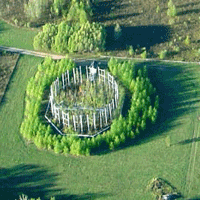
Field experiments using CO2 enrichment: a comparison of two main methods
iForest - Biogeosciences and Forestry, Volume 3, Issue 4, Pages 109-112 (2010)
doi: https://doi.org/10.3832/ifor0545-003
Published: Jul 15, 2010 - Copyright © 2010 SISEF
Review Papers
Collection/Special Issue: NFZ Summer School 2009 - Birmensdorf (Switzerland)
Long-term ecosystem research: understanding the present to shape the future
Guest Editors: Marcus Schaub (WSL, Switzerland)
Abstract
The dramatic increase in global atmospheric carbon dioxide over the past century is hypothesized to have significant impacts on the earth system. To understand the effects of elevated CO2 on terrestrial ecosystems, two main methods have been used to simulate an increase of CO2 in a semi-controlled field setting: 1) Open Top Chambers (OTC); and 2) Free Air CO2 Enrichment (FACE). The OTC method has been applied to study the components of forest ecosystems at small scale by manipulating seedlings or isolated juvenile trees, but is not able to address ecosystem processes as a whole. For technical reasons, OTC cannot be used to consider scaling issues, interaction with the boundary layer, and competition among species. To address these issues FACE technology was developed. FACE enables longer-term studies in larger plots, and allows studies of plant processes such as leaf area and canopy development, canopy energy balance and canopy gas exchange. In this review, I synthesize results from literature, in particular from meta-analysis techniques applied either to OTC or FACE. The results are qualitatively similar: CO2 enrichment leads to reduced stomatal conductance and leaf nitrogen, and enhanced photosynthesis and production. However, photosynthesis and crop yield were lower in FACE experiments than OTC, while starch content was higher. These results provide support for ecosystem model simulations, and help fill the gap between individual plants, forest and regional ecosystem. Neither OTC nor FACE can provide a clear indication of the regional-scale feedbacks between atmosphere and vegetation that might be expected under elevated CO2. To address this issue, further research is needed.
Keywords
Photosynthesis, Free-Air Carbon dioxide Enrichment FACE, Open top chamber, Carbon sequestration, Forest ecosystem
Introduction
Global atmospheric CO2 concentration has increased from a pre-industrial value of about 280 ppm in 1850 to 387 ppm in 2009 ([19]) and it will probably exceed 700 ppm by the end of the 21st century ([7]). A doubling of atmospheric CO2 will most likely lead to a global warming of 3-5 °C ([8]), whilst also inducing other non-climatic changes in the Earth system, particularly in the terrestrial biosphere which uses CO2 for photosynthesis. It is crucial to understand the consequences of elevated CO2 on terrestrial ecosystems, since land plants, through the process of carbon sequestration, can take up part of the atmospheric CO2 emitted by human activities, potentially slowing the increase of CO2 in the atmosphere and delaying climate change.
Körner ([10]) estimated that the global terrestrial biosphere sequesters 1-2 Pg C/yr (Fig. 1). However, much uncertainty exists in quantifying carbon sequestration due to natural variability in carbon pools and fluxes among the different terrestrial ecosystems ([22]).
Fig. 1 - Major components involved in the global biological carbon cycle, a synthesis from many sources (from: [10]).
Therefore, there is a need for a better understanding of the physiological responses of plant and forest ecosystems to elevated CO2. This information can provide support for ecosystem models and fill the gap between individual plants, forests and regional ecosystems.
To address these questions, a number of methodologies have been developed since the 1970s to simulate the effects of elevated CO2 concentrations on plants. Most of these methods used leaf cuvettes, plant grow chambers and greenhouses ([24]). However, these methods have important constraints in plot and plant size, and require active control of all environmental variables ([23]). To overcome some of these limitations, other methodologies were developed that could be performed under unenclosed conditions: Open Top Chamber (OTC), Free Air CO2 Enrichment (FACE) and Screen-aided CO2 Control (SACC).
