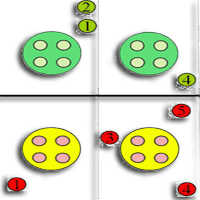
Changes in the proteome of juvenile European beech following three years exposure to free-air elevated ozone
iForest - Biogeosciences and Forestry, Volume 4, Issue 2, Pages 69-76 (2011)
doi: https://doi.org/10.3832/ifor0570-004
Published: Apr 05, 2011 - Copyright © 2011 SISEF
Research Articles
Collection/Special Issue: IUFRO RG 7.01 2010 - Antalya (Turkey)
Adaptation of Forest Ecosystems to Air Pollution and Climate Change
Guest Editors: Elena Paoletti, Yusuf Serengil
Abstract
Tropospheric ozone, one of the most phytotoxic air pollutants, may specially impose in long-lived forest trees substantial reduction in productivity and biomass. European beech saplings grown in lysimeter around areas were used to monitor proteomic changes upon elevated ozone concentrations following four vegetation periods of exposure. A proteome study based on highly sensitive two-dimensional fluorescence difference gel electrophoresis (2-D DIGE) was performed to identify protein changes in European beech, the most important deciduous tree in Central Europe. Main emphasis was on identifying differentially expressed proteins after long-time period of ozone exposure under natural conditions rather than short-term responses or reactions under controlled conditions. Our results clearly demonstrate a response of European beech saplings to long-term ozone fumigation at the protein level. We indicate changes in the protein abundance of 142 protein spots; among them 59 were increased and 83 decreased following three years of elevated ozone exposure. As the first step, 40 proteins were identified by a homology driven mass spectrometric approach. Some of the identified proteins have been previously described in the context of short-term ozone responses in plants, indicating, at least for certain cellular functions, the congruence of plant reactions following short- and long-term ozone exposure. Under elevated ozone exposure, abundance of proteins related to the Calvin cycle and photosynthetic electron transport chain were decreased whereas the abundance of proteins regarding the carbon metabolism/catabolism were increased.
Keywords
Abiotic stress, Elevated ozone, European beech, Woody plants, 2-D DIGE
Introduction
Tropospheric ozone, as an indirectly emitted greenhouse gas, is present in low concentrations, but minor changes in its abundance seem to have strong influences on living organisms. Mainly due to anthropogenic pollutants, such as those from biomass and fossil fuel burning, ground levels of ozone concentrations have more than doubled in the past 100 years ([17], [24]). Due to its powerful oxidizing properties and consequently its capability of damaging organic molecules, ozone has been well known to be detrimental for living organisms. In plants, ozone enters through the stomata, triggering a reactive oxygen species reaction in the apoplast of leaves ([35], [29]). The type and severity of injury can vary depending on concentration, weather conditions, length of exposure and genetical predisposition of plants.
Exposure to ozone has been linked to a number of altering effects in plants, including reduced growth, lower yields, accelerated senescence and necrosis in leaf tissue ([25],[28], [29]). At the physiological and biochemical level ozone has been shown to decline gas exchange ([20]) and to inhibit carboxylation efficiency and net photosynthesis ([11], [1], [6], [34]). Different studies have also shown an induction of glucose catabolism, mitochondrial respiration as well as activation of detoxification and reparation processes ([6], [15]). For the same material as in this experiment it was recently shown at the transcript level that several expressed sequence tags (ESTs) involved in cell structure, related to stress response and cell walls, signal transduction, as well as disease and defence were induced ([27]).
During the last years the use of proteomics in plant biology research has increased significantly ([18]), however, there is still very little information available in terms of proteomic analysis after long-term responses to ozone and even less for field experiments in woody plants upon this hazardous oxidant. Although seven previous proteomic studies in plant species have provided valuable insights into ozone-related protein expression, those reports have focused on short-time periods of ozone exposure under controlled conditions ([1], [6], [38], [8], [13], [2], [36]). The overall aim of this investigation was to study long-time effects of elevated ozone under natural conditions in European beech trees, the most important deciduous tree species in Central Europe.
Here we present for the first time a proteomic approach using highly sensitive two-dimensional fluorescence difference gel electrophoresis (2-D DIGE) coupled with a homology driven mass spectrometric approach in order to identify local changes in leaf proteins from European beech saplings following three years ozone fumigation, covering two entire and two partial vegetation periods. Furthermore we used complementary transcript results performed on the same study ([27]) as well as starch and sucrose results ([14]) from beech saplings grown in lysimeters of this experiment, in order to better understand ozone related responses on the molecular level.
