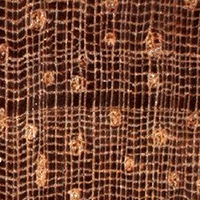
Characterization of technological properties of matá-matá wood (Eschweilera coriacea [DC.] S.A. Mori, E. odora Poepp. [Miers] and E. truncata A.C. Sm.) by Near Infrared Spectroscopy
iForest - Biogeosciences and Forestry, Volume 14, Issue 5, Pages 400-407 (2021)
doi: https://doi.org/10.3832/ifor3748-014
Published: Sep 01, 2021 - Copyright © 2021 SISEF
Research Articles
Abstract
The aim of this study was to determine the technological properties (chemical, mechanical and physical) of Eschweilera sp. woods using near infrared spectroscopy (NIRS). NIR spectroscopy proved to be efficient for chemical analysis (extractives, lignin and carbohydrates) and physical-mechanical testing (moisture content, basic density - BD, modulus of elasticity - MOE and modulus of rupture - MOR) of wood, providing a powerful tool for use in sustainable forest management activities in the Amazon. Wood samples from three trees of each Eschweilera species were collected from the Experimental Station of Tropical Forestry/INPA/Brazil. Specimens were extracted from the cross-sectional area (20 × 20 × 30 mm) in the direction sapwood-heartwood. NIR spectra (4.000-10.000 cm-1) were then obtained from the samples (moisture 12%) using Fourier-transform spectrometry. The Partial Least Squares (PLS) regression prediction models for the chemical, mechanical and physical properties of Amazonian woods were used for quantification. The results for total extractives (both in toluene and ethanol) and hot water solubility showed a maximum extractive concentration of 7.66% and 3.13% for E. odora (yellow matá-matá), including several compounds with low molecular weight, such as resins, gums, terpenes, alkaloids, flavonoids, and tannins. The highest concentration of phenolic substances (tannins) was found in E. Truncata (black matá-matá, 10.00%). The macrocomponents (primary metabolism) of Eschweilera species were in the range of 44.20-46.33% for cellulose and 28.89-31.21% for lignin. Mineral compounds (ash) were quantified in concentrations < 0.70%. The predictive results for the physical and mechanical properties of matá-matá wood are in the standard range for tropical woods. The higher calorific value (HCV) varied from 4.993-5.033 cal g-1 and the BD from 0.78-0.88 g m-3. Regarding moisture, the highest content was observed in E. truncata (13.46%). Values for mechanical resistance were in the range of 14,253-17,447 MPa for MOE and 146.04-175.73 MPa for MOR, with the greatest strength attributed to E. truncata wood. The values obtained for the wood technological properties of E. coriacea (white matá-matá), E. odora (yellow matá-matá) and E. truncata (black matá-matá) were compatible with those obtained by destructive determination of tropical species and also for other species of the genus Eschweilera.
Keywords
Eschweilera, Amazonian Woods, Wood Chemistry, Physico-mechanical Properties, NIR Spectroscopy, Forest Management
Introduction
The Amazon rainforest is considered one of the largest sources of tropical timber in the world, with a production at the end of 2014 of around 31 million m³ from the Brazilian states of Acre, Amazonas, Mato Grosso and Pará ([34]). This scenario would be satisfactory if this production originated from Sustainable Forest Management Programs that combine planned exploitation with legal, economic and ecological principles ([5]). At this level, 6000 botanical species with arboreal habit have already been cataloged; however, the commercial species are limited to a number of 100 ([17]).
Selective exploration in the Amazon of the so-called “commercial woods” is restricted to a dozen species with a large diameter, which dramatically reduces the size of their populations and may generate scarcity of wood provision from these species in the next future. On the other hand, widely abundant species with smaller standard diameter are neglected and/or relegated to the background, as their potential for use and indication for markets is not yet known ([15]). Many of these species are suitable for forest management and are not commercialized due to lack of knowledge of their properties; these include Eschweilera sp. and Couratari stellata (Lecythidaceae), Bixa arborea (Bixaceae), Helicostylis pedunculata (Moraceae), Inga sp. and Tachigali chrysophylla (Fabaceae), among others ([5], [14]).
The timber species known in the Amazon as matá-matá (Eschweilera sp.) are abundant and widely distributed in several regions of the Amazon rainforest, but there are major gaps in our knowledge of their technological characteristics. Understanding the relationship between these characteristics is important to recognize which combination of wood properties is the most suitable for specific uses ([15], [32]).
Technological characterization is fundamental for the indication/use of wood, especially when the intention is to introduce new or little-known species to the market, providing a range of options and adding value to new woods. In this context, assessment of the properties of surface, humidity, density, energy potential, silica content, color, smell, biological protection, etc., provides useful information in order to recommend the best use of this raw material ([50], [13]).
Estimation of wood quality is supported by reliable methodologies, and tools for assessing the properties of wood are constantly evolving, driven by the search for faster, more accurate and lower-cost methods for the study of this material ([25]). Non-destructive assessment (NDA) of wood permits estimation of important technological properties without changing its structure, preserving the material and thus not compromising its use. In most cases, non-destructive methods are based on the investigation of correlations and adjustment of calibration models between the properties of interest and others that are easier to measure, which classes these as indirect methods ([7]).