OTCs are made of a plastic enclosure with inclined walls and an open top while FACE is characterized by a series of vertical vent pipes, placed circularly around the plot, which release CO2 towards the centre of the ring. SACC is a middle ground between FACE and OTC; it includes screens to break the wind minimizing its effects on the microclimate (a well-known problem in OTC). Windscreens also reduce the amount of CO2 to be used and therefore its often-prohibitive costs (Leadley et al 1997).
OTC studies provided knowledge of mechanistic plant physiological responses such as stomatal conductance (Gs), transpiration, respiration, down-regulation of photosynthesis and yield. Those processes were further investigated under real forest conditions, by developing FACE technology ([6]). Although SACC resulted to be a good compromise between FACE and OTC, especially in a grassland environment ([13], [11], [18], [24]), it is still not able to replace FACE in a forest environment. Therefore in this mini-review paper I will only focus on FACE and OTC, which are also considered the two main methods used for CO2 enrichment.
I will review the design, advantages, and limitations of both methods, and discuss common and contrasting results of studies using either method. I have placed a particular emphasis on evaluating the effects of side walls in OTC, especially regarding microclimate (temperature, humidity, solar radiation and wind) and plant-atmosphere feedbacks.
OTC and FACE design
OTCs are made of a plastic enclosure with inclined walls and an open top. Air enriched with CO2 enters near the bottom and flows out the open top creating an enriched CO2 environment inside the chamber (Fig. 2). FACE, by contrast, is characterized by a series of vertical vent pipes, placed circularly around the plot, which release CO2 towards the center of the ring. CO2 concentration, wind velocity and wind direction are continuously measured and the information collected are used by a computer-controlled systems to maintain elevated concentration of CO2 throughout the plot ([2] - Fig. 3). Generally the CO2 concentration-using OTC is maintained at 700 ppm while in FACE concentrations between 550 and 600 ppm are more typical.
Fig. 2 - Open-top field chamber design. The diagram shows the air path through the chamber and its relative size (from: [2]).
Fig. 3 - FACE plots at the Aspen FACE experiment. [online] URL: ⇒ http://aspenface.mtu.edu/
Advantages and limitations of OTC and FACE
The main aspects of the two techniques are summarized in Tab. 1. In the OTC the presence of side walls limits CO2 consumption but induces a significant impact on the microclimate, altering air flow, intercepting rainfall, restricting access to insect pollinators and pests, increasing air temperature and water vapour humidity and lowering transmittance on sunny days ([12], [17]). In OTC, the wind is removed, preventing wind effects and dispersal of pathogens and pests. In FACE experiments, microclimate is minimally affected, but large quantities of CO2 are required to compensate the CO2 that diffuses away from the plot, especially under windy conditions.
Tab. 1 - Main aspects of the two major techniques used for CO2 enrichment.
FACE (Free Air CO2 Enrichment) |
OTC (Open Top Chamber) |
---|---|
- Diameter (8-30 m) - Sidewalls absent - CO2 distributed by a ring of vertical pipes - Computer-controlled system that adjusts CO2 flow rate - Forest canopy fully developed - Species can compete - High costs - Blower effect under still wind conditions - Step increase in CO2 |
- Diameter (~1 m) - Presence of sidewalls - CO2 distributed by a circular tube - UV-B are not transmitted trough the walls - No wind from outside - Increased temperature - No dispersion of pathogen and pests - Plant-atmosphere coupling is altered - Forest-ecosystem processes cannot be studied - Rooting barrier |
An additional limitation of OTC is the presence of a rooting barrier that prevents roots from exploiting soil outside the chamber and vice-versa, eventually inducing feedback inhibition on photosynthesis and production ([17]).
