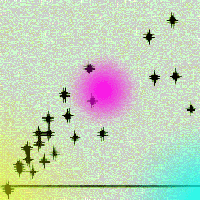
The use of branch enclosures to assess direct and indirect effects of elevated CO2 on photosynthesis, respiration and isoprene emission of Populus alba leaves
iForest - Biogeosciences and Forestry, Volume 1, Issue 1, Pages 49-54 (2008)
doi: https://doi.org/10.3832/ifor0429-0010049
Published: Feb 28, 2008 - Copyright © 2008 SISEF
Research Articles
Abstract
We used a novel system of branch enclosures to study the impact of elevated CO2 (900 ppm) on the gas-exchange characteristics of developed and developing leaves of white poplar (Populus alba L.), as well as of leaves subsequently developing at ambient CO2, outside the enclosures in which the CO2 concentration was raised. We found no significant effect of elevated CO2 on photosynthesis, respiration and isoprene emission, as the rates of developed and developing leaves inside the enclosures, and of leaves developing outside the enclosures, were similar to those recorded using enclosures maintained at ambient CO2. The enclosure system, however, largely influenced the rates of gas-exchange. In fact, leaves already developed inside the enclosures showed rates of photosynthesis, stomatal conductance, and isoprene emission higher than leaves developing inside the enclosures, and also higher than leaves developing outside the enclosure. These differences were caused by a higher efficiency in the light use and by a higher Ribulose 1.5 bisphosphate carboxylase (Rubisco) activity in leaves fully developed inside enclosures than in the other leaf classes. The experiment overall suggests that branch enclosures may alter the physiology of the plants, reducing or counteracting the impact of elevated CO2, which we predicted to stimulate photosynthesis and uncouple isoprene emission from photosynthesis. This may be an important bias against the use of enclosure systems for studies of the impact of environmental constraints and global change factors on physiological features.
Keywords
Populus alba, Elevated CO2, Branch Enclosure, Photosynthesis, Isoprene Emission
Introduction
Forests emit a wide range of volatile organic compounds (VOCs), mainly isoprenoids (e.g., [6]). Isoprenoids may provide enhanced leaf thermotolerance ([18]), and/or may scavenge ozone and help provide protection against oxidative stress ([11]) but none of these hypothesis have been proved beyond reasonable doubt. Some isoprenoids are also active molecules in plant defense against biotic stresses, deterring herbivores from feeding and protecting wounded parts of the plants from invasion by bacteria and fungi ([5]).
Biogenic VOC emission rates can also have direct and indirect effects on the carbon cycle at terrestrial level. A direct effect occurs because a part of the carbon assimilated by plants through photosynthesis is immediately re-emitted in the atmosphere as biogenic VOCs. In general about 1-2% of the carbon fixed by photosynthesis is released as isoprene by leaves ([19]), and this percentage can increase dramatically in stressed plants ([17]). The indirect effect of biogenic VOCs on the carbon cycle is linked to the high reactivity of these compounds with anthropogenic and natural compounds (mainly NOx) leading to high ozone episodes and photochemical smog, particularly during periods of high radiation and temperature, when biogenic VOC emissions are usually high ([1]). This in turn causes the accumulation of slowly reacting compounds, such as CH4 and CO2, thus indirectly potentially contributing to the global warming associated to the build-up of greenhouse gases in the atmosphere ([4]).
In the context of global climate change, there is therefore considerable interest in understanding how isoprenoids emission may change in the future, especially in response to CO2 concentration increase. The predicted increase of atmospheric CO2 concentration is expected to increase photosynthetic rates in C3 plants both by increasing the rate of carbon fixation and by reducing photorespiratory loss of carbon ([2]). However, in the long term, elevated CO2 may lead to a decline in the concentration of Rubisco and pigments of the light-harvesting system, resulting in a down-regulation of photosynthetic capacity ([2]).