Material and methods
Experimental design
The experimental design of the lysimeter and its surrounding area including the free-air ozone exposure device was previously described ([37], [30], [40]). In brief, forest soil from the forest site “Högelwald” was filled in and around lysimeters in 1999. For the subsequent three years soil was left untreated to ensure the development of a typical soil structure. In November 2002, three-year-old nursery grown European beech saplings were planted in and around lysimeters. Ambient and twice ambient ozone fumigation started in July 2003 and ended after four vegetation periods in August 2006. Ozone was fumigated during the day period and stopped for the first vegetation period in December 2003. For the year 2004 and 2005, ozone was fumigated from May (before bud break) until end of October (after leaf senescence). During the last experimental year saplings were fumigated from May until end of August. Ozone fumigation was restricted to concentrations lower than 150 nl l-1, in order to avoid acute injury of leaves. During the harvesting year of 2006 the ozone concentration resulted in an AOT 40 value of 52.6 μl l-1 h. Details about the ozone concentration are given by Winkler et al. ([40]). Ten trees from the lysimeter around area were used as biological replicates for each group category (ambient and twice ambient ozone defined respectively as control and treatment group - Fig. 1). From each tree, three sun exposed crown leaves were taken on 27th of July 2006. Leaves from one tree were combined and immediately frozen in liquid nitrogen. At the harvesting time point, beech saplings were seven years old. Fig. 2 gives an overview of the experimental setup.
Fig. 1 - Schematic drawing of the experimental site. Lysimeters are represented by light blue circles. Green and red circles represent beech saplings fumigated with respectively ambient and twice ambient ozone. Numbered circles show the position of the used beech saplings in the experiment.
Fig. 2 - Schematic diagram of the experimental design in the time scale. Proteomic analyses were performed for the time point 27th July 2006.
Protein extraction
Each biological sample consisted of a pool of three leaves from one tree. Samples were ground to a fine powder using a micro dismembrator (BraunTM) without interruption of the cooling chain. Soluble proteins were extracted using 100 mg of fresh weight material according to the protocol of Valcu & Schlink ([39]) with some modifications. In brief, proteins were precipitated over night at -20°C in 10% TCA, 1% polyvinyl polypyrrolidone (PVPP), 0.07% 2-mercaptoethanol in ice cold acetone ([10]). Pellet was centrifuged at 26 000 x g for 30 min at 4 °C. Supernatant was removed and the remaining pellet was washed in ice cold acetone with 0.07% 2-mercaptoethanol for one hour at -20°C. The washing procedure was repeated once. Finally the pellet was centrifuged (26 000 x g, 30 min, 4 °C), dried for 1 h on ice, resuspended in 1 ml extraction buffer (7 M urea, 2 M thiourea, 2% Octyl-β-D-glucopyranoside, 40 mM TRIS) and sonicated for 30 min in a water-bath sonicator, at 4-7 °C. The extract was centrifuged at 4 °C and the pH was adjusted to 8.5 using 100 mM NaOH. Total protein amount from each sample was measured based on a modified Lowry test using the RC-DCTM Protein Assay kit BioRadTM and BSA as standard to generate a regression line.
Two-dimensional gel electrophoresis
Prior separation 50 µg of each sample and internal standard were labeled with 200 pmol of CyDyes diluted in N,N-dimethylformamide. A randomized sample labeling with Cy3 and Cy5 dyes was used in order to avoid systemic errors introduced during the labeling reaction. Cy2 was used to label the internal standard that consisted of equal amounts of all protein extracts from control and treatment samples within the experiment. A total of ten 2-D gels, each consisting of an internal standard, a control and a treatment sample, were used to separate proteins. For the isoelectric focusing (IEF), 24 cm and 18 cm strips were used with a linear pI gradient ranging from 4-7 and 6-11 (GE Healthcare). CyDyes labelled samples (a total of 150 µg) were mixed in rehydration solution (7 M urea, 2 M thiourea, 2 mM 2-hydroxyethyl disulfide, 2% Octyl-β-D-glucopyranoside, 0.5% Pharmalyte 3-10, 0.002% bromophenol blue and further 10% isopropanol for basic gradients) and loaded onto IPG DryStrips using in-gel sample rehydration technique for gels with pI 4-7. Proteins separated in gels pI 6-11 were cup loaded prior to IEF. After rehydration for 12 hours IEF was carried out with a maximum current setting of 50 mA/strip at 20 °C on an Ettan IPGphor Manifold (GE Healthcare). The system was programmed for strips pI 4-7 as follows: 150 V for 6 h,150-300 V for 4 h, 300-1000 V for 11.25 h, 1000-8000 V for 3 h and 8000 V for 5 h. For strips pI 6-11 following settings were used: 150 V for 5 h, 300 V for 3 h, 300-1000 V for 6 h, 1000-8000 V for 3 h and 8000 V for 1.5 h. After IEF each strip was equilibrated with 10 mL of equilibration solution (6 M urea, 75 mM Tris-HCl pH 8.8, 30% glycerol, 2% SDS, 0.002% bromophenol blue). Equilibration was performed in two steps each 15 min, with 1% w/v dithiothreitol in the first equilibration, and 2.5% w/v iodoacetamide in the second equilibration. SDS-PAGE was carried out on an Ettan DALT six system (GE Healthcare) in 12.5%, 1-mm-thick polyacrylamide gels. Electrophoretical conditions were applied as follows: 30 mA for 1 h, 48 mA for 1 h and 98 mA over night.