One of the most powerful, non-destructive tools that has been gaining ground in research and industry for quality control in recent years is near-infrared spectroscopy (NIRS), which makes it possible to correlate the spectra with various properties of wood. This technique is considered robust since it combines mathematical modeling with chemistry (chemometrics), associating the physical, mechanical and biological properties of wood ([50], [48], [27]). It is believed that the NIRS methodology is the primary tool for technological characterization of wood that can be safely and quickly applied to sustainable forest management in the Amazon. In view of this, this study aimed to determine the physical, mechanical and chemical properties of matá-matá woods (Eschweilera coriacea [DC.] S.A Mori, E. odora Poepp. [Miers] and E. truncata A.C. Sm.) using near infrared spectroscopy - NIRS.
Materials and methods
Study site
The research was carried out with species from terra firme secondary forest at INPA/Brazil’s Experimental Tropical Silviculture Station (02° 35′ 55.5″ S, 60° 02′ 14.8″ W), located at km 23 of the ZF-2 highway (Fig. 1), accessed from km 50 of Highway BR-174 (Manaus- Boa Vista). In a 1-hectare plot (100 × 4 × 25 m sub-plots), 128 trees of different species were inventoried, with emphasis on the species of Eschweilera, which is the dominant genus in the region (Central Amazonian rainforest) and is promising for commercial purposes. Nine trees were selected at random for exploration, based on similar characteristics between individuals of the same species, such as diameter at breast height (DBH) and height. These trees were in the 25 cm ≤ DBH ≤ 50 cm diameter class and visual analyzes of the health status of each tree were also carried out. Discs of 10 cm thickness were cut at DBH from three individuals of each species, Eschweilera coriacea (white matá-matá), E. odora (yellow matá-matá) and E. truncata (black matá-matá), from which specimens (20 × 20 × 30 mm) were manufactured from the cross section, in the direction of the sapwood. Ten specimens were extracted from each individual to obtain the NIR spectra. Samples from each individual were sent to the Wood Anatomy Laboratory (COTI/INPA) for confirmation of species identification in the field.
Analysis procedures
Spectra in the near infrared region were obtained from the radial and tangential planes of the specimens (moisture 12%) using a Fourier-Transform spectrometer (FT-NIR/Termo Fisher Scientific, Antaris II model). The system uses the “Result Integration” software that operates in the 4,000 to 10,000 cm-1 range, with 16 scans and 8 cm-1 resolution. Three readings were taken from each side of the sample to obtain a mean spectrum that represents the sample ([31]).
Partial Least Squares (PLS) regression models were developed by Nascimento & Varejão ([29]) and Nascimento et al. ([30]) to predict the chemical, physical and mechanical properties of Amazonian woods. These models are based on the correlation of the NIR spectra (wood) with test results by the traditional method (destructive methodology), using the TQ AnalystTM software. This enables subsequent results for wood properties to be obtained through non-destructive means, i.e., the NIR spectrum of the wood is obtained and correlated with the chemometric data, with the new spectrum assessed for similarity with the data set.
The data set (NIR spectrum × reference value) is separated into two groups, the first is used to calibrate the equation (2/3 data) and the second to validate the model (1/3 data). The tested models are composed of a set of raw data, with mathematical treatment of 1st and 2nd derivative tests and also the option to apply filters or not. Tab. 1 shows the chemometric parameters used in the predictive models for technological properties, based on 1200 samples from 40 different wood species from the Amazon rainforest, with each species represented by three individuals and 10 specimens.
Tab. 1 - NIR modeling data used to predict the technological properties of Eschweilera woods. (n): number of samples; (Rc2): Determination coefficient - Calibration; (RMSEC): Root mean square error of calibration; (RMSEP): Root mean square error of prediction; (RPD): Ratio of performance to deviation.
