RWT) were planted. Two seeds were planted on the CPs of each PL, i.e., 36 seeds. The same number of seeds were planted on the SKT-M, i.e., 20 maple seeds (10 seeds each on the LWT and RWT) in each PL and two seeds on the CP of each PL. All the seeds were planted at a depth of 2 cm. The distance between the planted seeds was 0.6 m. The study area was fenced to prevent animal browsing. The treatments included weed control during the research period after seeding to eliminate the confounding effects of soil compaction on vegetation communities and competition ([13]).
Soil physical properties
The soil core samples and FF collected in the field were brought to the laboratory, oven-dried at 105 °C (24 h) for the soil and 65 °C (48 h) for the floor, respectively, and weighted to determine soil BD (i.e., the mass of dry soil per unit volume of soil core, g cm-3), soil porosity, soil moisture content (i.e., the amount of water associated with a given volume or mass of soil), and the dry weight of the FF.
Total soil porosity (TP, %) was calculated as (eqn. 1):
where BD is the dry bulk density (g cm-3) and 2.65 g cm-3 is the particle density measured by a pycnometer on the same soil samples used to determine the BD ([46]). Microporosity (MIP, %) was calculated as (eqn. 2):
where θm is the mass water content (%). Finally, macroporosity (MP, %) was calculated as follows (eqn. 3):
Soil chemical properties
To determine the chemical properties of the soil, soil samples were air-dried and only particles finer than 2 mm were used for analysis. Particle size distribution was determined using the Bouyoucos hydrometer method ([29]), while soil acidity was measured using a pH meter with glass electrodes in 1/2.5 distilled water. The percentage of organic carbon (OC) was determined following the Walkley-Black procedure, nitrogen (N) was evaluated using the semi-micro-Kjeldahl method, phosphorus (P) was determined using a spectrophotometer, and potassium (K) was determined following the ammonium acetate method using a flame photometer ([46]).
Seedling survival and growth data collection
In early October 2015, all seedlings were assessed for germination, and morphological, architectural, and growth parameters were collected. The following response variables were determined at the end of the study (180 days after seeding in late March 2015): (i) morphological parameters, such as germination rate (GR), seedling height (SH), stem length (SL), stem diameter (SD), main root length (MRL), main root diameter (MRD), lateral root length (LRL) and root penetration depth (RPD); (ii) growth parameters, such as total dry biomass (TDB), stem dry biomass (SDB) and root dry biomass (RDB); (iii) architectural parameters, such as ratio of lateral to main root length (RLM), root mass ratio (RMR, ratio of RDB to TDB), stem mass ratio (SMR, ratio of SDB to TDB), RRS and RPL.
Stem and root diameter were measured 5 cm above and below the soil surface, respectively, using a Vernier calliper (Insize Model 1205, Austria). The vertical distance from the tip of the root to the soil surface was taken as the RPD and measured using a metal ruler. Dry weights (biomass) of seedlings were obtained after drying at 70 °C until a constant weight was reached. Seedling quality index (SQI) was calculated per Dickson et al. ([12] - eqn. 4):
Statistical analysis
Data analyses were performed using SPSS® version 19 (Chicago, IL, USA) software. The normality of data distribution was checked by the Kolmogorov-Smirnov test (α = 0.05). The homogeneity of variance among treatments was tested using the Levene’s test (α = 0.01). The two-way analysis of variance (ANOVA) and post-hoc Tukey’s HSD test were used to compare the mean values of soil properties and seedling characteristics at various skidder traffic levels and trail gradients as well as their interactions at p ≤ 0.05. The independent samples t-test was applied for the comparison of mean values of soil properties and seedling characteristics between SCs. The relationships between soil properties and seedling characteristics were determined using Pearson’s correlation.
Results
Soil physical properties
The results of the ANOVA showed that: (i) the intensity of machine traffic (TI) had a significant effect on both physical and chemical properties of the soil; (ii) the SC of the skid trail had a significant effect on both physical and chemical (except pH) properties of the soil; and (iii) the interaction of TI and SC of the skid trail (TI × SC) had a significant effect only on soil MIP and N (see Tab. S1 in Supplementary material for more details).
According to Duncan’s test, the mean values of soil BD, PR and pH significantly increased with the increase of the number of machine passes in both LS and HS SCs (Tab. 1). The mean values of TP, MP, MC, FF, OC, N, P and K significantly decreased as the number of machine passes increased in both the LS and HS classes of skid trails, with the exception of HS for TP and LS for MP, which did not show any significant difference between six and 15 passes.