Protein visualization
Gels were scanned using a Typhoon 9410 imager (GE Healthcare) at 100 μm resolution. Cy2, Cy3 and Cy5 dyes were respectively excited at 488, 532 and 633 nm.
Raw gel images were aligned with Progenesis SameSpots® software (Nonlinear Dynamics) using a master gel as reference. Virtually wrapped gels were imported in DeCyder® 6.5 software (GE Healthcare) for further 2-DE spot identification, normalization and quantification.
Statistical analysis
The low separation and labeling quality of proteins from gel 7 resulted in the exclusion of samples from tree number 7 of both groups. A total of 27 gel images (9 controls, 9 treated and 9 internal standards) were used for statistical analysis. The abundance of each protein was estimated by the volumes (sum of pixel intensity within the spot boundary). Differences in spot intensity between groups were compared using the Student´s t-test function implemented into DeCyder. Based on the p values, q values were calculated according to Benjamini & Hochberg ([4]) in order to rejected false positives. Only protein abundances showing significances lower than (q ≤ 0.05) and an absolute ratio of at least 1.3 fold were used for protein identification.
Protein digestion and identification by liquid chromatographic-tandem mass spectrometry (LC MS/MS)
Preparative gels loading 700-800 µg total protein amount were run and stained with colloidal Coomassie G-250 (CBB) according to Candiano et al. ([7]). Low abundant protein spots were visualized using 150-250 µg total protein amount and silver stained with a MS compatible method ([16]), without using formaldehyde and glutaraldehyde in the sensitizing and silver solution, respectively). Selected spots were manually excised using a scalpel. CBB extracted spots were then destained in 40% ethanol/50 mM ammonium bicarbonate ultra pure. Silver stained spots were destained in a 2% potassium hexacyanoferrate/3.2% sodium thiosulphate 1:1 mixture and washed five times with water. Spots were dehydrated with acetonitrile (ACN) and dried in a vacuum centrifuge. Gel pieces were rehydrated in 10 ng/µL trypsin solution (Sigma-Aldrich) in 50 mM ammonium bicarbonate and incubated over night at 37 °C. Hydrophilic peptides were extracted with 40 mM ammonium bicarbonate 10% ACN at room temperature for 10 min. Hydrophobic peptides were extracted with 47% v/v ACN, 5% v/v formic acid and the extraction step was repeated twice. All three supernatants were pooled together, concentrated in a vacuum centrifuge, acidified with formic acid and stored at -20 °C.