Models | Treatment /smoothing | Spectral range (cm-1) |
n | Rc2 | RMSEC | RMSEP | RPD | Uncertainty (%) |
Standard range |
---|---|---|---|---|---|---|---|---|---|
Extractives | 2nd derivative Norris | 7500-4540 | 120 | 0.99 | 0.09 | 0.42 | 4.09 | 0.82 | 1.08-17.30% |
Polyphenols | 2nd derivative Norris | 6100-5900 | 120 | 0.95 | 2.48 | 5.68 | 4.66 | 9.06 | 0.13-13.60% |
Hot water | 2nd derivative Norris | 7500-4540 | 120 | 0.98 | 0.68 | 0.07 | 3.22 | 0.12 | 0.17-13.90% |
Lignin | 2nd derivative Norris | 6070-5000 | 60 | 0.99 | 0.46 | 5.43 | 3.01 | 10.04 | 21.56-44.35% |
Cellulose | 2nd derivative Norris | 5000-4000 | 60 | 0.99 | 0.12 | 3.85 | 3.05 | 4.55 | 35.00-54.85% |
Ash | 2nd derivative Savitzky-Golay | 8450-7300 | 120 | 0.97 | 0.11 | 0.47 | 5.01 | 0.61 | 0.04-3.00% |
Moisture | 2nd derivative Norris | 6070-5500 | 60 | 0.99 | 0.15 | 1.30 | 3.53 | 1.40 | 10.14-15.51% |
Higher calorific value | 1st derivative Savitzky-Golay | 7450-4090 | 90 | 0.94 | 49.30 | 62.40 | 3.99 | 4.01 | 4000-5263 cal g-1 |
Basic density | Untreated | 10000-4000 | 64 | 0.91 | 0.07 | 0.10 | 3.69 | 11.02 | 0.40-1.00 g cm-3 |
Modulus of elasticity | 1st derivative Savitzky-Golay | 5360-4000 | 120 | 0.90 | 1.25 | 1.91 | 4.09 | 4.36 | 7060-19420 MPa |
Modulus of rupture | 2nd derivative Savitzky-Golay | 6020-5075 | 120 | 0.89 | 16.10 | 28.00 | 5.82 | 12.20 | 46.68-200.42 MPa |
All of the models were built from original data obtained by the traditional methodology, as described in: (i) determination of the total extractives content - ethanol/toluene (D1105-96/2013, [4]); (ii) determination of the content of total polyphenols and tannins ([6]); (iii) determination of solubility in hot water (D1110-84/2013, [4]); (iv) Determination of lignin content (D1106-96/2013, [4]); (v) determination of the crude cellulose content ([18]); (vi) determination of ash content (D1102-84/2013, [4]); (vii) determination of moisture (D2016-74/1983, [4]); (viii) determination of the higher calorific value - HCV (D2015-00/2000, [4]); (ix) determination of basic density - BD (NBR 7190/1997, [1]); (x) determination of the modulus of elasticity (MOE) and rupture (MOR) in static bending (555 - [11]).
Statistical analysis
The NIR spectra of the matá-matá woods (total of 90 samples) were applied in different models to predict the physical, mechanical and chemical properties. The results obtained were subjected to statistical analysis to obtain the mean, minimum and maximum values, as well as to analysis of variance and normality tests. When a statistically significant difference in the property being evaluated was found (α = 0.05), the Tukey test (PAST v. 2.17c - [19]) was applied. At the end, a correlation matrix was obtained between the predicted properties. For this, the data that did not meet the assumption of normality were submitted to log10, square root and Box Cox data transformations to meet the assumption of Pearson’s correlation analysis. For variables that did not meet the assumption of normality even after transformations, Spearman’s correlations were applied. These analyzes were performed using the statistical software R v. 4.0.5 ([36]).
Results and discussion
Chemical characterization
The elucidation of the chemical characteristics of wood provides the basis for interpreting its properties in an engineering context, in order to better understand the strengths and limitations of this raw material. The results of the estimation of the technological properties of the matá-matá woods are presented in Tab. 2, Tab. 4 and the analysis of variance (ANOVA) in Tab. 3 and Tab. 5.
Tab. 2 - Results of chemical properties of Eschweilera woods by non-destructive methodology. Each value is an average of four repetitions. (SD): Standard deviation, Means followed by the same letter do not statistically differ (p>0.05) after Tukey test.
Species | Stats | Extractives | Polyphenols | Hot water |
Lignin | Cellulose | Ash |
---|---|---|---|---|---|---|---|
E. coriacea | Max. | 6.37 | 6.18 | 1.42 | 31.83 | 47.48 | 0.64 |
Min. | 5.55 | 5.77 | 0.93 | 30.57 | 44.81 | 0.41 | |
Average | 5.85 a | 5.92 b | 1.25 b | 31.14 a | 46.33 a | 0.49 a | |
SD | 0.45 | 0.23 | 0.28 | 0.64 | 1.37 | 0.13 | |
E. odora | Max. | 7.66 | 3.01 | 3.13 | 30.66 | 46.43 | 0.65 |
Min. | 5.81 | 1.63 | 2.81 | 24.88 | 43.46 | 0.42 | |
Average | 6.48 a | 2.09 c | 2.76 a | 28.89 a | 44.64 a | 0.55 a | |
SD | 1.02 | 0.79 | 0.39 | 3.48 | 1.57 | 0.12 | |
E. truncata | Max. | 6.45 | 10.00 | 2.14 | 31.67 | 45.73 | 0.71 |
Min. | 5.59 | 7.06 | 1.75 | 30.86 | 43.29 | 0.66 | |
Average | 6.08 a | 8.78 a | 1.90 b | 31.21 a | 44.20 a | 0.69 a | |
SD | 0.44 | 1.53 | 0.21 | 0.42 | 1.33 | 0.03 |
Tab. 4 - Results of the physical and mechanical properties of Eschweilera woods by non-destructive methodology. Each value is an average of four repetitions. SD = Standard deviation. Means followed by the same letter do not differ statistically after Tukey test (p>0.05). (HCV): higher calorific value; (BD): basic density; (MOE): modulus of elasticity; (MOR): modulus of rupture.
