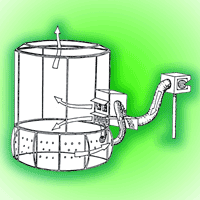
Open top chamber and free air CO2 enrichment - approaches to investigate tree responses to elevated CO2
iForest - Biogeosciences and Forestry, Volume 3, Issue 4, Pages 102-105 (2010)
doi: https://doi.org/10.3832/ifor0544-003
Published: Jul 15, 2010 - Copyright © 2010 SISEF
Review Papers
Collection/Special Issue: NFZ Summer School 2009 - Birmensdorf (Switzerland)
Long-term ecosystem research: understanding the present to shape the future
Guest Editors: Marcus Schaub (WSL, Switzerland)
Abstract
Open Top Chamber (OTC) and Free Air CO2 Enrichment (FACE) are currently the prevailing approaches to study plant responses to elevated carbon dioxide. Method-inherent characteristics of either method distinctively influence results. Advantages and disadvantages of both methods are reviewed here, leading to the conclusion that Open Top Chambers seem to be more suitable for investigating the physiological responses of single trees to high levels of carbon dioxide, while Free Air CO2 Enrichment systems are more useful for studying the effects of elevated carbon dioxide on whole forest ecosystems since they have a large diameter, thus allowing to work with larger trees. Free Air CO2 Enrichment systems also provide a natural microclimate, thus leading to ecologically more meaningful results. Methods involving Screen-Aided CO2 Control (SACC) are proposed as a compromise eliminating disadvantages and combining advantages of both the Open Top Chamber and the Free Air CO2 Enrichment methods. Considering the wide variety of experiments under a range of additional environmental factors it is difficult to identify a typical bias that may be inherent in the data generated by the Open Top Chamber and the Free Air CO2 Enrichment. Meta analysis of large number of past studies revealed that Open Top Chamber experiments produce a stronger growth enhancing effect of carbon dioxide than Free Air CO2 Enrichment experiments. Future comparative discussion of Open Top Chamber and Free Air CO2 Enrichment data needs to take into account this potential bias to yield biologically meaningful interpretations.
Keywords
Open Top Chamber (OTC), Free Air CO2 Enrichment (FACE), Tree response to elevated CO2, Screen-Aided CO2 Control, Chamber effect, Experimental bias, Elevated CO2 treatment
Introduction
Carbon dioxide (CO2) is the second most abundant greenhouse gas after water vapour. The concentration of greenhouse gases was almost constant in the pre-industrial era ([23], [6]). Due to anthropogenic activities such as burning fossil fuels and deforestation, the concentration of atmospheric CO2 has risen dramatically from 280 to 380 ppm since the beginning of the industrial revolution ([6]).
Along with oceans and the atmosphere, terrestrial ecosystems play an important role as a carbon reservoir in the natural carbon cycle. Growing forests act as a carbon sink by means of photosynthetic conversion of carbon dioxide to plant biomass. Mature forests are believed to show a neutral balance of photosynthetic carbon fixation and CO2 release by respiration (e.g., [8]). According to the latest studies, the world’s remaining old-growth forests are usually carbon sinks with positive net ecosystem productivity whereas only very few studies show old forests with a negative net carbon balance of the forest including soils ([19]).
Most physiological studies have shown that elevated CO2 induces changes in tree growth patterns, tissue structure and developmental processes. It influences the rate of physiological gas exchange resulting in enhanced net carbon assimilation (e.g., [25]). Furthermore, stomatal conductance is reduced ([11]) and Rubisco properties are altered ([2]). Moreover, elevated CO2 may affect cell division, expansion and patterning. Trees under elevated CO2 produce taller and thicker stems, increase total leave area and foliar starch concentration. In addition, shifts in timing of developmental phases may be observed ([2], [20], [14], [24]). Elevated CO2 can also inhibit various developmental effects of the hormone ethylene ([22]) and has a marked impact on soil nitrogen availability for plants by increasing soil net nitrification ([3]).
Over the years, a variety of techniques has been applied to study tree responses to elevated atmospheric CO2 at the plant organ, entire trees, or ecosystems. Early studies on the effects of elevated CO2 were typically done under controlled conditions in closed chambers or greenhouses, such as: a) branch and leaf chambers ([25]); b) phytotrons ([18]); c) controlled environment chambers ([16]); or variations thereof. A shortcoming of these difficult methods is that they create an artificial environment compared to natural ecosystem conditions. Attempts to bring experimental set-ups in a more natural context have yielded more elaborate techniques that tend to allow, to varying degrees, for an exchange with the natural environment. These include: d) open top chambers (OTC - e.g., [28]); e) free air CO2 enrichment systems (FACE - e.g., [15]); and f) screen-aided CO2 control (SACC - [17]).