Peptide mixtures were analyzed by on-line capillary nano HPLC (LC Packings, Amsterdam, Netherlands) coupled to a nanospray LCQ Deca XP ion trap mass spectrometer (ThermoFinnigan, San Jose, CA, USA). Peptide digest (10 µL) were loaded onto 300 µm inner diameter x 5 mm C18 PepMap™ trap column (LC Packings, Amsterdam, Netherlands) at a flow rate of 30 µl/min. The peptides were eluted from the trap column onto an analytical 75 µm inner diameter x 15 cm C18 PepMap™ column (LC Packings, Amsterdam, Netherlands) with a 5-40% linear gradient of solvent B in 30 min (solvent A was 0.1% formic acid in 5% ACN, and solvent B was 0.1% formic acid in 80% ACN). The separation flow rate was set at 200 nl/min. The mass spectrometer operated in positive ion mode at a 1.8 kV needle voltage and a 28 V capillary voltage. Data acquisition was performed in a data-dependent mode by alternating a MS scan survey over the range m/z 300-1700 and three MS/MS scans in an exclusion dynamic mode. MS/MS spectra were acquired using a 2 m/z units ion isolation window at 35% relative collision energy and 0.5 min dynamic exclusion duration. Peptides were identified with SEQUEST™ algorithm through Bioworks 3.3.1 interface (Thermo-Finnigan, Torrence, CA, USA) against a Fagus contigs consensus database (293 388 entries from ⇒ http://www.evoltree.com/) translated in 6 reading frames by Transeq software (⇒ http://www.cbib.u-bordeaux2.fr/pise/transeq.html). The generation of mass spectra data in SEQUEST™ format allowed the averaging of several MS/MS spectra corresponding to the same precursor ion with a tolerance of 1.4 Da. Spectra from precursor ion higher than 3500 Da or lower than 600 Da were rejected. The search parameters were as follows: mass accuracy of the peptide precursor and peptide fragments was set to 2 and 1 Da, respectively. Only b- and y-ions were considered for mass calculation. Oxidation of methionines (+16 Da) and carbamidomethylation of cysteines (+57 Da) were considered as differential modifications. Two missed trypsin cleavages were allowed. Tryptic peptides were validated using the following criteria (DeltaCN ≥ 0.1, Xcorr ≥ 1.9 (single charge), 2.2 (double charge), 3.75 (triple charge), 3.0 (quadruple charge), peptide probability ≤ 0.001. EST annotations were identified by searching with a protein Viridiplantae index from Swiss-Prot (BLASTX) and TrEMBL (BLASTX) database using UniProtKB (⇒ http://www.uniprot.org/).
Results and discussion
In the present study we analyzed ozone-related responses of European beech leaves following four vegetation periods of ozone fumigation under field conditions. Visible foliar symptoms represented by leaf discoloured areas were recorded for five time points on beech saplings grown inside lysimeters (n=16 trees/group - Grams, pers. comm.). Results did not show any visually symptom on foliar injury following ozone exposure for the harvesting time point of the proteomic approach (27th of July).
Ozone responsive proteins detected by 2-D DIGE
After image analysis of 2-D gels we could observe on average a total amount of 1350 spots in both pI ranges (pI 4-7 and pI 6-11). Our results showed that 9% of the total protein spots were differentially regulated on abundance after elevated ozone fumigation. The image analysis revealed that in the pI range 4-7, 114 spots and in the pI range 6-11, 28 spots showed different protein abundances by comparing samples fumigated with twice ambient ozone versus the control treatment (Fig. 3). From the total of 142 spots showing a significant different spot volume, 88 spots could be visualized in agreement with the preparative silver stained gels. As a first step, 46 protein spots were subjected to mass spectrometry identification (LC-MS/ MS) followed by a homology driven search. The mass spectra of 6 spots failed to show any peak while 27 spots resulted in multiple protein mixtures. As for the remaining 13 spots, proteins were identified (Tab. 1), which are particularly involved in the Calvin cycle, photosynthetic electron transport chain and carbon metabolism/catabolism.
Fig. 3 - DIGE labelled 2-D gels of separated soluble proteins from European beech leaves. Red marked protein spots indicate changes in their regulation after statistical analysis. Zoomed squares exemplify patterns of protein regulation. Spots labeled with triangles area visualized as 3-D graph in the area below.
Tab. 1 - List of identified proteins by LC-MS/MS. (a) Number of protein spot on the 2-D gel; (b) Accession number from the best homologous protein in Swiss-Prot and/or TrEMBL database; (c) Q-value calculated by treatment interaction of control versus treated samples. Values were calculated based on of the Student’s t-test after applying a false discovery rate according to Benjamini & Hochberg ([4]); (d) Average ratio calculated from the normalized protein volumes. Negative values are given as negative reciprocal value (average ratio=-1/average); (e) Normalized average spot volumes (sum of pixel intensity within the spot boundary) for control (Cont) and treated (Treat) groups; (f) Standard deviation from protein spot volumes of the controls (Cont) and treated (Treat) samples; (g) Experimental Mw (in Da) and pI. Data were estimated in comparison to a 2-D gel with marker proteins; (h) Theoretical Mw (in kDa) computed by the expasy website (⇒ http://us.expasy.org/tools/peptide-mass.html); (i) Number of matching peptides. See ⇒ http://www.sisef.it/iforest/pdf/Kerner_570@suppl01.xls for peptides sequence; (j) Ratio of the amino acids in detected peptides to total protein amino acids: coverage. Values are given in %; (k) Location of identified proteins: chloroplast (chloropl.), mitochondrion (mitoch.), plasma membrane (pl. memb.).