Tab. 1 - Effect of machine traffic and slope on soil physical (mean ± standard deviation). Zero (0) passes refer to undisturbed area (no machine traffic). Different letters within each treatment indicate significant differences between machine traffic intensity and uncompacted area by Duncan’s test at α = 0.05. (*): significant differences between slope classes by independent samples t-test at α = 0.05. (LS): low slope; (HS): high slope. Physical properties: (BD) bulk density; (TP) total porosity; (MP) macroporosity; (MIP) microporosity; (PR) penetration resistance; (MC) moisture content. Chemical properties: (FF) forest floor; (OC) organic carbon; (N) nitrogen; (P) phosphorous; (K) potassium; (pH) acidity.
Soil physicochemical properties | Slope class | Traffic intensity (no. passes) | |||
---|---|---|---|---|---|
0 | 2 | 6 | 15 | ||
BD (g cm-3) | LS | 0.75 ± 0.05 d | 1.12 ± 0.04 c | 1.32 ± 0.06 b | 1.43 ± 0.05 a |
HS | 0.76 ± 0.04 d | 1.21 ± 0.05* c | 1.41 ± 0.05* b | 1.51 ± 0.05* a | |
TP (%) | LS | 71.7 ± 3.50 a | 57.7 ± 2.36 b | 50.2 ± 2.10 c | 46.0 ± 1.96 d |
HS | 71.0 ± 3.50 a | 54.3 ± 2.36* b | 46.8 ± 2.57* c | 43.0 ± 1.70 c | |
MP (%) | LS | 43.1 ± 1.17 a | 35.2 ± 2.25 b | 31.4 ± 2.05 c | 30.1 ± 1.85 c |
HS | 42.8 ± 1.05 a | 30.7 ± 2.25* b | 24.3 ± 2.02* c | 20.9 ± 1.73* d | |
MIP (%) | LS | 24.5 ± 1.10 b | 32.4 ± 1.05 a | 31.6 ± 1.00 a | 32.1 ± 1.02 a |
HS | 24.0 ± 1.12 b | 33.2 ± 1.04 a | 32.5 ± 1.02* a | 32.2 ± 1.01* a | |
PR (MPa) | LS | 1.05 ± 0.06 d | 1.37 ± 0.09 c | 1.45 ± 0.08 b | 1.58 ± 0.09 a |
HS | 1.08 ± 0.05 d | 1.50 ± 0.08* c | 1.56 ± 0.06* b | 1.68 ± 0.05* a | |
MC (%) | LS | 48.2 ± 4.27 a | 36.5 ± 3.30 b | 30.4 ± 3.05 c | 25.5 ± 2.83 d |
HS | 48.0 ± 4.35 a | 32.5 ± 2.41* b | 23.1 ± 2.64* c | 18.2 ± 2.43* d | |
FF (kg ha-1) | LS | 3645 ± 205 a | 2518 ± 191 b | 1426 ± 124 c | 907 ± 76 d |
HS | 3218 ± 183* a | 1855 ± 146* b | 1044 ± 108* c | 427 ± 59* d | |
OC (%) | LS | 6.55 ± 0.20 a | 4.87 ± 0.21 b | 3.95 ± 0.20 c | 3.05 ± 0.16 d |
HS | 6.47 ± 0.19 a | 3.92 ± 0.17* b | 3.03 ± 0.14* c | 2.41 ± 0.15* d | |
N (%) | LS | 0.276 ± 0.02 a | 0.225 ± 0.02 b | 0.160 ± 0.01 c | 0.091 ± 0.01 d |
HS | 0.274 ± 0.02 a | 0.202 ± 0.02* b | 0.108 ± 0.01* c | 0.052 ± 0.01* d | |
P (ppm) | LS | 41.2 ± 2.8 a | 35.4 ± 2.6 b | 30.0 ± 1.6 c | 26.1 ± 1.3 d |
HS | 40.4 ± 2.5 a | 31.1 ± 2.1* b | 25.2 ± 1.5* c | 21.2 ± 1.5* d | |
K (ppm) | LS | 12.1 ± 0.52 a | 10.8 ± 0.50 b | 9.0 ± 0.28 c | 6.0 ± 0.22 d |
HS | 12.0 ± 0.52 a | 9.1 ± 0.31* b | 7.2 ± 0.3* c | 4.3 ± 0.14* d | |
pH (%) | LS | 5.15 ± 0.16 d | 6.31 ± 0.21 c | 7.40 ± 0.32 b | 7.96 ± 0.30 a |
HS | 5.45 ± 0.14 d | 6.94 ± 0.30* c | 7.84 ± 0.30* b | 8.35 ± 0.28* a |
The mean values of MIP were significantly lower in the CPs than in the trails but no significant differences were recorded among the TIs.