OTC- and FACE-derived systems are nowadays the most frequently used methods to study tree responses to elevated CO2 under close to natural conditions ([17]). In this review, the principles of these two methods and the specific experimental approaches of OTC and FACE will be explained. Their advantages and disadvantages to individual research goals will be discussed. Screen-aided CO2 control (SACC) will be proposed as a possible compromise eliminating disadvantages and combining advantages of both OTC and FACE for many research applications. The second part of this review compares and validates the effects of elevated CO2 on plant biomass, leaf expansion, and photosynthesis obtained from OTC and FACE experiments aiming to identify method inherent biases.
Description of the technology
Open Top Chamber (OTC)
Open Top Chambers consist of metal constructions with transparent vertical side-walls (e.g., polyvinyl chloride, Plexiglas) and a frustum on top. An opening in the middle of the frustum allows an air exchange to reduce temperature and humidity effects in the chamber. CO2-enriched air is distributed from a circular tube, and air blowers ensure the uniform distribution of carbon dioxide within the chamber. The actual concentration of carbon dioxide within the OTC is measured by a CO2 analyzer and controlled by computer supported regulation of inlet valves (e.g., [14], [27], [28]).
Free Air CO2 Enrichment system (FACE)
In FACE systems, CO2 is transported by a ring-shaped pipe surrounding the plot and is distributed by vertically oriented pipes. The dosage of the carbon dioxide depends on the actual CO2 concentration inside the plot and climatic factors such as wind direction and speed. The valves of the vertical pipes can be closed and opened to adjust for changes in wind direction. To minimise experimental costs, CO2-enriched air can thus be released only upwind (e.g., [13], [11], [10], [29]).
Screen-Aided CO2 Control (SACC)
Screen-Aided CO2 Control consists of a transparent polycarbonate sheet mounted on a steel frame. CO2 is dispensed within the plot through a pipe with small holes attached below the screen. A gap between the soil surface and the distribution pipe, as well as the open top of SACC allows temperature, air humidity, and precipitation to equalize between plot and the surrounding field. Moreover, the gap enables small animals to access the plot. In comparison to FACE-methods, the total CO2 consumption is reduced due to the plastic screen acting as a windshield.
In OTC, FACE, and SACC, other factors (e.g., the ambient and elevated concentration of carbon dioxide, wind speed and direction, air pressure, photosynthetically active radiation, precipitation, air and soil temperature, soil moisture, and nutrient availability) need to be measured to clearly separate the influence of CO2 concentration from the possible influence of the other factors.
Evaluation of chamber effects in OTC and SACC plot
Closed side-walls and frustum in OTC create an artificial microclimate within the chamber that may increase temperature, alter humidity, photosynthetically active radiation and precipitation, and exclude interacting fauna and flora ([17], [27]). Therefore, it is important to validate the results from OTC with elevated CO2 by comparing with results from both OTC under ambient CO2 and open-air control plots. Compared to plants in open-air control plots, Pinus taeda grown in chambers (i.e., ambient CO2) showed a larger increase in height and the number of primary branches ([26]). However, this effect only became apparent after 15 month of growth, pointing to the importance of the control being included over the entire duration of the experiment. The severity of a chamber effect is likely to vary between species. This is illustrated in the work by Drake et al. ([7]) who noticed no difference between plant growth of community of Spartina patens, and mixed communities of Scirpus olneyi, Spartina patens and Distichlis spicata grown in OTC under ambient CO2 and in open-air control plots. In contrast, dry weight and shoot density of Scirpus olneyi grown in OTC under ambient CO2 were higher than in the control plot. The reasons for this can be: a) higher differences between midday air temperature inside and outside the chambers with Scirpus olneyi (2.7 ± 1.9 °C) in comparison to chambers with Spartina patens (2.1 ± 1.2 °C) and mixed communities of Scirpus olneyi, Spartina patens and Distichlis spicata (1.2 ± 1.4 °C - [7]); b) more light coming from the side into OTC because of damaged area surrounding the OTC with Scirpus olneyi ([7]).
Concerning SACC, no statistically significant difference in biomass accumulation and plant community composition between SACC experimental plots and the screenless control plots was found by Leadley et al. ([17]) under ambient CO2 in natural temperate grasslands.