Spot number(a) | Accession number(b) | Identified protein | Q-value(c) | Average ratio(d) | Volume(e) | Standard deviation(f) |
Exp.(g) | Theo.(h) | Data from the SEQUEST search | Compart.(k) | |||||
---|---|---|---|---|---|---|---|---|---|---|---|---|---|---|---|
Cont | Treat | Cont | Treat | pI | Mw | pI | Mw | M(i) | Cov.(j) | ||||||
Calvin Cycle photosynthesis related proteins | |||||||||||||||
613 | Q43848 | Transketolase | 0.0054 | ↓-2.13 | 13.1 | 6.16 | 5.64 | 3.24 | 6.16 | 88 | 5.53 | 72.93 | 3 | 3.97 | chloropl. |
1389 | Q42961 | Phosphoglycerate kinase | 0.0040 | ↓-2.09 | 9.02 | 4.21 | 3.37 | 2.14 | 6.19 | 55 | 5.59 | 42.58 | 2 | 7.58 | chloropl. |
1353 | Q40281 | RuBisCO activase | 0.0024 | ↓-1.89 | 11.75 | 6.27 | 5.04 | 2.78 | 5.08 | 56 | 8.2 | 48.07 | 3 | 8.49 | chloropl. |
408 | Q9ZU52 | Probable frutctose-bisphosphate aldolase 3 | 0.0091 | ↓-1.81 | 67.51 | 36.36 | 2.96 | 1.56 | 7.12 | 40 | 6.08 | 38.08 | 4 | 10.75 | chloropl. |
621 | P27141 | Carbonic anhydrase | 0.0043 | ↓-1.61 | 215.32 | 154.59 | 6.87 | 4.88 | 6.79 | 28 | 6.19 | 23.96 | 1 | 3.6 | chloropl. |
1463 | P16096 | Fructose-bisphosphate aldolase | 0.0001 | ↓-1.55 | 27.49 | 17.6 | 7.65 | 4.5 | 6.51 | 53 | 5.8 | 37.7 | 4 | 7.19 | chloropl. |
1428 | P46283 | Sedoheptulose-1.7 bisphosphatase | 0.0077 | ↓-1.55 | 12.27 | 7.72 | 3.49 | 1.67 | 5.16 | 53 | 6.17 | 42.41 | 3 | 10.86 | chloropl. |
1386 | P27774 | Phosphoribulokinase | 0.0021 | ↓-1.53 | 29.72 | 18.74 | 13.99 | 8.43 | 5.33 | 55 | 5.22 | 39.18 | 5 | 10.7 | chloropl. |
490 | Q9ZUC1 | Quinone oxidoreductase-like protein Atlg23740 | 0.0012 | ↓-1.64 | 181.16 | 113.29 | 6.12 | 5.39 | 6.49 | 35 | 8.46 | 40.98 | 7 | 22.4 | chloropl. |
Carbon metabolism/catabolism | |||||||||||||||
1563 | Q9SIDO | Probable fructokinase-1 | 0.0075 | ↑ 1.32 | 1.04 | 1.38 | 0.39 | 0.22 | 5.17 | 49 | 5.31 | 35.28 | 2 | 4.8 | pl. memb. |
2326 | P80499 | Cytochrome c oxidase subunit 5B | 0.0011 | ↑ 1.7 | 1.72 | 2.93 | 0.29 | 0.74 | 4.88 | 18 | 4.96 | 3.1 | 3 | 6.13 | mitoch. |
2288 | P80499 | Cytochrome c oxidase subunit 5B | 0.0006 | ↑ 5.19 | 0.64 | 3.47 | 0.47 | 1.92 | 4.87 | 20 | 4.96 | 3.1 | 1 | 3.68 | mitoch. |
2188 | Q9FT52 | ATP synthase subunit d | 0.0040 | ↑ 1.77 | 1.39 | 2.4 | - | 0.66 | 5.46 | 26 | 5.09 | 19.45 | 2 | 3.8 | mitoch. |
Ozone exposure affects proteins related to the Calvin cycle and the photosynthetic electron transport chain
The highest number of altered proteins according to a single metabolic pathway was observed in the Calvin cycle, where a total of seven chloroplast proteins were reduced in amount. The enzyme probable ribulose-1.5-bisphosphate carboxylase/oxygenase (RuBisCO) activase (spot 1353) and carbonic anhydrase (spot 621), two enzymes involved in the carboxylation process by regulating the activity of RuBisCO, showed respectively decreased abundance ratios of -1.89 and -1.61 fold compared to the controls. Also the reduction part of the Calvin cycle was affected by decreased abundance levels of phosphoglycerate kinase (spot 1389). Furthermore, phosphoribulokinase (spot 1386), sedoheptulose-1.7-bisphosphatase (spot 1428), two isoforms of probable fructose-bisphosphate aldolase (spot 408/1463) and transketolase (spot 613), enzymes implicated in the regeneration of ribulose-1.5-bisphosphat, were also reduced in their amount. Similar results were observed in other plant species treated with short-term ozone exposure ([8], [13], [2]). In rice seedlings a considerably reduction of both, the large and the small subunit of RuBisCO was shown at the protein level ([1]). Nearly the same results as in this report were found after a short-time period of elevated ozone exposure in poplar, where different proteins regarding the Calvin cycle were reduced in amount ([6]). From this fact it can be deduced that reduced carboxylation may be a general reaction of plants after short- as well as long-time periods of ozone exposure. Although in the present study there was no indication that the amount of RuBisCO present in the leaves was affected after treatment, ozone may directly or indirectly reduce the amount of RuBisCO activase and carbonic anhydrase. As a consequence of decreased CO2 fixation, less enzyme intermediates in the Calvin cycle are needed to process the substrate.