Unlike photosynthesis, phytogenic isoprenoid emissions are relatively insensitive to, or are even negatively affected by elevated CO2 ([19], [9]). Sharkey et al. ([16]) found that the basal emission in Populus tremuloides seedlings grown in growth chambers was reduced by 30-40% in response to elevated CO2 (900 ppm). A similar result was obtained in a cottonwood (Populus deltoides) plantation exposed to elevated CO2 ([14]). Other reports, however, do not show such a reduction of isoprene emission upon growth at elevated CO2. As Rosenstiel et al. ([14]) showed, increased CO2 may uncouple photosynthetic process from isoprene emission process as a consequence of different isoprene synthase activity or reduced synthase substrate (i.e., dimethylallyl diphosphate, DMAPP) availability. Moreover, elevated CO2 exposure may vary isoprene emission altering the partioning of phosphoenolpyruvate (PEP) between mitochondrial and chloroplastic process.
To further investigate the interesting, and apparently often conflicting, response of isoprene to elevated CO2, we set up an experimental system which allowed us to study how growth of expanded leaves at elevated CO2 affects the isoprene emission, as well as primary metabolism, of new leaves expanding at ambient CO2. Specifically, we wanted to investigate whether the negative impact of elevated CO2 on isoprene emission could give origin to a metabolic signal affecting isoprene emission of other parts of the same plant not directly exposed to elevated CO2.
Materials and methods
Plant material and experimental set up
Poplar (Populus alba) saplings were grown in a greenhouse during April-July at CNR research area (Monterotondo, 42° N, Italy). The greenhouse was shaded to reduce temperature during summer. The average temperature during the day increased from 27°C in April to 33°C in July while the light intensity at the plant levels never exceeded 1000 μmol photons m-2 s-1 during bright, sunny days (> 80% of the days during which the experiment was carried out).
When three to four leaves developed (at the beginning of June), the branches were enclosed in soft transparent Teflon bags (2 L vol) mounted on a plastic frame to avoid direct contact of the film with the leaves. The bags were flown with 3 L min-1 air containing a RH of 50-70 % and ambient (380 ppm) or elevated CO2 concentrations (900 ppm). Elevated CO2 was obtained diluting pure CO2 gas cylinders with ambient air using mass flow controllers. The upper bud was not enclosed in the bag but was allowed to develop at ambient CO2 and three to four new leaves were expanded outside the bag by the end of the experiment (after 30 days). At this moment we measured the gas exchange of CO2, H2O and isoprene, and chlorophyll fluorescence, from the following three classes of leaves: a) leaves expanded ex novo at ambient conditions outside the bags; b) developed leaves which were enclosed in the bags and exposed to elevated CO2); c) leaves developing inside the bags at elevated CO2 during the experiment and not developed at the beginning of the experiment. These leaves will be referred to as A, B, and C, respectively.
Gas-exchange measurements
Gas exchange measurements were performed after a 30-days long fumigation, by temporary removing the leaves B and C from the bags while this was not needed for leaves of class A. A portion of the leaf was clamped in the cuvette of a portable Li-Cor 6400 (Li-Cor, Lincoln, NE, USA) gas exchange system as described by Scholefield et al. ([15]). This system allows very fast changes of CO2 concentration (380 ppm or 900 ppm) and source (12CO2 or13 CO2) or light intensity while controlling all other environmental parameters. Photosynthesis (A), stomatal conductance (gs ), transpiration (Tr), intercellular CO2 concentration (Ci), and mitochondrial respiration in the dark (Rn) and in the light (Rd) were measured both at normal and elevated CO2 while maintaining leaves at 30 °C, and at a light intensity of 1000 μmol photons m-2 s-1. As described by Loreto et al. ([10]), mitochondrial respiration in the light was directly measured monitoring the12 CO2 emission from illuminated leaves exposed to air containing13 CO2 using a LI-800 CO2 analyser (Li-Cor, Lincoln, NE, USA) which has a low sensitivity to13 CO2. In other experiments, CO2 and light intensity were varied to investigate the responses to these two parameters of the leaves of the three classes.
Measurements were carried out in the morning (10.00 - 12.00 h) to avoid variations caused by daily trends of photosynthesis and isoprene emission in response to physiological (e.g., starch accumulation) or environmental (e.g., light, temperature) factors.
Isoprene emission measurements
Isoprene emission was sampled when other gas exchange parameters were stable by diverting a part of the air exiting the gas-exchange cuvette system, as explained by Scholefield et al. ([15]).