Advantages and disadvantages of FACE and OTC
Earlier methods for investigating the effects of elevated CO2 on trees tended to concentrate on a single component approach, i.e., elevated CO2 as the single altered factor with other environmental conditions left unchanged. In contrast, both OTC and FACE methods are used with field conditions that aim to include a natural microenvironment and biotic interactions as part of the ecosystem studied. OTC and FACE differ, however, in their design. To choose which system is best suited for a particular research question, it is therefore important to consider the advantages and disadvantages of these methods.
FACE typically has a diameter between 1 m to 30 m to study not only seedlings but also mature trees (e.g., [13], [12]). Nevertheless, mature trees investigated in FACE usually have a life history of growing under an ambient CO2 concentration for a long time prior until the experiment takes place (e.g., [11]), and there might be a longer transition from a low to a high CO2 phenotype. On the other hand, OTC enables easily growing of plants under elevated CO2 for their entire lifetime (e.g., [26]). However, chamber size and project duration limit working with large forest trees. The construction of FACE does not negatively affect the plot’s microenvironment such as wind direction and speed, rain fall, snow fall, radiation, or the influence of insects. This enables the researcher to investigate the effects of elevated atmospheric CO2 on ecosystems under natural conditions (e.g., [17]).
One of the greatest disadvantages of FACE experiments is the very high cost arising from the high consumption of CO2 during fumigation. To lower the costs, transparent polyethylene windshields can be applied in the main wind direction ([11]), although they might influence the plot’s microclimate negatively. An additional disadvantage is that short-term CO2 fluctuations may be larger than within OTC’s experiments because wind has free access to the experimental plot ([13]).
In contrast to FACE, OTC systems have lower costs per experiment due to a significantly lower consumption of carbon dioxide, because air exchange is reduced by the closed side walls and frustum (see [28]). In contrast to experiments where the effects of elevated CO2 on plants are measured in the greenhouse, OTCs eliminate artefacts such as the growth of the trees being restricted by pots ([27], [28]).
OTCs have typically a smaller diameter than FACE. This makes them useful for working with seedlings but not with tall mature trees. This is particularly disadvantageous as the physiological response of seedlings and mature trees to elevated CO2 concentrations can be very different ([13]). A second major point of criticism in OTC is the possible chamber effects on microclimate, as outlined above.
In summary, OTCs are often used to investigate tree physiological responses to high levels of CO2 in the field under conditions near to the local natural conditions, and are thus in many cases superior to classical greenhouse or laboratory experiments as addressed in the introduction (a, b, c) that perform tests under well controlled, though entirely artificial conditions. While OTC experiments are not as close to the natural conditions as FACE, they have the advantage of lower cost.
FACE systems are valuable for the study of forest ecosystems. They involve fewer experimental artefacts than may be observed when using OTC, thus allowing studies that are closer to the natural, unaltered microclimate ([13]). The effects of elevated CO2 in a FACE study can be observed in relation to a wider range of variables, such as changing weather conditions, interactions among individual plants of one or several tree species.
A compromise can be found in the method of Screen-Aided CO2 Control (SACC), which can be used as an alternative to either FACE or OTC alone ([17]). This approach enables the investigation of tree responses to higher levels of CO2 under more natural conditions than in OTCs. In contrast to OTCs, the temperature peaks in midday are lower, rainfall and radiation are less altered and the interaction between plants and small animals is possible. Moreover, the experimental costs should be lower than for FACE ([17]).
Comparison of OTC and FACE results on tree CO2 responsiveness
To validate results of the effects of elevated CO2 on tree growth and development, comparative studies of one effect using different research approaches are necessary. Unfortunately, there are only few published results on biological effects of elevated CO2 comparing individual plants of the same tree species and of the same age, grown under similar growing conditions and identical CO2 treatments using both methods, OTC and FACE.