The results of the t-tests indicated that in the CP there were no significant differences between the LS and HS classes in any of the soil physicochemical properties. After two passes, only MIP was not significantly different between LS and HS. After six passes, the mean values of all of the soil physicochemical properties were significantly different between LS and HS. After 15 passes, only TP was not significantly different between LS and HS (Tab. 1).
Pearson’s correlation analysis indicated a significant negative relationship of BD with most soil physicochemical properties and a significant positive relationship of BD with MIP, PR, and pH. TP showed a significant positive correlation with MP, MC, FF, OC, N, P and K and a significant negative relationship with BD, MIP, and PR. Additionally, PR showed a significant positive correlation with most soil physicochemical properties with the exception of TP, MP, MIP and pH (Tab. 2).
Tab. 2 - Pearson correlation coefficients (r) of soil physical and chemical properties. (BD): bulk density; (TP): total porosity; (MP): macroporosity; (MIP): microporosity; (PR): penetration resistance; (MC): moisture content; (FF): forest floor; (OC): organic carbon; (N): nitrogen; (P): phosphorous; (K): potassium; (pH): acidity; (*): P<0.01; (*): P<0.05; (NS): not significant.
Variables | TP | MP | MIP | PR | MC | FF | OC | N | P | K | pH |
---|---|---|---|---|---|---|---|---|---|---|---|
BD | -0.996** | -0.954** | 0.544** | 0.874** | -0.750** | -0.845** | -0.905** | -0.879** | -0.899** | -0.884* | -0.758** |
TP | 1 | 0.982** | -0.235 NS | -0.906** | 0.935** | 0.894** | 0.905** | 0.894** | 0.868** | 0.906** | 0.873** |
MP | - | 1 | -0.315 NS | -0.924** | 0.955** | 0.922** | 0.936** | 0.904** | 0.859** | 0.542* | 0.544* |
MIP | - | - | 1 | -0.938** | 0.910** | 0.768** | 0.842** | 0.560* | 0.532* | 0.507* | -0.462* |
PR | - | - | - | 1 | 0.808** | 0.759** | 0.687** | 0.609** | 0.618** | 0.499* | -0.719** |
MC | - | - | - | - | 1 | 0.798** | 0.816** | 0.614** | 0.584* | 0.482* | 0.540* |
FF | - | - | - | - | - | 1 | 0.916** | 0.930** | 0.954** | 0.840** | 0.849** |
OC | - | - | - | - | - | - | 1 | 0.882** | 0.885** | 0.861** | 0.831** |
N | - | - | - | - | - | - | - | 1 | 0.872** | 0.890** | 0.786** |
P | - | - | - | - | - | - | - | - | 1 | 0.909** | 0.817** |
K | - | - | - | - | - | - | - | - | - | 1 | 0.793** |
Seedling response
The results of the ANOVA revealed that: (i) TI had a significant effect on all seedling characteristics; (ii) SC had a significant effect on most seedling characteristics with the exception of all the dry biomass values of alder seedlings; and (iii) the interaction of TI and SC had a significant effect on most seedling characteristics, with the exception of GR and SQI for both alder and maple seedlings (see Tab. S2 in Supplementary material for more details).
The results indicated that GR decreased with increasing TI (Fig. 1). The results of the independent t-tests revealed that the mean GR of alder seedlings was significantly higher than the mean GR of maple seedlings at all TIs.
The results of Duncan’s tests showed that both the morphological (SL, MRL, SD and LRL) and growth (SDB, RDB and TDB) characteristic values of the seedlings significantly decreased with increasing TI (Tab. 3). However, no significant difference was recorded between the control and two passes for alder SD in both SCs and between two and six passes for maple SDB in both SCs. Taking SC into consideration, significant differences between LS and HS were recorded for alder SL after 15 passes, alder MRL after six passes and maple MRL after six and 15 passes (Tab. 3).
Tab. 3 - Effect of machine traffic and slope on the morphology and growth characteristics (mean ± SD). Zero (0) passes refer to undisturbed area, no machine traffic. Different letters within each treatment indicate significant differences between machine traffic intensity and uncompacted area by Duncan’s test at α = 0.05. (*): significant differences between slope classes by independent samples t-test at α = 0.05. (SL): stem length; (MRL): main root length; (SD): stem diameter; (LRL): lateral root length; (SDB): stem dry biomass; (RDB): root dry biomass; (TDB): total dry biomass of alder and maple seedlings.