This flow was diverted into a PTR-MS (Proton Transfer Reaction Mass Spectrometer, Ionicon, Innsbruck, Austria) which allowed real-time detection (with response times less than 100ms) of isoprene, avoiding the need to specific sample preparation before injection into the inlet. This technique also allows very low fragmentation and high detection sensitivity ([7]). Validation of isoprene measurements by PTR-MS was performed using an isoprene certified standard (70 ppb) previously quantified by GC (Syntech Spectras, Groeningen, The Netherlands).
Statistical analysis
The experiments were repeated on at least five different leaves of different plants. Means and standard deviations are presented. ANOVA was first used to test significance of differences between treatments (CO2 levels). Since these treatments were not statistically significant, ANOVA was again used to separate means of the three leaf classes (t-test, P < 0.05 or 0.10).
Results
After a 30-d long growth in our experimental system, leaves of class C (those leaves expanded ex novo inside the bags) showed photosynthetic rates lower than in leaves of class A (newly expanding outside the cuvette) and B (already expanded inside the cuvette at the beginning of the treatment). This effect was observed both when measuring photosynthesis at ambient (Fig. 1a) or elevated CO2 (Fig. 1b). However, exposure to elevated CO2 in the bag did not influence per se photosynthesis, as indicated by the non significantly different photosynthetic rates observed on leaves of each class developing from bags exposed at ambient or elevated CO2.
Fig. 1 - Photosynthetic rate measured at 380 ppm CO2 (A) or at 900 ppm CO2 (B). Other experimental conditions as defined in the text. Black and grey bars represent leaves grown at 380 and 900 ppm, respectively. The three leaf classes are identified by capital letters: A = leaves developing at ambient CO2 (380 ppm) above the enclosures; B = leaves already developed inside the enclosure at the beginning of the treatment; C = leaves developing inside the enclosure during the treatment (ontogenetically similar to A leaves). Means + standard deviations (n = 5) is shown. Treatment (CO2 levels) means were not statistically different (t-test). Differences between means of the three leaf classes (t-test) are reported with different letters (single letter, P < 0.05, double letters P < 0.10).
The stomatal conductance of class C leaves was also lower than in the other two leaf classes, when assayed both at ambient (Fig. 2a) or elevated CO2 (Fig. 2b). As for photosynthesis, the stomatal conductance of leaves exposed to elevated CO2 in the bags was not further reduced with respect to leaves exposed to ambient CO2.
Fig. 2 - Stomatal conductance measured at 380 ppm CO2 (A) or at 900 ppm CO2 (B). Other experimental conditions as defined in the text. Bar assignment, leaf class assignment and statistical analysis as shown in Fig. 1 legend.
However, analysis of the response of photosynthesis to different CO2 and light levels, identified limitations different than at stomatal level. In particular, the slope of the CO2 response of C leaf photosynthetic rates was much lower than in B leaves, independently of the CO2 concentration experienced by the leaves during growth (Fig. 3). The leaves of class A showed rates intermediate between those of the two classes grown in the bags.
Fig. 3 - Relationship between photosynthesis and intercellular CO2 concentration in leaves developing at ambient CO2 (380 ppm) above the enclosures (class A leaves = circles); in leaves developing inside the enclosure during the treatment (ontogenetically similar to A leaves) (class C leaves = squares); and in leaves already developed inside the enclosure at the beginning of the treatment (class B leaves = triangles). The mean of 10 measurements are shown, with standard deviations always < 10% of the reported means. The measurements refer to both CO2 treatments (380 or 900 ppm CO2 in the enclosures) since the two CO2 levels did not cause significant differences in the CO2 response of photosynthesis.
Similar to the CO2 response, leaves of class C and B showed the lowest and highest rates of photosynthesis (respectively) at high light intensity (Fig. 4). The light response of photosynthesis was similar in the leaves grown at ambient or elevated CO2, and was similar in the three leaf classes in the range of linear response (at low light), denoting no large differences in the efficiency of light use. However, B leaves showed the best response of photosynthesis at thigh light intensity, again suggesting that when light is not a limiting factor the biochemistry of these leaves performed much better than in the other leaf classes of this study.