Tissue et al. ([26]) found that the response of Pinus taeda L. seedlings grown under elevated CO2 in an OTC experiment changed over the course of the experiment. Seedlings were grown in OTCs under ambient CO2 (control trees) or elevated CO2 (51.6 and 66.6 Pa) concentrations since germination. Elevated CO2 led firstly to rapid increase in plant biomass. After 11 months of CO2 exposition, the plant biomass of trees grown under 51.6 Pa or 66.6 Pa CO2 was 111 % and 233 % higher, respectively, than that of control trees grown under ambient CO2. During the following months, the differences in biomass accumulation between the plants grown with CO2 exposition or as control gradually diminished in both treatments and disappeared in the 51.6 Pa CO2-treatment after 19 months. Trees grown at 66.6 Pa CO2 were during this time “only” 111 % larger than control trees. The initial rapid increase of total plant biomass response to elevated CO2 followed by its decline can be explained by parallel changes in net assimilation rates over the study period. CO2-treated trees showed higher photosynthetic rates than plants grown under ambient conditions. However, the total Rubisco activity of trees under elevated CO2 was reduced in the first year pointing to an acclimation effect in plants exposed to elevated CO2 ([26], [9]). This photosynthetic acclimation connected with reduced levels of Rubisco proteins was also observed in one-year-old needles of well-developed, 16-year-old Pinus taeda growing for approx. 2.5 years under elevated CO2 in FACE system that was established in the same forest as the Pinus taeda seedling experiment addressed above. This Rubisco acclimation can be associated with an elevated content in soluble starch ([21]).
The response of leaf growth to elevated CO2 in hybrid poplar trees growing in OTC and FACE was studied by Taylor et al. ([24]). Young hybrid poplar trees (Populus x interamericana, P. x euramericana) showed a positive effect of elevated CO2 on leaf extension rate and total leaf area in all plants irrespective of whether trees were grown in OTC or FACE. However, the absolute rates of leaf extension in FACE-grown plants exceeded those of OTC-grown plants ([24]). According to Taylor et al. ([24]), the cause might have been different regimes of nitrogen, water, temperature and light that are inherent to the different systems. In contrast to the first year of FACE experiment, in the second year, the leaf extension rates by P. x euramericana grown under elevated CO2 decreased due to the acclimation effect under elevated CO2.
Another way of comparing and validating results of elevated CO2 effects on trees obtained from OTC and FACE experiments is the synoptic analysis of an available body of data using meta-analytical techniques. One of the parameters typically studied is total tree biomass. This response parameter to elevated CO2 ranged from a biomass reduction of 31 % to a 284% increase in biomass in relation to the ambient CO2 treatment ([4]). A meta-analysis can help interpreting such large differences, for example by detecting additional effects such as nutrient limitations. De Graaff et al. ([5]) found a significantly stronger CO2 induced increase in above-ground biomass in plants grown in OTC as compared to plants grown in FACE. These differences may result from a bias in age and size of trees used in either system. In OTC, mainly individual seedlings and young trees are used due to the size limitation of the chamber, while FACE is applied with older and larger trees in forests. The faster plant growth in OTC may also be caused by CO2 concentrations found in OTC systems being typically higher than those in FACE ([4], [5]). Furthermore, an altered stomatal CO2 responsiveness caused by short-term CO2 fluctuations in FACE is a possibility, which is however challenged by Hendrey et al. ([13]). Also OTC favours in-chamber temperature peaks on hot and sunny days thus potentially boosting photosynthetic rates and above-ground biomass growth ([17]). On the other hand, the side walls and the frustum of the OTC have a screening effect thus reducing the available photosynthetic active radiation ([27], [28]). Free access of small herbivorous mammals into FACE may cause browsing damage to trees, whereas trees in OTC are better protected.
In summary, the body of work reviewed here, suggests that the method of CO2 treatment can greatly influence the tree responses that are measured, thus limiting the comparability of data from either FACE or OTC. To collect biologically more meaningful data that are less biased by the choice of the experimental set-up, different experimental approaches should be applied in parallel, including both seedlings and mature trees as test objects. Furthermore, a comprehensive documentation of other factors (e.g., wind and light characteristics, precipitation, air and soil temperature, soil moisture, nutrient availability and potential chamber effects) increases the information value and comparability of data generated by either approach.
Meta-analytical techniques applied to results of past studies on tree responses to elevated CO2 are useful instruments for a better understanding of CO2 effects on plants. For example nutrient availability plays an important role for tree response to elevated CO2 ([14]). According to De Graaff et al. ([5]), high nitrogen concentration in the soil significantly increased the plant responses to elevated CO2 in form of an increased production of aboveground plant biomass. Meta-analysis can also be used to highlight differences in responsiveness to elevated CO2 between different types of plants. For instance, according to the meta-analysis by De Graaff et al. ([5]), woody species show significantly stronger responses in above-ground biomass to elevated CO2 than herbaceous species which is in accordance with the data by Ainsworth & Long ([1]).
Conclusion
This review discusses potential Open Top Chamber and Free Air CO2 Enrichment systems as methods to investigate the effects of elevated CO2 on single mature trees and seedlings. The OTC is useful for studying mechanistic tree physiological responses to elevated CO2, whereas FACE allows assessing the effects of elevated CO2 in entire forest ecosystems. The method of Screen-Aided CO2 Control can provide an alternative by combining the advantages and eliminating the disadvantages of OTC and FACE.