Seedling characteristics |
Seedling species |
Slope class |
Traffic intensity (no. passes) | |||
---|---|---|---|---|---|---|
0 (n=18) |
2 (n=60) |
6 (n=60) |
15 (n=60) |
|||
SL (cm) | Alder | LS | 13.6 ± 1.3 a | 9.6 ± 1.1 b | 7.5 ± 0.6 c | 5.3 ± 0.5 d |
HS | 12.6 ± 1.5 a | 8.5 ± 1.1 b | 6.7 ± 0.9 c | 4.1 ± 0.5* d | ||
Maple | LS | 19.7 ± 1.6 a | 12.4 ± 1.1 b | 9.8 ± 1.0 c | 6.2 ± 0.6 d | |
HS | 18.1 ± 1.6 a | 11.7 ± 1.0 b | 8.5 ± 0.7 c | 5.5 ± 0.5 d | ||
MRL (cm) | Alder | LS | 18.9 ± 2.4 a | 12.3 ± 1.7 b | 8.6 ± 1.2 c | 4.7 ± 0.5 d |
HS | 17.0 ± 2.1 a | 11.5 ± 1.6 b | 7.1 ± 1.1* c | 3.6 ± 0.5 d | ||
Maple | LS | 29.0 ± 3.3 a | 22.3 ± 2.5 b | 16.0 ± 1.5 c | 10.7 ± 1.0 d | |
HS | 27.6 ± 3.2 a | 20.0 ± 2.2 b | 13.3 ± 0.08* c | 7.1 ± 0.8* d | ||
SD (mm) | Alder | LS | 3.3 ± 0.05 a | 3.0 ± 0.04 a | 2.4 ± 0.05 c | 1.8 ± 0.03 d |
HS | 3.0 ± 0.05 a | 2.9 ± 0.03 a | 2.3 ± 0.04 c | 1.7 ± 0.03 d | ||
Maple | LS | 3.5 ± 0.05 a | 3.0 ± 0.04 b | 2.5 ± 0.04 c | 2.0 ± 0.03 d | |
HS | 3.5 ± 0.05 a | 3.0 ± 0.04 b | 2.5 ± 0.04 c | 1.9 ± 0.03 d | ||
LRL (cm) | Alder | LS | 25.5 ± 2.6 a | 17.0 ± 2.1 b | 10.6 ± 1.3 c | 5.5 ± 0.08 d |
HS | 23.4 ± 2.5 a | 16.1 ± 2.0 b | 10.1 ± 1.3 c | 5.4 ± 0.08 d | ||
Maple | LS | 38.1 ± 2.7 a | 27.5 ± 2.4 b | 16.4 ± 2.1 c | 8.6 ± 0.10 d | |
HS | 36.3 ± 2.8 a | 24.0 ± 2.4 b | 16.7 ± 2.1 c | 8.3 ± 0.10 d | ||
SDB (g) | Alder | LS | 4.7 ± 0.05 a | 3.9 ± 0.03 b | 3.0 ± 0.04 c | 2.2 ± 0.03 d |
HS | 4.2 ± 0.03 a | 3.5 ± 0.03 b | 2.8 ± 0.04 c | 2.0 ± 0.03 d | ||
Maple | LS | 5.5 ± 0.04 a | 4.4 ± 0.05 b | 3.5 ± 0.03 b | 2.5 ± 0.03 d | |
HS | 5.3 ± 0.03 a | 4.1 ± 0.04 b | 3.2 ± 0.03 b | 2.1 ± 0.03 d | ||
RDB (g) | Alder | LS | 3.1 ± 0.04 a | 2.5 ± 0.05 b | 1.7 ± 0.03 c | 1.2 ± 0.03 d |
HS | 3.0 ± 0.04 a | 2.5 ± 0.05 b | 1.7 ± 0.03 c | 1.1 ± 0.03 d | ||
Maple | LS | 4.2 ± 0.06 a | 2.8 ± 0.04 b | 1.9 ± 0.04 c | 1.3 ± 0.03 d | |
HS | 4.0 ± 0.05 a | 2.7 ± 0.04 b | 1.9 ± 0.04 c | 1.4 ± 0.03 d | ||
TDB (g) | Alder | LS | 7.8 ± 0.06 a | 6.4 ± 0.03 b | 4.7 ± 0.05 c | 3.3 ± 0.04 d |
SH | 7.2 ± 0.06 a | 6.0 ± 0.04 b | 4.5 ± 0.05 c | 3.1 ± 0.03 d | ||
Maple | LS | 9.7 ± 0.05 a | 7.2 ± 0.04 b | 5.4 ± 0.05 c | 3.8 ± 0.04 d | |
HS | 9.3 ± 0.06 a | 7.1 ± 0.04 b | 5.1 ± 0.04 c | 3.5 ± 0.04 d |
The results indicated that the highest reduction rate due to machine traffic compared with the control was in TDB, followed by MRL, SL and GR after 15 passes (Fig. 2). The reduction rates of all growth parameters were higher in alder seedlings than in maple seedlings at all TIs.