Fig. 4 - Relationship between photosynthesis and light intensity in leaves developing at ambient CO2 (380 ppm) above the enclosures (class A leaves = circles); in leaves developing inside the enclosure during the treatment (ontogenetically similar to A leaves) (class C leaves = squares); and in leaves already developed inside the enclosure at the beginning of the treatment (class B leaves = triangles). The mean of 10 measurements are shown, with standard deviations always < 10% of the reported means. The measurements refer to both CO2 treatments (380 or 900 ppm CO2 in the enclosures) since the two CO2 levels did not cause significant differences in the light response of photosynthesis.
The mitochondrial respiration measured in the dark (Fig. 5a) and in the light (Fig. 5b) also followed the same trend observed for photosynthesis and stomatal conductance, being lowest in C leaves and highest in B leaves. However, due to the high error of these measurements, the differences were not statistically significant. The respiration rates were not affected by the CO2 treatment, being similar in leaves grown at ambient or elevated CO2.
Fig. 5 - Mitochondrial respiration measured in dark conditions (Rn - panel A) and in the light (Rd, 1000 μmol m-2 s-1 of light intensity - panel B). Other experimental conditions as defined in the text. Bar assignment, leaf class assignment, and statistical analysis as shown in Fig. 1 legend.
Isoprene emission was significantly reduced in C leaves in comparison to B leaves, with A leaves showing intermediate rates of emission (Fig. 6). As in all other measurements, this effect was not attributable to the CO2 concentration in the bags, as the rates were similar in leaves grown at ambient or elevated CO2.
Fig. 6 - Isoprene emission by leaves developing at ambient CO2 (380 ppm) above the enclosures (A class); leaves developing inside the enclosure during the treatment (ontogenetically similar to A leaves) (C class); and leaves already developed inside the enclosure at the beginning of the treatment (B class). Measurements were conducted at a CO2 concentration of 380 ppm, other conditions as detailed in the text. Statistical analysis as shown in Fig. 1 legend.
Discussion
Research generally indicate that elevated CO2 positively affects photosynthesis, especially when exposure is limited to short periods (minutes to days - [20]). Surprisingly, we did not observe such an effect in our experiment. Elevated CO2 should also affect negatively stomatal conductance. The inverse effects on photosynthesis and stomatal conductance generally improve the water use efficiency of plants exposed or grown at elevated CO2 ([2]). Again, this was not observed in our experiment. Since the experiment did not yield differences in the physiological parameters of leaves exposed to the treatment, we were not surprised to observe no difference in these parameters also in leaves developing outside the bags (class A leaves), independently on their development from enclosures exposed to ambient or elevated CO2.
We speculate that the effect of CO2 was somehow reduced or counteracted by a simultaneous, strong, effect of the enclosures. We have recorded, during bright, sunny days, temperatures up to 2°C warmer and a 25% light attenuation inside the bags with respect to outside. The combined effect of these two variants might have decreased photosynthesis, favoring photorespiration instead. Interestingly, however, bag enclosures caused an opposite effect on the gas exchange of leaves depending on their ontogeny. In leaves already expanded (class B), the exchange of CO2, water and isoprene was higher than in leaves developing outside the bags. In new leaves developing during the experiment in the bags (class C) all gas-exchanges were down-regulated with respect to the other leaf classes.
The stomata of C leaves were less open than in the other two classes of leaves. However, we do not believe that this may have limited photosynthesis in C leaves since the calculated intercellular CO2 concentration was similar in all leaf classes (180 + 20 ppm, data not shown). Rather, the activity of ribulose 1-5 bisphosphate carboxylase (Rubisco) was reduced in C leaves. This was clearly indicated by the slope of the relationship between photosynthesis and intercellular CO2 concentration ([3]), which was lower in C leaves than in the other leaf types. Consistent with other gas-exchange measurements, B leaves showed the steeper slope and, consequently, the highest Rubisco activity among the different classes. B leaves also showed a clearly larger photosynthetic rate at high light intensity with respect to the other leaf classes, denoting again a better use of CO2 (i.e., a more efficient biochemistry) under non-limiting light intensity. It remains to be understood what has caused the chain of biochemical adjustment in turn leading to contrasting physiological response in leaf developing and developed inside the enclosure. The different leaf age may be invoked as the main factor driving contrasting responses of leaf class B and C to the enclosure. However, it is interesting to note that leaves of class A and C leaves were of similar age, both expanding during the experiment outside (class A) or inside (class C) the enclosures. This suggest no effect of leaf ontogeny on the reported differences, although it remains to be tested by using other markers (e.g., anatomical), whether leaves expanding in the enclosures developed more slowly than those growing outside the enclosures.