Species | Stats | Moisture (%) |
HCV (cal g-1) |
BD (g cm-3) |
MOE (MPa ×1000) |
MOR (MPa) |
---|---|---|---|---|---|---|
E. coriacea | Max. | 12.66 | 5.094 | 0.83 | 16.570 | 153.78 |
Min. | 12.52 | 4.972 | 0.71 | 14.310 | 145.28 | |
Average | 12.58 b | 5.033 a | 0.78 a | 15.337 b | 149.32 b | |
SD | 0.07 | 61.000 | 0.07 | 1.144 | 4.27 | |
E. odora | Max. | 13.46 | 5.114 | 0.93 | 14.640 | 147.57 |
Min. | 12.77 | 4.871 | 0.80 | 13.710 | 144.01 | |
Average | 13.21 b | 4.996 a | 0.88 a | 14.253 b | 146.04 b | |
SD | 0.38 | 121.650 | 0.08 | 484.390 | 1.83 | |
E. truncata | Max. | 13.80 | 5.135 | 0.91 | 18.310 | 176.35 |
Min. | 13.11 | 4.849 | 0.74 | 16.300 | 174.81 | |
Average | 13.46 a | 4.993 a | 0.81 a | 17.447 a | 175.73 a | |
SD | 0.35 | 143.010 | 0.09 | 1.034 | 0.82 |
Tab. 3 - Result of the analysis of variance of the chemical properties of the different Eschweilera woods.
Variables | Sum of squares | Mean square | F | P-value |
---|---|---|---|---|
Extractives | 0.6167 | 0.3083 | 0.6398 | 0.5599 |
Polyphenols | 67.4644 | 33.7322 | 33.4435 | 0.0006 |
Hot water | 3.4711 | 1.7355 | 19.1139 | 0.0025 |
Lignin | 10.4790 | 5.2395 | 1.2402 | 0.3542 |
Cellulose | 7.5783 | 3.7891 | 1.8514 | 0.2365 |
Ash | 0.0622 | 0.0311 | 2.8426 | 0.1354 |
Tab. 5 - Results of the analysis of variance of the physical and mechanical properties of the different Eschweilera woods.
Variables | Sum of squares | Mean Square | F | P-value |
---|---|---|---|---|
Moisture | 1.2313 | 0.6156 | 6.8312 | 0.0284 |
HCV | 2.978 | 1.489 | 0.1146 | 0.8936 |
BD | 0.0124 | 0.0062 | 1.1069 | 0.3898 |
MOE | 15.823.089 | 7.911.544 | 9.0805 | 0.0153 |
MOR | 1.589.897 | 794.948 | 107.3328 | 0.0000 |
The extractives in organic solvents (toluene and ethanol) and solubility in hot water showed maximum concentrations of 7.66% and 3.13% for Eschweilera odora (yellow matá-matá), where these compounds add up to more than 10% in dry mass of this species (Tab. 2). The analysis of variance (ANOVA) was applied to the extractives concentration and no overall significant difference among species (p = 0.5599) was found. However, for water solubility, the existence of differences in mean values among species (p = 0.0025 - Tab. 3) was confirmed by the Tukey test, indicating that there is a difference in the concentration in E. odora (2.76%) compared with E. coriacea (white matá-matá - 1.25%) and E. truncata (black matá-matá - 1.90%).
A range of compounds with low molecular weight was found in the extractives, including resins, gums, terpenes, alkaloids, and flavonoids. Also within these compounds are several chemical classes of phenolic substances, such as tannins. The extraction quantification process uses, in general, nonpolar and/or low polar solvents (hexane, benzene and chloroform) that are responsible for the removal of resins, waxes and essential oils. Toluene-ethanol, acetone, methanol and water, which are medium- to high-polarity solvents, carry a large amount of compounds such as flavonoids, tannins and sugars ([6], [26]). For tannins, a higher concentration was observed in the extractives of E. truncata (10%). The Tukey test confirmed the formation of three classes of values for polyphenols and total tannins (p = 0.0006), with E. truncata (8.78%) differing from the other two species and E. coriacea (5.92%) displaying significant differences in relation to E. odora (2.09%).
Secondary metabolites (extractives) can be found in the middle lamella, parenchymal cells, and vessels and voids in the xylem tissue. The anatomical aspects of the three species of Eschweilera studied are presented in Fig. 2, where the similarity can be observed in the vasicentric paratracheal parenchyma and in lines (P) and solitary vessels (V) obstructed by organic substances (extractives).
Fig. 2 - Cross-sectional view (10×) of matá-matá (Eschweilera sp.) wood samples. (A): E. coreacea; (B): E. odora; (C): E. truncata; (P): parenchyma; (V): vessels.
The macrocomponents (primary metabolism) of Eschweilera species were in the range of 44.20-46.33% for cellulose and 28.89-31.21% for lignin (Tab. 2). Despite this variation, no statistical difference was observed between the means of each species for these compounds (lignin: p = 0.3542; cellulose: p = 0.236). Comparing these results with data from the literature for tropical woods, it was found that these values fall within the standard range for species in the region ([40], [12]). As for the mineral compounds present in the xylemic tissue, which in technology are quantified as ash (a product of carbonization), in the present study these were quantified in concentrations < 0.70%, with no statistical difference among species (p = 0.1354).
Cellulose is the most abundant polysaccharide in nature. It is formed of a chain composed of glucose units joined by 1-β, 4-glycosidic bonds. Plant cell walls are compactly arranged in such a way that the fibers present clearly crystalline regions. Due to its chemical and physical properties, as well as its supramolecular structure, cellulose is linked to the volumetric contraction of the species ([40], [50]).