Fig. 2 - Effect of traffic intensity and slope gradient on germination rate. (LS): low slope; (HS): high slope.
The results revealed that mean SQI decreased with increasing machine passes and was lower in the HS class than in the LS class (Fig. 3). The results of the t-tests indicated that the mean SQI of maple seedlings was significantly higher than that of alder seedlings in the control (zero passes) and after two passes, while no significant differences were observed after six and 15 passes in both LS and HS classes.
Fig. 3 - Reduction rate (%) in alder and maple seedling characteristics after (a) two, (b) six, and (c) 15 machine passes compared to the control area.
The results showed that the architectural characteristics of the seedlings were affected by machine traffic (Fig. 4). The RLM value of both alder and maple seedlings in the LS class decreased with increasing TI, while in the HS class, the RLM value of alder seedlings increased with increasing TI. The RMR value of both alder and maple seedlings decreased with increasing TI, the SMR value of both alder and maple seedlings increased with increasing TI in the LS class and the RRS value of alder seedlings decreased with increasing TI in the LS class. The RLM values of alder seedlings were higher than those of maple seedlings, whereas the RRS values of maple seedlings were higher than those of alder seedlings (Fig. 4).
Fig. 4 - Effect of traffic intensity and slope gradient on seed quality index. (LS): low slope; (HS): high slope.
The results of the Pearson correlation tests showed that there were significant relationships between seedling growth characteristics and soil physicochemical properties (see Tab. S3 in Supplementary material for detail used to perform this analysis). The highest r value was observed for the association between seedling growth characteristics and soil BD, and the lowest r value was observed for the association between seedling growth characteristics and soil pH (Fig. 5).
Fig. 5 - Effect of machine traffic and slope on alder and maple seedling architecture. (RLM): lateral/main root length; (RMR): root mass ratio; (SMR): stem mass ratio; (RRS): main root/stem length; (RPL): root penetration/main root length; (LS): low slope; (HS): high slope.
Discussion
Changes in soil physicochemical properties
The results of the current study revealed that soil physicochemical properties significantly changed due to machine traffic. These results are similar to previous findings in the literature ([52], [15], [11], [16], [51], [44], [28], [42], [47]). Our findings showed that soil bulk density (BD), penetration resistance (PR) and pH increased, whereas total porosity (TP), macro porosity (MP), moisture content (MC), forest floor (FF), organic carbon (OC), nitrogen (N), phosphorus (P) and potassium (K) decreased, with an increasing number of machine passes. This observation has been confirmed by other researchers, including Von Wilpert & Schäffer ([54]), Marchi et al. ([37]), Naghdi et al. ([44]), Picchio et al. ([45]) and Mohieddinne et al. ([42]). Similar to our findings, Picchio et al. ([45]) demonstrated that the compaction of forest soils caused by heavy machinery is a common consequence of logging operations. Changes in soil physical properties are the most prominent indicator of soil disturbances following the use of logging equipment ([47]). In line with our results, Jourgholami et al. ([28]) reported that soil compaction occurs following ground-based skidding operations, which causes an increase in soil BD and a decrease in TP.
The results of our research showed that in addition to machine traffic, the longitudinal slope of the skid trail is one of the factors causing changes in the physicochemical characteristics of the soil, which is in line with the results of previous research ([51], [44], [28]).
Solgi et al. ([51]) reported no significant differences in TP between the undisturbed area and various points of the track across which the skidder passed once. However, differences were observed when traffic intensity increased to three passes (at a slope of ≥ 20 %) and more than six passes (at a slope of < 20 %). The authors also reported a decrease in TP ranging from 15.5% for low traffic intensity (one pass and a slope of < 20%) to 37.27% for severe treatments (15 passes and a slope of ≥ 20%).