The main objective of our study was the investigation of the impact of elevated CO2 on isoprene emission from leaves directly exposed to the enrichment or developing at ambient CO2 but from leaves exposed to elevated CO2. Isoprene is formed predominantly from photosynthetic carbon fixation ([19]) and it was expected that the two processes be simultaneously stimulated by increasing availability of CO2. However, exposure to or growth at elevated CO2 often reduce isoprenoid emission by vegetation ([8], [9], [15], [14]), with few exceptions (e.g., [16], [12]). This uncoupling between the two processes may be due to an inhibition of isoprene synthase activity under elevated CO2 ([15]) or to a reduction of the availability of isoprene synthase substrate (predominantly dimethylallyl diphosphate (DMADP), [14]). According to Rosenstiel et al. ([14]) DMADP shortage could be due to the competition of mitochondrial respiration for the same substrate. Larger rates of mitochondrial respiration in fact require an increased conversion of phosphoenolpyruvate (PEP) to pyruvate under elevated CO2. Therefore, elevated CO2 may vary isoprene emission by altering the partitioning of PEP between mitochondrial and chloroplastic process. In our experiment, however, elevated CO2 did not cause any decrease of isoprene emission, either in leaves developed or developing at elevated CO2 (class B and C), or in leaves developing at ambient CO2 (class A). The emission rates of isoprene of the three leaf classes were associated to photosynthesis, suggesting that the rates of photosynthetic carbon fixation drive, and control, isoprene synthesis in all conditions. Thus, the effect of elevated CO2 per se on isoprene emission remains ambiguous, and it may be possible that future environmental conditions will not down-regulate isoprene emission by vegetation as speculated by other authors ([14]). Contrary to our expectations, isoprene emission rates were also associated to the rates of mitochondrial respiration. We performed measurements of the respiration in the light, under the ground that these are the actual rates when isoprene biosynthesis occurs, and may be lower than in the dark ([10]). However, also the rates of mitochondrial respiration in the light were closely associated to isoprene emission, which does not support the hypothesis that pyruvate requirement by respiration competes with isoprene and controls isoprene emission, especially at elevated CO2.
It is interesting to observe that many experiments in which the inhibition of isoprene by elevated CO2 was not observed, were based on branch enclosure long-term measurements (e.g., [13]). We therefore also put forward the suggestion that branch enclosures strongly and independently affect many physiological features, and do not offer representative indications of the actual impact of elevated CO2 on primary and secondary carbon metabolism in nature.
In summary, our experiment shows that growth at elevated CO2 may not perturb primary carbon exchange by photosynthesis and respiration and emission of carbon as secondary metabolite (isoprene) in either developed or developing leaves, or in leaves expanding at ambient CO2 above those grown at elevated CO2, at least when only a part of the plant is exposed to elevated CO2 in enclosures. The experiment suggests, however, that the enclosure system may have a profound effect on primary and secondary metabolism, negatively or positively altering the rates of gas-exchange in developed and developing leaves, respectively. This should be considered in further experiments based on enclosure systems.
Acknowledgments
We acknowledge funding by the European Science Foundation programme Volatile Organic Compounds in the Biosphere-Atmosphere System (VOCBAS), and by the Italian Ministry for Research, projects FIRB - RBAU018FWP: “Attività antiossidante degli isoprenoidi volatili e loro ruolo nella protezione delle piante dagli stress abiotici”, and PRIN - “Valutazione di rischio di livello II da esposizione ad ozono per la vegetazione mediterranea. Analisi sperimentali in condizioni controllate di laboratorio e in campo, misure e modellistica dei flussi di ozono”.