Lignin is another major component of wood. It is an amorphous, heterogeneous and predominantly aromatic polymer, which involves polysaccharides in the cell structure. It is characterized by the high number of -OCH3 and -OH groups, and its chemical structure differs depending on whether it comes from hardwood or coniferous wood. Lignified cell walls tend to contract less than non-lignified ones. Since lignin provides resistance to impact, compression and bending, it decreases water permeability, in addition to protecting against microorganisms by preventing the penetration of destructive enzymes into the cell wall ([12], [44]).
Extractives, or secondary metabolites, include a wide range of organic compounds, such as terpenes, fatty acids, aromatic compounds (mainly phenolic compounds) and vegetable oils. These substances have a physiological function in the tree, whether as a reserve material, for energy storage or antioxidant activity against environmental stressors, among others. They are responsible for certain characteristics of the wood, such as taste, color, flammability, and especially the natural resistance to xylophagous organisms ([23]).
In addition to macrometabolites and extractives, small amounts of mineral compounds are found in wood, which are absorbed from the soil during the growth of trees and deposited in the cell wall. In general, these compounds are analyzed in ash that is characterized as alkaline material (pH 8-13), formed by carbonates, salts and oxides of Ca, K, Mg, P, Na and Si ([39], [44]).
Castilho ([9]) carried out the chemical characterization of Eschweilera iquitoensis wood (machimango colorado), a species from the Peruvian Amazon, using traditional destructive methodology, reporting values of 30% lignin, 8% total extractives and 1% ash. Moutinho et al. ([28]), investigating matá-matá woods (E. amazonica, E. coriacea, E. idatimon and E. ovata) from the southeastern region of Pará (Brazil) recorded values from 23.40 to 30.80% for lignin content and extractives concentrations from 3.60% (white biriba, E. ovata) to 11.10% (E. coriacea). Nascimento et al. ([32]), studying the chemical profile of extractives of tree species from the Lecythidaceae family of the Central Amazon, found total extractives levels ranging from 1.93% (castanha sapucaia, Lecythis usitata) to 7.10% (E. odora), and total polyphenols concentrations of 0.33% (white tauari, Couratari stellata) to 2.79% (tauari, Couratari guianensis).
Physical and mechanical characterization
The results of predictions for the physical and mechanical properties of matá-matá wood are presented in Tab. 4. The analysis of variance did not detect significant differences for the values of higher calorific value (HCV, p = 0.8936) and basic density (BD, p = 0.3889) between the studied species, with the HCV ranging from 4.993-5.033 cal g-1 and BD from 0.78-0.88 g cm-3. As for moisture content, the highest value was observed for E. truncata (13.46%). This was evidenced by the Tukey test with a significant difference (p = 0.0284) when compared with the means of E. Coriacea (12.58 %) and E. odora (13.21%). The same statistical difference was observed for modulus of elasticity (MOE, p = 0.0153) and modulus of rupture (MOR, p < 0.0001), with a variation of 14,253-7,447 MPa and 146.04-175.73 MPa, respectively (Tab. 4, Tab. 5). It is worth mentioning that the values predicted for the properties are in the range for high density Amazonian woods ([21], [5]).
Kollmann & Cotê Junior ([24]) and Silveira et al. ([43]) state that the physical and mechanical properties depend on the moisture content of the wood, with this variable being inversely proportional to BD, HCV, MOE and MOR, i.e., the greater the water content, the greater the interrelation of hydroxyls with cellulose and hemicellulose molecules, increasing dimensional instability and defects in pieces of wood and reducing the energy potential, as well as the biological and mechanical resistance of the wood. The statistical analysis indicated the formation of two moisture groups with lower (E. coriacea and E. odora) and higher moisture content (E. truncata). Within this range, Silva et al. ([41]) observed a moisture level of 12.16-14.22% when applying near infrared spectroscopy (NIR) to predict this property in wood waste from the Amazon.
The calorific value of wood is one of the main parameters reflecting the energy production of this material, along with the concentration of chemical compounds, moisture and BD. The Amazonian biome contains forest species that have not been studied for energy purposes, although energy production from wood is widespread, mainly for domestic use and for the production of charcoal for the steel industry. A study by Silva et al. ([42]) used a traditional methodology to characterize the energy values of wood waste for the production of briquettes, finding high HCV values that ranged from 5.213 cal g-1 (angelim pedra, Hymenolobium petraeum) to 5.832 cal g-1 (jatobá, Hymenaea courbaril). Moutinho et al. ([28]) found lower values for several matá-matá woods (Eschweilera sp.), ranging from 4.438-4.758 cal g-1. However, in one of the few studies using non-destructive prediction (NIR), Silva et al. ([41]) characterized waste wood from the state of Amazonas (Brazil), finding HCV values similar to those of the present study, ranging from 4.608 (cedrinho, Scleronema sp.) to 4.928 cal g-1 (louro, Ocotea sp.).
One of the key variables for wood usage is density, which is closely related to chemical composition and anatomical structure ([24], [49]). Applying the non-destructive methodology (NIR) on wood from Picea sitchensis (Sitka spruce), Pinus sylvestris (Scots pine) and 24 tropical species, Nuopponen et al. ([33]) sought to establish the relationship between chemical constitution and wood density, concluding that tropical wood had greater density than spruce and pine woods, and also verifying that tropical species had a higher content of lignin in their composition than other woods.