Our results indicated additional BD increments of 49.3%, 17.9% and 8.3% after two, six and fifteen machine passes, respectively, in low slope (LS). The corresponding values in high slope (HS) were 59.2%, 16.5% and 7.1%, respectively. These results indicate that when the number of machine passes increases, the additional BD increment is negligible, aligning with the results of previous research ([51], [44],). Several researchers showed that one of the critical factors affecting the degree of soil compaction is the number of machine passes over a specific point, with most compaction occurring within the first 10 passes of a vehicle and the first three passes having the maximum impact. Subsequent passes generally have little additional effect ([1]). Matangaran & Kobayashi ([38]) found that BD increased markedly with the first and second pass of the tractor but did not change after the fifth pass.
The results of previous work evaluating two traffic frequencies (TIs) are in accordance with the results of our research. However, a divergence from our results is seen with increases in TI, such that our results showed significant differences in soil characteristics with TIs of six and fifteen compared with lower TIs.
Remarkably, our results revealed that PR increased by 30.5% and 38.9% after only two machine passes in LS and HS, respectively, compared with the control (from 1.05 to 1.37 MPa in LS and from 1.08 to 1.50 MPa in HS). Jakobsen & Greacen ([24]) reported that the values of soil PR increased by 0.2 to 0.3 MPa with each machine (forwarder) pass. Ampoorter et al. ([1]) additionally indicated that high soil PR was unfavorable to soil-dwelling fauna.
Seedling germination and growth response
Regeneration is a key process for the persistence of species in a community under varied environmental conditions ([32]). The results of this study revealed that machine traffic had a significant negative effect on seedling germination and growth. Similar results were obtained by Naghdi et al. ([44]), Jourgholami et al. ([26]), Picchio et al. ([46]) and Tavankar et al. ([53]). The mean germination rate (GR) of alder and maple seedlings on the control area was 58.3% and 46.1%, respectively, while after two, six and fifteen passes, the GR of alder seedlings reduced to 50%, 46.4% and 37.5%, respectively, while the GR of maple seedlings reduced to 36.1%, 28.6% and 25.6%, respectively. The GR of alder seeds was significantly higher than that of maple seeds in compacted soils. Naghdi et al. ([44]) showed that GR was strongly negatively correlated with soil BD. Additionally, the root length of the seedlings decreased consistently with increasing traffic frequency on all trail gradients and with increasing trail gradient at all traffic frequencies, with the greatest reductions at the highest traffic frequencies and steeper gradients. In compacted soils, forest regeneration can be impeded or even prevented for long periods ([47]). Jamshidi et al. ([25]) showed that soil compaction caused by machine-based skidding markedly reduced the main root growth of seedlings five months after planting.
Our results indicated that the stem and root growth of seedlings decreased with an increasing number of machine passes. After two passes, all seedling growth characteristics (except SD, the stem diameter of alder seedlings) significantly decreased compared with the control area; thus, stem length (SL), main root length (MRL) and total dry biomass (TDB) decreased by 28.7%, 34.9% and 34%, respectively, in alder seedlings and by 27.9%, 27.6% and 33.3%, respectively, in maple seedlings. These results show that even with low TIs, machine traffic had significant negative effects on seedling growth parameters. Similar results were also reported by Misra & Gibbons ([41]), Bassett et al. ([3]), Naghdi et al. ([44]) and Jourgholami ([27]).
The results of the current study revealed that machine traffic had a negative effect on seedling quality such that seedling quality index (SQI) decreased with an increasing number of machine passes. These results are in line with those of previous research by Picchio et al. ([45]) and Tavankar et al. ([53]), which demonstrated a lower SQI of beech seedlings on skid trails compared with uncompacted soils (control).
Our results revealed that the four architectural characteristics of seedlings (ratio of lateral to main root length, RLM; root mass ratio, RMR; stem mass ratio, SMR; and ratio of main root to stem length, RRS) were significantly affected by machine traffic. These findings were also confirmed by Cambi et al. ([7]), Jourgholami et al. ([28]) and Tavankar et al. ([53]). The RMR and RRS values of both alder and maple seedlings decreased with increasing TI. This is because the reductions in root growth and biomass are greater than the reductions in stem growth and biomass on compacted soils. The trends observed with respect to RLM and SMR values with an increasing number of machine passes were not regular and depended on the seedling species as well as the slope of the skid trail, in addition to machine traffic.
One of our results of interest indicated that seedling growth characteristics were significantly correlated with soil physicochemical properties; the highest correlation coefficient was found for the association between soil BD and the growth of the seedling root system. In accordance with our results, Picchio et al. ([46]), Jourgholami et al. ([28]) and Tavankar et al. ([53]) reported that SL, MRL, total, stem, and root dry masses, RMR and R/S were positively correlated with TP and MP and negatively correlated with soil BD and PR.