References
CrossRef | Gscholar
Gscholar
CrossRef | Gscholar
Authors’ Info
Authors’ Affiliation
Domenico Tricoli
Silvano Fares
Francesco Loreto
CNR - Istituto di Biologia Agroambientale e Forestale, v. Salaria Km. 29.300, I-00016 Monterotondo Scalo, RM (Italy)
CNR - Istituto sull’Inquinamento Atmosferico, v. Salaria Km. 29.300, I-00016 Monterotondo Scalo, RM (Italy)
Corresponding author
Paper Info
Citation
Brilli F, Tricoli D, Fares S, Centritto M, Loreto F (2008). The use of branch enclosures to assess direct and indirect effects of elevated CO2 on photosynthesis, respiration and isoprene emission of Populus alba leaves. iForest 1: 49-54. - doi: 10.3832/ifor0429-0010049
Paper history
Received: Mar 09, 2006
Accepted: Jan 01, 2007
First online: Feb 28, 2008
Publication Date: Feb 28, 2008
Publication Time: 14.10 months
Copyright Information
© SISEF - The Italian Society of Silviculture and Forest Ecology 2008
Open Access
This article is distributed under the terms of the Creative Commons Attribution-Non Commercial 4.0 International (https://creativecommons.org/licenses/by-nc/4.0/), which permits unrestricted use, distribution, and reproduction in any medium, provided you give appropriate credit to the original author(s) and the source, provide a link to the Creative Commons license, and indicate if changes were made.
Web Metrics
Breakdown by View Type
Article Usage
Total Article Views: 55454
(from publication date up to now)
Breakdown by View Type
HTML Page Views: 45428
Abstract Page Views: 3260
PDF Downloads: 5662
Citation/Reference Downloads: 52
XML Downloads: 1052
Web Metrics
Days since publication: 6333
Overall contacts: 55454
Avg. contacts per week: 61.29
Article Citations
Article citations are based on data periodically collected from the Clarivate Web of Science web site
(last update: Mar 2025)
Total number of cites (since 2008): 2
Average cites per year: 0.11
Publication Metrics
by Dimensions ©
Articles citing this article
List of the papers citing this article based on CrossRef Cited-by.
Related Contents
iForest Similar Articles
Research Articles
A new approach to ozone plant fumigation: The Web-O3-Fumigation. Isoprene response to a gradient of ozone stress in leaves of Quercus pubescens
vol. 1, pp. 22-26 (online: 28 February 2008)
Research Articles
Photosynthesis of three evergreen broad-leaved tree species, Castanopsis sieboldii, Quercus glauca, and Q. myrsinaefolia, under elevated ozone
vol. 11, pp. 360-366 (online: 04 May 2018)
Research Articles
Influence of inorganic salts on biomass production, biochemical composition, and bioethanol production of Populus alba
vol. 13, pp. 566-574 (online: 07 December 2020)
Research Articles
Links between phenology and ecophysiology in a European beech forest
vol. 8, pp. 438-447 (online: 15 December 2014)
Research Articles
Species-specific morphological and physiological characteristics and progressive nitrogen limitation under elevated CO2 concentration
vol. 13, pp. 270-278 (online: 03 July 2020)
Research Articles
Response of juvenile progeny of seven forest tree species and their populations to simulated climate change-related stressors, heat, elevated humidity and drought
vol. 11, pp. 374-388 (online: 15 May 2018)
Short Communications
Variation in growth, photosynthesis and water-soluble polysaccharide of Cyclocarya paliurus under different light regimes
vol. 10, pp. 468-474 (online: 04 April 2017)
Research Articles
Adaptability of Indocalamus decorus to climate change based on physiological and biochemical responses to elevated carbon dioxide and ozone
vol. 9, pp. 311-317 (online: 22 October 2015)
Short Communications
Stomata morphological traits in two different genotypes of Populus nigra L.
vol. 8, pp. 547-551 (online: 16 September 2014)
Review Papers
Leaf volatile isoprenoids: an important defensive armament in forest tree species
vol. 5, pp. 13-17 (online: 14 February 2012)
iForest Database Search
Search By Author
Search By Keyword
Google Scholar Search
Citing Articles
Search By Author
Search By Keywords
PubMed Search
Search By Author
Search By Keyword