Araújo et al. ([3]), studying managed wood species in the Amazon and applying the traditional methodology, identified the predominance of wood with high density (murici, Byrsonima crispa, 0.71 g cm-3; piãozinho, Micrandropsis sclerorylon, 1.18 g cm-3). Within this range, Fróes et al. ([15]) found mean values of 0.77 g cm-3 for E. truncata wood by applying the same (destructive) methodology, while Nascimento et al. ([31]) determined the density of E. odora by NIR, obtaining mean values of 0.80 g cm-3. In the current study the matá-matá woods were classified as high density (BD > 0.70 g cm-3).
Knowledge of the mechanical properties of wood is essential for structural purposes. Balboni et al. ([5]) characterized the physical and mechanical properties of the Amazonian woods Pseudopiptadenia psilostachya (timborana) and E. ovata obtained MOE values of 16,030-17,870 MPa and MOR of 103.90-127.10 MPa, with the greatest resistance observed for Eschweilera wood. In the database of the Institute of Technological Research ([22]), the tropical species Hymenaea courbaril is classified as a high resistance wood with MOR of 151.8 MPa and MOE of 17,062 MPa.
Predictive studies based on high-quality hardwoods are not as common. Araújo et al. ([3]) used the Stress Wave Timer technique to determine the MOE of Amazonian woods, obtaining values from 11,049 (breu vermelho, Protium puncticulaton) to 14,201 MPa (Micrandropsis sclerorylon). In recent years, several studies have been developed to validate models based on NIR spectroscopy in order to predict the mechanical properties of solid wood. Andrade et al. ([2]) determined the mechanical strength of Eucalyptus urophylla (red eucalyptus) wood by NIR spectroscopy and obtained MOR of 94.40 MPa and MOE of 15,972.20 MPa. Schimleck et al. ([38]) compared several NIR predictive models for six species of pines (Pinus caribaea, P. chiapensis, P. maximinoi, P. oocarpa, P. taeda and P. tecunumanii), where MOR ranged from 55.15-70.13 MPa and MOE from 5,930.21-9,110.24 MPa. Also using spectroscopy, Mancini et al. ([27]) recorded MOR values of 102.40 MPa and MOE values of 12,132.00 MPa for Castanea sativa (sweet chestnut). Yu et al. ([51]) compared the mechanical properties of Quercus mongolica (mongolian oak) wood using NIR modeling and observed values for breaking strength in the range of 120.00 to 200.00 MPa and resistance to elasticity from 12,000 to 18,000 MPa.
The non-destructive prediction method used for technological characterization of Eschweilera woods is entirely based on near infrared spectroscopy (NIRS), a tool based on vibrational spectroscopy. This involves the exposure of wood to electromagnetic radiation in the range of 4,000 to 12,000 cm-1, which allows the estimation of various attributes of wood based on the combination of chemical constituents with physical and mechanical properties by multivariate statistics and chemometrics. The technological properties of Eschweilera woods predicted by the NIR models in this study are within the standard range for tropical woods based on traditional methods, thus proving the model accuracy in the variable predictions.
To investigate the possible biological relationships between the physical, mechanical and chemical characteristics of matá-matá wood, principal component analysis (PCA) was carried out based on Pearson’s correlation matrix (Fig. 3, Tab. 6). The first four components explained 86% of the total variation. Based on the PC1 and PC2 (accounting for 62% of the total variation - Fig. 3), four groups of wood properties could be identified. Group 1 (G1) included ash, polyphenols, MOE and MOR; group 2 (G2) included cellulose, lignin and HCV; group 3 (G3) included BD, extractives and solubility in hot water; and group 4 (G4) included only moisture. Among the wood properties grouped in G1, MOR showed a highly significant relationship with MOE (Tab. 6), which can be partly explained by the macro components of wood (cellulose and lignin). Fujimoto et al. ([16]) stated that the OH group (linked to specific NIR bands) in the semicrystalline or crystalline regions of cellulose strongly affect wood resistance (MOR and MOE) in flexural tests. As regards the strong relationship of polyphenols with mechanical strength, little evidence is available in the literature. A possible explanation could be proposed here, as phenolic compounds present in extractives, such as highly reactive tannins, form intra and intermolecular hydrogen bridges with various compounds in the wood ([35]). Similar to polyphenols, the correlation between ash and wood strength has been little studied, and could possibly be explained by the intramolecular interactions of carbonates, phosphates and silicates within the plant cell ([20]).
Fig. 3 - Factorial distribution of the analyzed wood properties by PCA. (BD): Basic density; (MOE): Modulus of elasticity; (MOR): Modulus of rupture; (HCV): Higher calorific value.
Tab. 6 - Result of Pearson’s correlation among all variables obtained from the relationships between chemical, mechanical and physical variables Eschweilera wood. (BD): Basic density; (MOE): Modulus of elasticity; (MOR): Modulus of rupture; (HCV): Higher calorific value. (*): For the correlation of this variable with the others, Spearman correlations were applied. (Class): strength of the correlation based on Callegari-Jacques ([8]): moderate (0.30 ≤ r < 0.60); strong (r ≥ 0.60).