While OTC is made of inexpensive materials and requires low amounts of CO2, FACE requires a high investment in instrumentation, building material, CO2 and transport.
The major limitations of OTC in a forest environment are: the influence of trees and stand development patterns, the lack of an ecosystem prospective, scaling issues and absence of boundary layers. Trees and forests are very well coupled to the atmosphere, but this coupling is often greatly perturbed when enclosed in chambers ([16]). In the OTC method, ventilation disables the natural coupling between vegetation and atmosphere. The applied artificial turbulence alters the exchanging process between canopy and atmosphere, by means of periodic irruptions of air in the canopy instead of a continuous mixing as it occurs in a natural environment.
In FACE experiments, the components of the plant-soil nutrient cycle can be integrated, species can compete for resources, and a forest canopy may fully develop ([20]).
However, FACE also has several important limitations. For example, CO2-enriched through vent-pipes has the potential to cause microclimate perturbations (“blower effect”) under very stable and calm atmospheric conditions as during still nights ([6]).
FACE experiments typically impose a steep increase in CO2 concentrations at the beginning of the experiment. This abrupt change in environmental conditions may induce different responses of plants and ecosystem processes that have grown under normal CO2 for decades. In particular, enhanced photosynthesis induces an elevation in nitrogen-demand, which often leads to nutrient stress and consequent down-regulation of photosynthesis ([6]).
Finally, even though FACE experiments are sufficiently large to capture most critical ecosystem processes, they are still like an island within the surrounding ecosystem ([6]).
Results and discussion
A considerable number of papers have been published that investigated plant responses to elevated CO2. Here we focus on reviews, where meta-analytic techniques have been adopted for quantitatively analyzing the results obtained by independent experiments made using chambers and FACE methodology. A synthesis of the results obtained with these studies is presented (Tab. 2).
Tab. 2 - Summary of meta-analysis results from FACE and chamber techniques and literature reviews. Gs: stomatal conductance; Asat: light saturated CO2 uptake. Sources: (a): [1]; (b): [4]; (c): [17]; (d): [5]; (e): [14].
Parameters | FACE (%) | Chambers (%) |
---|---|---|
Asat | 47 a | 31 b |
Above-ground dry-matter | 28 a | 28.8 b |
Yield | 17 a | 28-35 b |
Gs | -20 a | -20 b |
Starch content | 83 c | 67.6 b |
Leaf Nitrogen | -4 a | -15 b |
Rubisco | -20 c | -20 b, d |
Photosynthesis | 30 c | 53 b |
Dark respiration | 0 e | 15-20 b, d |
Conclusions mainly drawn from chamber studies suggest that an increase in CO2 induced a reduction in Gs and transpiration while improved water-use efficiency, photosynthesis and light use efficiency ([5]). Drake et al. ([5]) also found that due to increased soil water content, water use efficiency and growth would be enhanced an that photosynthesis and growth increased even when N is limited, because of higher nitrogen use efficiency. In contrast, Norby et al. ([20]) reported from a meta-analysis of OTC experiments little evidence of Gs reduction, furthermore photosynthesis and growth did not increase where N is limiting. Results from Ainsworth & Long ([1]) and Curtis & Wang ([4]) confirm the findings of Drake et al. ([5]), reporting a 20% decrease in Gs.
Ainsworth & Long ([1]), found an increase in light-saturated carbon uptake (Asat) for trees, grass and crop of 47%, 37% and 17% respectively. Different functional group responses are also found by Norby et al. ([20]) regarding above-ground growth.
Norby et al. ([20]) found an increase of fine root density between 60 and 140% in elevated CO2. This induces an increase of carbon in the soil profile suggesting that forests may have more potential for C sequestration that may be apparent from aboveground analysis ([21]).
The lower increase in crop yield and the 20% increase in photosynthesis reported in FACE compared with chamber studies could be explained by the lower CO2 concentration of FACE (600 ppm) compared with chambers (700 ppm). However, as yield and photosynthesis responses are not linear, the alteration of microclimate in OTC could underestimate the effect of elevate CO2 on yield and photosynthesis.