The Calvin cycle activity has been suggested to be a major sink of ATP and nicotinamide adenine dinucleotide phosphate produced during photosynthesis ([21]). Thus, an overall decrease in the activity of the Calvin cycle upon ozone exposure would release an accumulation of both photosynthetic products. In order to prevent photooxidative damage, plants may reduce the electron transport chain of photosynthesis ([31], [6]). Our results support this hypothesis since the enzyme quinone oxidoreductase-like protein (spot 490) responsible for reducing quinone, showed decreased levels. Elevated ozone also decreased gene expression levels of quinone oxidoreductase-like protein in two silver birch genotypes following two growing seasons of ozone exposure ([19]). Furthermore, our finding is in accordance with previous reported transcript analysis from the same plant material which revealed a down-regulation of ESTs related to photosynthesis such as chloroplast envelope quinone-oxidoreductase of electron transport, chlorophyll a/b-binding protein, thioredoxin M-type, glycolate oxidase a and nuclear-encoded chloroplast ribulose-5-phosphate-3-epimerase thioredoxin on the 27th of July 2006 ([27]).
An ozone related down-regulation of enzymes associated with the Calvin cycle suggest that less triose-phosphates are produced during the CO2 fixation process, thus leading to a decreased availability of substrates for energy production ([6]). Initially most triose-phosphates are used in the synthesis of starch and sucrose, the primary photosynthetic products and the most important energy source in plants. Our results indicating a general down-regulation of the Calvin cycle and its product triose-phosphate are in agreement with the reduced levels of sucrose concentrations in leaves exposed to elevated ozone fumigation at any time point of this experiment; also on the 26th of July 2006 ([14]). Similar results were reported in juvenile as well as mature beech leaves exposed to elevated ozone ([22], [5]).
Ozone induced an accumulation of proteins regarding carbon metabolism/catabolism and mitochondrial electron transport chain
In contrast to the Calvin cycle and the photosynthetic apparatus three proteins regarding the carbon metabolism/catabolism showed increased amounts in elevated ozone exposed beech leaves. There is strong evidence that catabolic pathways (e.g., glycolysis, hexose monophosphate pathway, tricarboxylic acid (TCA) cycle and the mitochondrial electron transport system) are up-regulated upon ozone exposure in order to maintain the energy and the reducing power needed to detoxify and repair cellular damage caused by reactive oxygen species ([11], [6], [12], [2]). Responses against cellular damage were observed in the present study. For the time point 27th of July 2006, transcript levels of allene oxide synthase, lipoxygenase, manganese superoxide dismutase, superoxide dismutase Fe and catalase 1 were observed to be increased ([27]). At the proteome level we could identify increased amounts of the enzyme probable fructokinase 1 (spot 1563), a phosphotransferase involved in carbon metabolism/catabolism used for respiration and biosynthesis of starch and other complex carbohydrates ([26]). Based on these results, the reduced levels of starch and sucrose inticated in this experiment ([14]) as well as previous evidences that catabolic pathways are increased upon ozone, we support the hypothesis that the carbon metabolism/catabolism is impaired during ozone stress ([2]). Thus, starch is converted to sucrose which is then degraded by the increased carbon catabolism in order to feed the TCA cycle (Fig. 4).