Properties | Variable 1 | Variable 2 | Pearson r | P-value | Class |
---|---|---|---|---|---|
Chemical | Extractives | Cellulose | -0.47 | 0.2030 | Moderate |
BD | 0.46 | 0.2017 | Moderate | ||
Hot water | 0.36 | 0.3380 | Moderate | ||
HCV | -0.41 | 0.2780 | Moderate | ||
Polyphenols | Lignin* | 0.33 | 0.3800 | Moderate | |
MOE | 0.87 | 0.0020 | Strong | ||
MOR | 0.84 | 0.0040 | Strong | ||
Mechanical | MOR | Ash | 0.56 | 0.1130 | Moderate |
MOE | 0.90 | <0.0001 | Strong | ||
Physical | BD | Hot water | 0.30 | 0.4270 | Moderate |
HCV | Ash | -0.33 | 0.3920 | Moderate |
Among the variables grouped in G2, the positive correlation of lignin and HCV is well known, especially in energy studies: the higher the lignin content, the greater the energy potential (HCV), as the higher the concentration of fixed carbon in the matrix, the stronger the glow when burned ([46], [47], [42]). As for the cellulose/lignin connection, the correlation is negative ([12]). Regarding the G3 wood attributes, the extractives/BD association has been explained in several studies ([31], [45], [15]), since high-density tropical forest species grow slowly in diameter, thus having time for greater deposition of compounds in the xylem ([40], [10]). Conversely, pioneer species, which are generally fast growing, display low density and concentration of extractives ([49], [23]).
Wood moisture (the only attribute included in G4) has an indirect correlation with most properties. For example, Silveira et al. ([43]) studied the inverse correlation between moisture and BD, with low density wood having a higher moisture content. In the energy industry using wood biomass, species with higher moisture content have a low HCV and consequently a lower energy yield ([41], [42]). By assessing the influence of extractives on hydraulic architecture in tree species of the Central Amazon, Santos ([37]) found that certain species evolved an adaptive strategy to face water stress, whereby a high moisture content was associated with a higher concentration of aqueous extractives.
The most significant correlations among the analyzed wood properties (Tab. 6) were found between MOR and polyphenols (r = 0.84, p = 0.004), MOE and polyphenols (r = 0.87, p = 0.002), and MOE and MOR (r = 0.90, p = 0.000). The following sequence was obtained from the variables classified as moderate correlation: MOR/ash > Extractives/cellulose > Extractives/BD > Extractives/HCV > Extractives/hot water > Lignin/polyphenols > HCV/ash> BD/hot water. The largest contributions to these combinations are associated with extractives, polyphenols and MOR.
In recent studies on Amazonian woods, Silva et al. ([42]) found significant correlations between extractives and BD (r = 0.56) as well as ash and HCV (r = -0.58). Fernandes et al. ([13]) found a significant correlation between extractives and hot water (r = 0.550), while Soares et al. ([45]) working with Eucalyptus sp., also observed significant correlations between extractives and BD (r = 0.48) and extractives and cellulose (r = -0.563). Other studies showed correlations between chemical and physical variables in wood, including Moutinho et al. ([28]) who reported a moderate correlation for the extractives/HCV combination (r = -0.390). It is worth mentioning that in all of these studies similar correlation trends were observed as in the present study.
As for physical properties, Moutinho et al. ([28]) observed a correlation between BD and HCV (r = 0.360), while for mechanical properties, Fernandes et al. ([13]) showed a highly significant relationship between MOE and MOR (r = 0.779), which is lower than that detected in this study (r = 0.902). MOE is a wood rigidity parameter that is highly and positively correlated with strength and therefore can be used to assess wood strength parameters like MOR ([25]).
Conclusions
In this study, chemical, physical and mechanical properties of the wood of Eschweilera coriacea (white matá-matá), E. odora (yellow matá-matá), and E. truncata (back matá-matá), estimated by NIR spectroscopy, were compatible with those obtained by the destructive determinations of tropical species and also with other species of the genus Eschweilera. Knowledge of the chemical composition and the distribution of chemical components in plant tissues is key to improve the use of wood and its commercialization in different industrial sectors, such as civil or naval construction, manufacture of furniture, boards and panels, etc.
The technological characterization of matá-matá woods by near infrared spectroscopy (NIRS) provided robust and satisfactory results generated in short time. Besides, in contrast to the traditional methodology, there is no need to destroy the sample and do not produce waste. Finally, PLS models used in this study to predict wood technological properties showed high performance with high determination coefficient (> 0.90) and RPD (ratio of performance to deviation > 3), and low calibration and prediction errors (RMSEC and RMSEP, respectively).
Acknowledgements
The authors thank the Coordenação de Aperfeiçoamento de Pessoal de Nível Superior - MEC/CAPES (Ph.D. Candidate CFT/INPA to 88882.4444 19/2019-01); the MCTI/CNPq/FAPEAM project “INCT Madeiras da Amazônia” for financial support; Jorge A. Freitas for the identification of the sample woods; and Ana Julia O. Godoy for reviewing the English text.