Leaf Area Index (LAI) of seedlings and saplings grown in OTC has usually increased with CO2 enrichment ([20]), while no increases in LAI are reported in most of the FACE experiments ([1], [5]). This is in contrast with results from global vegetation models, which report an increase in LAI; consequently they may overestimate future evapotranspiration and photosynthesis carbon uptake ([17]).
Plant starch content observed by Long et al. ([17]) in a FACE study was 15.4% higher than the one observed by Curtis & Wang ([4]) using OTC. Plants grown in chambers receive less light than in FACE because of the effect of the sidewalls; this effect may be the cause of these contrasting results.
Chamber studies showed that the initial stimulation of photosynthesis and growth diminishes or disappears in the long term, while FACE studies show that there is little or no evidence of loss of stimulation of photosynthesis on the long-term ([17]). Reduction in stimulation could be the result of either down-regulation by carbohydrate accumulation or acclimation ([20]).
Arp ([3]) reported that rooting volume suppressed the response of plants to elevated CO2, demonstrating that loss of a response to increased CO2 through acclimation was an artifact of pot size (the “pot effect”). Experiments at the Oak Ridge FACE site confirm that there has been no evidence for acclimation of photosynthesis to elevated CO2 ([21]). However, a mechanism that fully explains this response is not yet known.
Dark respiration is usually inhibited by 15-20% in chamber studies while FACE experiments on average did not observe increased dark respiration ([14]).
Conclusions
CO2 enrichment studies using OTC are useful for research conducted at a small scale such as seedlings or juveniles, where detailed measurements are conducted to provide a fundamental and mechanistic understanding of component plant-processes. For investigations at ecosystem scale, FACE experiments are the only currently available method of providing realistic CO2 enrichment. Direct scaling from OTC to FACE is difficult because seedlings or saplings respond differently as compared with mature trees: competition is altered, plant architecture and species composition is different, leaf area and tree density may change.
FACE experiment provides support for the inclusion of a carbon sequestration effect into models of the future trajectory of the global carbon cycle ([21]).
Elevated CO2 leads to a decrease in Gs and therefore to a reduction in transpiration. It has been hypothesized that CO2 enrichment acting at regional scales (> 100 km2) may result in the drying of the lower troposphere. This in turn could increase evaporative demand on plants. But quantifying this feedback is difficult. Neither OTC nor FACE can provide an answer to this question that implies a direct action of the vegetation on the atmosphere.
Predicting the future responses of ecosystems to elevate CO2 remains difficult. This is because species respond differently and the complex interaction between plants, soils, pests and pollutants are difficult to detect. A description of the different species responses to CO2 enrichment, especially in form of functional groups, could be important for ecosystem models.
FACE is the best methodology available even though the study site remains an island in the host ecosystem and large-scale feedbacks cannot be detected ([6]). There is a need for studies under realistic conditions where trees are exposed to elevated CO2 for their entire life span of the stand, with natural stresses and where species can compete with each other ([9]. Further studies conducted in natural springs, where the local vegetation has been exposed to elevated CO2 for decades, could help to improve our understanding.
Acknowledgements
I thank Dr. Marcus Schaub and Dr. Erwin Dreyer for beneficial discussions during the WSL Summer School that inspired me to write this review and Prof. Franco Miglietta, Prof. Jed Kaplan and Dr. Basil Davis for their valuable comments, which improved this manuscript. The Italian Ministry of Education (FIRB) through project CASTANEA (contract no. 511305) provided financial support for this work and my participation in the WSL Summer School.
List of abbreviations
The following abbreviations have been used throughout the manuscript:
- CO2: carbon dioxide;
- Gs: stomatal conductance;
- OTC: Open Top Chamber;
- FACE: Free Air CO2 Enrichment;
- LAI: Leaf Area Index;
- Asat: light saturated carbon uptake.