Reductions in air permeability and soil porosity reduce the elongation and penetration of roots, thus reducing access to, and uptake of, water and nutrients by roots ([20], [31], [6]). Soil pore size, water content, aeration, temperature, nutrient availability, and microorganisms are interrelated and can all be negatively impacted by soil compaction, leading to negative effects on plant root growth and functions ([10]). Soil compaction resulting from harvesting operations limit the effective rooting depth of plants by restricting access to water and nutrients and reducing gaseous exchange ([19]).
In compacted soil, seedlings and saplings are more susceptible to drought as the root system is prevented from quickly reaching deep and moist compartments ([3], [49], [48]).
Conclusions
To guarantee a proper sustainable management forest a continuous improvement of knowledge about the disturbs, in general, due to forest logging, and in particular, due to the impact of log skidding on soil and seedlings is needed. Overall, the results of this study showed that timber extraction by skidding, in addition to the initial negative effects on soil physical properties (such as increasing BD and PR and reducing soil pores) and soil chemical properties (such as reducing FF, OC, K, N and P and increasing pH), reduced the GR, below- and aboveground growth, and quality of maple and alder seedlings in later stages. These negative changes in the physical and chemical properties of the soil and the consequent decrease in the growth parameters and quality of seedlings may impair the sustainability and long-term productivity of the forest in the future. Specifically, we found that the number of machine passes and the longitudinal slope of the skid trails are important factors affecting the physicochemical properties of the soil and the subsequent growth of seedlings. According to the results of the present research, the amount of GR of alder seeds was higher than the amount of GR of maple seeds on compacted soils, therefore seedlings with alder species will more suitable than seedlings with maple species in compacted soils after the end of log skidding in these forests. Following the main results of this study, our suggestions for reducing the negative effects of skidding in the pre-harvest stage are: (i) limiting the surface disturbed by skid trails, through a synergistic planning and design of these temporary trails; (ii) designing skid trails with slopes of less than 15%; (iii) planning a restrained of the skidding pass repetition for every single trails.
Our main suggestions during the harvesting stage are: (i) implementing skidding when the soil is with low moisture content or is frozen; (ii) using logging residuals (branches and tops) on the skid trails during skidding in order to mitigate ground disturbance due to skidder traffic.
Finally, our suggestions in the post-harvest stage are: (i) using mulch on abandoned skid trails, especially, in particularly difficult environmental conditions; (ii) constructing water bars on active skid trails on loose soils with slopes > 10% and tracks longer than 50 m.
List of abbreviations
ANOVA: Analysis of variance; BD: Bulk density; CPs: Control plots; DBH: Diameter at breast height; FF: Forest floor; GR: Germination rate; HS: High slope, ≥20%; LRL: Lateral root length; LWT: Left wheel track; RWT: Right wheel track; RLM: Lateral/main root length; LM: Litter mass; LS: Low slope, <20%; MP: Macroporosity; MIP: Microporosity; MRL: Main root length; RRS: Main root/stem length; RWT: Right wheel track; MIP: Microporosity; MC: Moisture content; MRD: Main root diameter; N: Nitrogen; OC: Organic carbon; OP: Subplot; P: Phosphorus; PR: Penetration resistance; K: Potassium; RDB: Root dry biomass; RLM: Main root length; MRD: Main root diameter; RMR: Root mass ratio; RPD: Root penetration depth; RPL: Root penetration/main root length; RRS: Ratio main root/stem length; R/S: Root mass/shoot mass ratio; SH: Seedling height; SQI: Seedling quality index; SC: slope classes; SD: Stem diameter; SDB: Stem dry biomass; SL: Stem length; SKT: Skid trail; SMR: Stem mass ratio; SQI: Seedling quality index; TDB: Total dry biomass; TP: Total porosity; TI: Traffic intensity/ frequencies; PLs: Treatment plots; UC: Undisturbed control; SKT-A: Skit trail - Alder; SKT-M: Skid trail - Maple.
Authors’ contributions
Conceptualization: RN, FT, AS, MN; Methodology: RN, FT, AS, MN, EM, RP; Collection of data: RN, FT, AS, MN; Data analysis: RN, FT, AS, MN; Writing original draft preparation: RN, FT, AS, MN, EM, RP; Writing-review and editing: RN, FT, AS, MN, EM, RP; Funding acquisition: RN, FT, AS, MN; Supervision: FT, MN, EM, RP.