Authors contribution
All authors contributed to the design, results and discussion of this study. CSN and CCN are primarily responsible for the conception, design, analysis and writing of the manuscript. RDA produced and processed NIR spectral data. CSN performed the chemical, mechanical and physical analysis of the wood. JCRS edited the images and supported the statistical analysis. NH is the general coordinator of the project “INCT Madeiras da Amazônia/MCT/CNPq/FAPEAM”, which supported field activities and collection. CCN made a critical review of the paper content. Finally, all authors approved the final version of the manuscript.
References
Gscholar
Gscholar
Gscholar
Gscholar
Gscholar
Gscholar
Gscholar
Gscholar
Gscholar
Gscholar
Gscholar
Gscholar
Online | Gscholar
Authors’ Info
Authors’ Affiliation
Graduate Program in Tropical Forest Science (PPG-CFT), National Institute of Amazonian Research (INPA). Av. Efigênio Sales, 2.239 - Aleixo - CEP 69060-020, Manaus, AM (Brazil)
Roberto Daniel de Araújo 0000-0002-9653-305X
Engineering Laboratory and Wood Artifacts (LEAM/COTEI), National Institute of Amazonian Research (INPA). Av. André Araújo, 2.936 - Petrópolis - CEP 69067-375, Manaus, AM (Brazil)
Master’s, Graduate Program in Environmental and Forestry Sciences (PPG-CIFA), Federal University of Amazonas (UFAM). Av. Rodrigo Otávio, Coroado - CEP 69067005, Manaus, AM (Brazil)
Forest Management Laboratory (LMF/CODAM), National Institute of Amazonian Research (INPA). Av. Efigênio Sales, 2.239 - Aleixo - CEP 69060-020, Manaus, AM (Brazil)
Corresponding author
Paper Info
Citation
Nascimento CS, Nascimento CC, Araújo RD, Soares JCR, Higuchi N (2021). Characterization of technological properties of matá-matá wood (Eschweilera coriacea [DC.] S.A. Mori, E. odora Poepp. [Miers] and E. truncata A.C. Sm.) by Near Infrared Spectroscopy. iForest 14: 400-407. - doi: 10.3832/ifor3748-014
Academic Editor
Giacomo Goli
Paper history
Received: Jan 10, 2021
Accepted: Jul 02, 2021
First online: Sep 01, 2021
Publication Date: Oct 31, 2021
Publication Time: 2.03 months
Copyright Information
© SISEF - The Italian Society of Silviculture and Forest Ecology 2021
Open Access
This article is distributed under the terms of the Creative Commons Attribution-Non Commercial 4.0 International (https://creativecommons.org/licenses/by-nc/4.0/), which permits unrestricted use, distribution, and reproduction in any medium, provided you give appropriate credit to the original author(s) and the source, provide a link to the Creative Commons license, and indicate if changes were made.
Web Metrics
Breakdown by View Type
Article Usage
Total Article Views: 31321
(from publication date up to now)
Breakdown by View Type
HTML Page Views: 26702
Abstract Page Views: 2033
PDF Downloads: 2100
Citation/Reference Downloads: 2
XML Downloads: 484
Web Metrics
Days since publication: 1398
Overall contacts: 31321
Avg. contacts per week: 156.83
Article Citations
Article citations are based on data periodically collected from the Clarivate Web of Science web site
(last update: Mar 2025)
Total number of cites (since 2021): 10
Average cites per year: 2.00
Publication Metrics
by Dimensions ©
Articles citing this article
List of the papers citing this article based on CrossRef Cited-by.
Related Contents
iForest Similar Articles
Research Articles
Physical, chemical and mechanical properties of Pinus sylvestris wood at five sites in Portugal
vol. 10, pp. 669-679 (online: 11 July 2017)
Research Articles
Mechanical and physical properties of Cunninghamia lanceolata wood decayed by brown rot
vol. 12, pp. 317-322 (online: 06 June 2019)
Research Articles
Validation of models using near-infrared spectroscopy to estimate basic density and chemical composition of Eucalyptus wood
vol. 17, pp. 338-345 (online: 03 November 2024)
Research Articles
Estimation of total extractive content of wood from planted and native forests by near infrared spectroscopy
vol. 14, pp. 18-25 (online: 09 January 2021)
Research Articles
NIR-based models for estimating selected physical and chemical wood properties from fast-growing plantations
vol. 15, pp. 372-380 (online: 05 October 2022)
Research Articles
Kinetic analysis of poplar wood properties by thermal modification in conventional oven
vol. 11, pp. 131-139 (online: 07 February 2018)
Research Articles
Density, extractives and decay resistance variabilities within branch wood from four agroforestry hardwood species
vol. 14, pp. 212-220 (online: 02 May 2021)
Technical Advances
Technical properties of beech wood from aged coppices in central Italy
vol. 8, pp. 82-88 (online: 04 June 2014)
Research Articles
Physical-mechanical properties and bonding quality of heat treated poplar (I-214 clone) and ceiba plywood
vol. 8, pp. 687-692 (online: 17 December 2014)
Research Articles
Physical and mechanical characteristics of poor-quality wood after heat treatment
vol. 8, pp. 884-891 (online: 22 May 2015)
iForest Database Search
Search By Author
Search By Keyword
Google Scholar Search
Citing Articles
Search By Author
Search By Keywords
PubMed Search
Search By Author
Search By Keyword