References
Gscholar
Gscholar
Gscholar
Gscholar
Gscholar
Gscholar
Gscholar
Gscholar
Authors’ Info
Authors’ Affiliation
Soil-Vegetation-Atmosphere Research Group Institute for Environmental Science and Technology, Ecole Polytechnique Fédérale de Lausanne, Station 2, CH-1015 Lausanne (Switzerland)
Corresponding author
Paper Info
Citation
Mauri A (2010). Field experiments using CO2 enrichment: a comparison of two main methods. iForest 3: 109-112. - doi: 10.3832/ifor0545-003
Paper history
Received: May 25, 2010
Accepted: Jun 01, 2010
First online: Jul 15, 2010
Publication Date: Jul 15, 2010
Publication Time: 1.47 months
Copyright Information
© SISEF - The Italian Society of Silviculture and Forest Ecology 2010
Open Access
This article is distributed under the terms of the Creative Commons Attribution-Non Commercial 4.0 International (https://creativecommons.org/licenses/by-nc/4.0/), which permits unrestricted use, distribution, and reproduction in any medium, provided you give appropriate credit to the original author(s) and the source, provide a link to the Creative Commons license, and indicate if changes were made.
Web Metrics
Breakdown by View Type
Article Usage
Total Article Views: 58591
(from publication date up to now)
Breakdown by View Type
HTML Page Views: 50372
Abstract Page Views: 3147
PDF Downloads: 4058
Citation/Reference Downloads: 29
XML Downloads: 985
Web Metrics
Days since publication: 5464
Overall contacts: 58591
Avg. contacts per week: 75.06
Article Citations
Article citations are based on data periodically collected from the Clarivate Web of Science web site
(last update: Mar 2025)
Total number of cites (since 2010): 4
Average cites per year: 0.25
Publication Metrics
by Dimensions ©
Articles citing this article
List of the papers citing this article based on CrossRef Cited-by.
Related Contents
iForest Similar Articles
Review Papers
Open top chamber and free air CO2 enrichment - approaches to investigate tree responses to elevated CO2
vol. 3, pp. 102-105 (online: 15 July 2010)
Research Articles
Adaptability of Indocalamus decorus to climate change based on physiological and biochemical responses to elevated carbon dioxide and ozone
vol. 9, pp. 311-317 (online: 22 October 2015)
Research Articles
Voluntary carbon credits from improved forest management: policy guidelines and case study
vol. 11, pp. 1-10 (online: 09 January 2018)
Review Papers
Comparative assessment for biogenic carbon accounting methods in carbon footprint of products: a review study for construction materials based on forest products
vol. 10, pp. 815-823 (online: 25 September 2017)
Research Articles
Modeling air pollutant removal, carbon storage, and CO2 sequestration potential of urban forests in Scotlandville, Louisiana, USA
vol. 9, pp. 860-867 (online: 22 September 2016)
Research Articles
Exploring machine learning modeling approaches for biomass and carbon dioxide weight estimation in Lebanon cedar trees
vol. 17, pp. 19-28 (online: 12 February 2024)
Research Articles
Roadside vegetation: estimation and potential for carbon sequestration
vol. 3, pp. 124-129 (online: 27 September 2010)
Research Articles
Forest management with carbon scenarios in the central region of Mexico
vol. 14, pp. 413-420 (online: 15 September 2021)
Research Articles
Identifying priority conservation areas for above-ground carbon sequestration in Central Mexico
vol. 10, pp. 923-929 (online: 07 December 2017)
Editorials
Change is in the air: future challenges for applied forest research
vol. 2, pp. 56-58 (online: 18 March 2009)
iForest Database Search
Search By Author
Search By Keyword
Google Scholar Search
Citing Articles
Search By Author
Search By Keywords
PubMed Search
Search By Author
Search By Keyword