Experimental bias differs between OTC and FACE and thus comparability of data generated by these methods is limited. In interpretation and discussion of data from past experiments this potential bias has to be considered to draw biologically more meaningful conclusions. The experimental design of future experiments must be based on the specific biological question rather than the availability of either system.
Acknowledgements
I would like to thank the German Academic Exchange Service (DAAD) for financial support for my PhD, Marcel Robischon for proof-reading this review and reviewers for the valuable comments.
References
Gscholar
Gscholar
Gscholar
Gscholar
Gscholar
Authors’ Info
Authors’ Affiliation
Chair of Tree Physiology, Institute of Forest Botany and Tree Physiology, Albert-Ludwigs-Universität Freiburg, Georges-Köhler Allee, Geb. 53/54, D-79085 Freiburg (Germany)
Corresponding author
Paper Info
Citation
Macháčová K (2010). Open top chamber and free air CO2 enrichment - approaches to investigate tree responses to elevated CO2. iForest 3: 102-105. - doi: 10.3832/ifor0544-003
Paper history
Received: May 25, 2010
Accepted: May 31, 2010
First online: Jul 15, 2010
Publication Date: Jul 15, 2010
Publication Time: 1.50 months
Copyright Information
© SISEF - The Italian Society of Silviculture and Forest Ecology 2010
Open Access
This article is distributed under the terms of the Creative Commons Attribution-Non Commercial 4.0 International (https://creativecommons.org/licenses/by-nc/4.0/), which permits unrestricted use, distribution, and reproduction in any medium, provided you give appropriate credit to the original author(s) and the source, provide a link to the Creative Commons license, and indicate if changes were made.
Web Metrics
Breakdown by View Type
Article Usage
Total Article Views: 65880
(from publication date up to now)
Breakdown by View Type
HTML Page Views: 54683
Abstract Page Views: 3893
PDF Downloads: 6312
Citation/Reference Downloads: 43
XML Downloads: 949
Web Metrics
Days since publication: 5468
Overall contacts: 65880
Avg. contacts per week: 84.34
Article Citations
Article citations are based on data periodically collected from the Clarivate Web of Science web site
(last update: Mar 2025)
Total number of cites (since 2010): 21
Average cites per year: 1.31
Publication Metrics
by Dimensions ©
Articles citing this article
List of the papers citing this article based on CrossRef Cited-by.
Related Contents
iForest Similar Articles
Review Papers
Field experiments using CO2 enrichment: a comparison of two main methods
vol. 3, pp. 109-112 (online: 15 July 2010)
Technical Reports
Canopy Chamber: a useful tool to monitor the CO2 exchange dynamics of shrubland
vol. 10, pp. 597-604 (online: 15 May 2017)
Research Articles
Species-specific morphological and physiological characteristics and progressive nitrogen limitation under elevated CO2 concentration
vol. 13, pp. 270-278 (online: 03 July 2020)
Research Articles
Changes in the proteome of juvenile European beech following three years exposure to free-air elevated ozone
vol. 4, pp. 69-76 (online: 05 April 2011)
Research Articles
The use of branch enclosures to assess direct and indirect effects of elevated CO2 on photosynthesis, respiration and isoprene emission of Populus alba leaves
vol. 1, pp. 49-54 (online: 28 February 2008)
Research Articles
Response of juvenile progeny of seven forest tree species and their populations to simulated climate change-related stressors, heat, elevated humidity and drought
vol. 11, pp. 374-388 (online: 15 May 2018)
Research Articles
Adaptability of Indocalamus decorus to climate change based on physiological and biochemical responses to elevated carbon dioxide and ozone
vol. 9, pp. 311-317 (online: 22 October 2015)
Research Articles
Retranslocation of foliar nutrients of deciduous tree seedlings in different soil condition under free-air O3 enrichment
vol. 9, pp. 835-841 (online: 17 June 2016)
Research Articles
Performances of an expanding insect under elevated CO2 and snow cover in the Alps
vol. 1, pp. 126-131 (online: 27 August 2008)
Research Articles
Photosynthesis of three evergreen broad-leaved tree species, Castanopsis sieboldii, Quercus glauca, and Q. myrsinaefolia, under elevated ozone
vol. 11, pp. 360-366 (online: 04 May 2018)
iForest Database Search
Google Scholar Search
Citing Articles
Search By Author
Search By Keywords