Fig. 4 - Illustration of possible molecular changes in beech leaves exposed to elevated ozone. Red and green colored transcripts/proteins/metabolites were respectively decreased/increased in amount. Transcripts are shown in italics, whereas metabolites in bold. (CA): carbonic anhydrase; (RuBisCO activase): ribulose-1.5-bisphosphat-carboxylase/-oxygenase activase; (PGK): phosphoglycerate kinase; (PRK): phosphoribulokinase; (SBPase): sedoheptulose-1.7-bisphosphatase; (ALDP): fructose-bisphosphate aldolase; (TKTL): transketolase; (COX subunit 5B): cytochrome c oxidase subunit 5B; (Fru-6-P): fructose 6-phosphate; (Glu-6-P): glucose 6-phosphate; (ATP): Adenosine-5’-triphosphate; (ADP): adenosine diphosphate; (NADPH): reduced nicotinamide adenine dinucleotide phosphate; (NADP+): nicotinamide adenine dinucleotide phosphate (FK 1): probable fructokinase-1.
Further two proteins which are embedded in the mitochondrial electron transport chain, cytochrome c oxidase subunit 5B (spot 2326/2288) and ATP synthase subunit D (spot 2188), were more abundant in treated leaves. In Scots pine needles ozone led to increased enzymatic activities of cytochrome oxidase ([23]). Furthermore, ozone increased mitochondrial respiration rates of aspen clones ([9]), hybrid polar ([32]) and ginkgo biloba ([15]). Cytochrome c oxidase and ATP synthase are mitochondrial enzymes needed for generating an electrochemical potential and production of ATP. Consequently higher amounts of both molecules may indicate an increased respiration. In leaves increased respiration intensity has frequently observed to be linked with simultaneous reduction in photosynthesis ([33]). Furthermore, higher respiration intensity has been suggested to be a response of defense and repair mechanism in ozone damaged tissues ([3]). Our results showing increased amounts of two essential proteins for the ATP production support the hypothesis of an increased mitochondrial respiration.
Concluding remarks
Although our proteome profile represents only a snap-shot of chronic ozone effects in plants grown under field conditions, it confirmed previous reported short-term ozone effects on plant species including poplar, rice and soybean. In general CO2 fixation is reduced showing decreased amounts in proteins related to the Calvin cycle. These results are accompanied by reduced amounts of photosynthetic proteins/transcripts and an overall increase in proteins involved in metabolic/catabolic pathways.
In the present work proteomic analyses, indicated complementary responses to previous reported transcript analysis from the same experiment ([27]) as well as sucrose and starch results of leaves from lysimeter grown beech saplings. Our comprehensive protein analysis will be continued by extending time points and linking analytical data in order to better understand ozone-related effects under a more realistically ecological environment.
Acknowledgements
We are grateful to Christophe Plomion and Stephane Claverol for very helpful comments and substantial organizational support concerning the LC-MS/MS analyses. The authors would also like to thank Sarah Sturm for excellent technical assistance and Edgar Delgado-Eckert for critical reading the manuscript. This research was carried out with financial support from the Deutsche Forschungsgemeinschaft (SFB 607) and, with respect to LC-MS/MS, by the Biodiversité Gènes et Communautés, INRA within the frame of the Network of Excellence “EVOLTREE”.
List of abbreviations
The following abbreviations have been used throughout the text:
- 2-D DIGE: two-dimensional fluorescence difference gel electrophoresis
- ACN: acetonitrile
- AOT 40: accumulated exposure over a threshold of 40 ppb
- ATP: adenosine triphosphate
- BSA: bovine serum albumin
- CBB: colloidal Coomassie G-250
- DCn: delta correlation value
- ESTs: expressed sequence tags
- IEF: isoelectric focusing
- LC-MS/MS: liquid chromatographic- tandem mass spectrometry
- MS/MS: tandem mass spectrometry
- Mw: molecular weight
- NaOH: Sodium hydroxide
- pI: isoelectric point
- RuBisCO: ribulose-1.5-bisphosphate carboxylase/oxygenase
- TCA: tricarboxylic acid cycle
- TRIS: tris(hydroxymethyl)-aminomethan
References
Gscholar
CrossRef | Gscholar
CrossRef | Gscholar
CrossRef | Gscholar
CrossRef | Gscholar
Gscholar
CrossRef | Gscholar
Supplementary Material
Authors’ Info
Authors’ Affiliation
G Müller-Starck
Section of Forest Genetics, Technische Universität München, Hans-Carl-von-Carlowitz-Platz 2, D-85354 Freising (Germany)
M Jürgensen
C Lindermayr
D Ernst
Institute of Biochemical Plant Pathology, Helmholtz Zentrum München, Ingolstädter Landstr. 1, D-85764 Neuherberg (Germany)
Université de Bordeaux, Centre de Génomique Fonctionnelle Bordeaux, Plateforme Protéome, F-33000 Bordeaux (France)
Department of Environmental Engineering, Helmholtz Zentrum München, Ingolstädter Landstr. 1, D-85764 Neuherberg (Germany).