References
CrossRef | Gscholar
Gscholar
CrossRef | Gscholar
Authors’ Info
Authors’ Affiliation
Ahmad Solgi 0000-0001-5850-6328
Mehrdad Nikooy 0000-0002-4985-2648
Department of Forestry, Faculty of Natural Resources, University of Guilan, P.O. Box 1144, Somehsara, Guilan (Iran)
Department of Forestry, Khalkhal Branch, Islamic Azad University, Khalkhal 56817-31367 (Iran)
Department of Agricultural, Food, Environmental and Forestry Science and Technology, University of Florence, 50145 Florence (Italy)
Department of Agriculture and Forest Sciences - DAFNE, University of Tuscia, 01100 Viterbo (Italy)
Corresponding author
Paper Info
Citation
Naghdi R, Tavankar F, Solgi A, Nikooy M, Marchi E, Picchio R (2023). Effects on soil physicochemical properties and seedling growth in mixed high forests caused by cable skidder traffic. iForest 16: 127-135. - doi: 10.3832/ifor4103-016
Academic Editor
Manuela Romagnoli
Paper history
Received: Mar 22, 2022
Accepted: Feb 14, 2023
First online: Apr 23, 2023
Publication Date: Apr 30, 2023
Publication Time: 2.27 months
Copyright Information
© SISEF - The Italian Society of Silviculture and Forest Ecology 2023
Open Access
This article is distributed under the terms of the Creative Commons Attribution-Non Commercial 4.0 International (https://creativecommons.org/licenses/by-nc/4.0/), which permits unrestricted use, distribution, and reproduction in any medium, provided you give appropriate credit to the original author(s) and the source, provide a link to the Creative Commons license, and indicate if changes were made.
Web Metrics
Breakdown by View Type
Article Usage
Total Article Views: 19125
(from publication date up to now)
Breakdown by View Type
HTML Page Views: 16544
Abstract Page Views: 1263
PDF Downloads: 1097
Citation/Reference Downloads: 1
XML Downloads: 220
Web Metrics
Days since publication: 754
Overall contacts: 19125
Avg. contacts per week: 177.55
Article Citations
Article citations are based on data periodically collected from the Clarivate Web of Science web site
(last update: Mar 2025)
Total number of cites (since 2023): 3
Average cites per year: 1.00
Publication Metrics
by Dimensions ©
Articles citing this article
List of the papers citing this article based on CrossRef Cited-by.
Related Contents
iForest Similar Articles
Research Articles
Use of terrestrial laser scanning to evaluate the spatial distribution of soil disturbance by skidding operations
vol. 8, pp. 386-393 (online: 08 October 2014)
Research Articles
Influence of slope on physical soil disturbance due to farm tractor forwarding in a Hyrcanian forest of northern Iran
vol. 7, pp. 342-348 (online: 17 April 2014)
Research Articles
Impact of wheeled and tracked tractors on soil physical properties in a mixed conifer stand
vol. 9, pp. 89-94 (online: 22 May 2015)
Research Articles
Effect of forwarder multipassing on forest soil parameters changes
vol. 15, pp. 476-483 (online: 24 November 2022)
Research Articles
The natural recovery of disturbed soil, plant cover and trees after clear-cutting in the boreal forests, Russia
vol. 13, pp. 531-540 (online: 18 November 2020)
Research Articles
Estimating machine impact on strip roads via close-range photogrammetry and soil parameters: a case study in central Italy
vol. 11, pp. 148-154 (online: 07 February 2018)
Research Articles
Deploying an early-stage Cyber-Physical System for the implementation of Forestry 4.0 in a New Zealand timber harvesting context
vol. 17, pp. 353-359 (online: 13 November 2024)
Research Articles
Use of LIDAR-based digital terrain model and single tree segmentation data for optimal forest skid trail network
vol. 8, pp. 661-667 (online: 22 December 2014)
Research Articles
Effects of soil compaction on seedling morphology, growth, and architecture of chestnut-leaved oak (Quercus castaneifolia)
vol. 10, pp. 145-153 (online: 13 June 2016)
Research Articles
Identification of wood from the Amazon by characteristics of Haralick and Neural Network: image segmentation and polishing of the surface
vol. 15, pp. 234-239 (online: 14 July 2022)
iForest Database Search
Search By Author
Search By Keyword
Google Scholar Search
Citing Articles
Search By Author
Search By Keywords
PubMed Search
Search By Author
Search By Keyword