Corresponding author
Paper Info
Citation
Kerner R, Winkler JB, Dupuy JW, Jürgensen M, Lindermayr C, Ernst D, Müller-Starck G (2011). Changes in the proteome of juvenile European beech following three years exposure to free-air elevated ozone. iForest 4: 69-76. - doi: 10.3832/ifor0570-004
Paper history
Received: Sep 13, 2010
Accepted: Jan 17, 2011
First online: Apr 05, 2011
Publication Date: Apr 05, 2011
Publication Time: 2.60 months
Copyright Information
© SISEF - The Italian Society of Silviculture and Forest Ecology 2011
Open Access
This article is distributed under the terms of the Creative Commons Attribution-Non Commercial 4.0 International (https://creativecommons.org/licenses/by-nc/4.0/), which permits unrestricted use, distribution, and reproduction in any medium, provided you give appropriate credit to the original author(s) and the source, provide a link to the Creative Commons license, and indicate if changes were made.
Web Metrics
Breakdown by View Type
Article Usage
Total Article Views: 59857
(from publication date up to now)
Breakdown by View Type
HTML Page Views: 50183
Abstract Page Views: 2828
PDF Downloads: 5180
Citation/Reference Downloads: 72
XML Downloads: 1594
Web Metrics
Days since publication: 4979
Overall contacts: 59857
Avg. contacts per week: 84.15
Article Citations
Article citations are based on data periodically collected from the Clarivate Web of Science web site
(last update: Feb 2023)
Total number of cites (since 2011): 12
Average cites per year: 0.92
Publication Metrics
by Dimensions ©
Articles citing this article
List of the papers citing this article based on CrossRef Cited-by.
Related Contents
iForest Similar Articles
Research Articles
Effects of abiotic stress on gene transcription in European beech: ozone affects ethylene biosynthesis in saplings of Fagus sylvatica L.
vol. 2, pp. 114-118 (online: 10 June 2009)
Research Articles
A comparison between stomatal ozone uptake and AOT40 of deciduous trees in Japan
vol. 4, pp. 128-135 (online: 01 June 2011)
Research Articles
Soil drench of ethylenediurea (EDU) protects sensitive trees from ozone injury
vol. 4, pp. 66-68 (online: 05 April 2011)
Research Articles
Ozone fumigation effects on the morphology and biomass of Norway spruce (Picea abies L.) saplings
vol. 2, pp. 15-18 (online: 21 January 2009)
Research Articles
Prediction of ozone effects on net ecosystem production of Norway spruce forest
vol. 11, pp. 743-750 (online: 15 November 2018)
Research Articles
Ambient ozone phytotoxic potential over the Czech forests as assessed by AOT40
vol. 5, pp. 153-162 (online: 25 June 2012)
Short Communications
Ozone flux modelling for risk assessment: status and research needs
vol. 2, pp. 34-37 (online: 21 January 2009)
Review Papers
Monitoring the effects of air pollution on forest condition in Europe: is crown defoliation an adequate indicator?
vol. 3, pp. 86-88 (online: 15 July 2010)
Research Articles
Long-term monitoring of air pollution effects on selected forest ecosystems in the Bucegi-Piatra Craiului and Retezat Mountains, southern Carpathians (Romania)
vol. 4, pp. 49-60 (online: 05 April 2011)
Research Articles
Sensitivity of European beech trees to unfavorable environmental factors on the edge and outside of their distribution range in northeastern Europe
vol. 9, pp. 259-269 (online: 16 October 2015)
iForest Database Search
Search By Author
Search By Keyword
Google Scholar Search
Citing Articles
Search By Author
Search By Keywords
PubMed Search
Search By Author
Search By Keyword