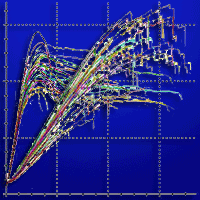
Physical and mechanical characteristics of poor-quality wood after heat treatment
iForest - Biogeosciences and Forestry, Volume 8, Issue 6, Pages 884-891 (2015)
doi: https://doi.org/10.3832/ifor1229-007
Published: May 22, 2015 - Copyright © 2015 SISEF
Research Articles
Abstract
Poor-quality Corsican pine (Pinus nigra subsp. laricio (Poir.) Maire) and Douglas fir (Pseudotsuga menziesii (Mirb.) Franco) wood samples were heat treated with the aim of testing the improvement of wood quality that could increase their economic value. Wood properties were measured to assess quality in treated and non-treated materials, including density, hardness, anti-swelling efficiency (ASE), modulus of elasticity (MOE), modulus of rupture (MOR), and compression strength. The results showed higher dimensional stability in heat-treated wood, yet mechanical performance in compression and bending strength were only marginally affected by loss of density. Despite having a relatively low density after heat treatment, Corsican pine sapwood has potential in manufacturing higher-value products. In contrast, heat treatment applied to Douglas fir wood did not appear economically viable; insufficient differences were detected between the naturally desirable characteristics of this species and heat-treated samples.
Keywords
Thermowood, Density, Compressive Strength, Bending Strength, Sapwood, Wood Quality, Douglas Fir, Corsican Pine
Introduction
High-temperature heat treatment is considered a suitable method to improve wood characteristics. It reduces toxic chemical applications normally required to increase wood durability ([26], [58], [37]) and enhance dimensional stability, reducing the hygroscopic behavior of the material ([29]). High-temperature treatment can be viewed as a type of wood modification, due to the possibility of controlling color changes ([7], [25], [16], [19]) and mechanical performance ([7], [59], [6]). The process results in a new material in terms of durability, wood-water relationships and some mechanical properties, which exhibits performance dependent on the specific high-temperature treatment conditions ([35], [36], [18]), but the wood product does not present any environmental hazards in its disposal ([24]). The final product typically has the disadvantage of reduced mass and mechanical performance, although some difference in degree of change has been observed between softwoods and hardwoods depending on the treatment method ([27], [33]).
Therefore, the overall extent of modification and the final result of the process is quite variable. In this study, we assessed heat treatment effects on Corsican pine and Douglas fir wood properties obtained from a conifer reforestation site in Italy, with particular consideration of different sapwood and heartwood behavior. This timber is considered poor quality and is currently used as biomass for energy or packaging.
Materials and ethods
Study area
Forty Douglas fir (Pseudotsuga menziesii) and 40 Corsican Pine (Pinus nigra ssp. laricio) trees were sampled in an artificial mixed stand in Poggio Nibbio near Viterbo, Central Italy (latitude 42° 22′ N, longitude 12° 10′ W). Trees were arranged in rows with a cross-distance of 4 m; the stand was thinned to promote tree regeneration and introduction of indigenous hardwoods. The harvested wood is generally of poor quality due to the fact that the trees have grown quite fast, and not straight, producing large rings that contain a high proportion of earlywood. The sapwood is also quite large, particularly in Corsican pine.
From each of the eighty trees sampled, single boards 6 cm thick, 80 cm long, and 7 cm in width were produced and subsequently crosscut to obtain two half-boards of approximately 40 cm in length. From these two half boards, we obtained twin specimens measuring 2 × 2 cm in the transverse radial and tangential directions, and 40 cm longitudinally. The wood samples were largely juvenile, approximately 10 years in cambial age, and evidence of reaction wood was not observed in any samples analyzed. For each of the 2 × 2 × 40 cm sample pairs, one sample was heat treated, and the second sample was left untreated. Subsequently, two blocks measuring 2 × 2 × 3 cm in size were cut from the end of each sample to measure post-treatment/treated (t) and control sample/ not treated (nt) physical properties and compression strength; the total sample size was 160 specimens. Only sapwood samples were used in Corsican pine (CP), as the heartwood was limited to a very narrow inner portion of the sample section. However, the heartwood occupied a much larger surface area and increased number of rings in Douglas fir; therefore it was possible to distinguish and discuss the results for sapwood (D-S) and heartwood (D-H) samples separately.
Heat treatment
Thermowood treatment was performed at the Margaritelli farm in France (⇒ http://www. margaritelli.com/france) according to the following steps:
- Preliminary heating stage at 110 °C.
- Drying phase. The wood was fully dried to prepare it for high-temperature treatment by a gradual warming over 14 h until a temperature of 140 °C was reached. An atmosphere of superheated steam, with low oxygen content, prevented the formation of cracks and alterations within the wood. At the end of this phase, wood moisture content was reduced to little more than 0%.
- High-temperature treatment phase. Application of 210 °C for 2 h.
- Cooling phase. Following the heat treatment, the cooling phase slowly reduced the wood temperature to 80-90 °C over the course of 15-20 h, allowing for moisture adsorption. The wood typically measured equilibrium moisture content between 3-4% at the end of this treatment.
Physical parameters
Untreated samples in fresh state were conditioned in the laboratory at 20 °C and 65% relative humidity (RH), reaching an equilibrium moisture content (EMC) of 12%. Half of the samples were then subjected to heat-treatment; after the treatment the heat-treated samples showed a moisture content between 8 and 11%. Density of samples was measured and their values were subsequently adjusted in treated samples to 12% (ρ
12) using the UNI-ISO-1361 formula for moisture content correction (eqn. 1):
where W
is the wood moisture measured as W
= [(m
w - m
o)/m
o] · 100, m
w is the fresh weight, m
0 is the dry weight (0% moisture content), and K
is the coefficient of volumetric shrinkage, assumed to be K
= 0.85 ρ
w. We adopted the above procedure to compare all the physical and mechanical properties of treated samples with the untreated ones at the same moisture content.
Following EMC measurements, all samples (both treated and untreated) were soaked in water until a constant weight was reached, which was considered the maximum water content, and sample linear size and volume were measured again. The samples were subsequently placed in a 103 °C oven until achieving a constant weight and dry state. Size and weight of the samples were measured again, and moisture content of the fully soaked samples (both treated and untreated) was determined by a gravimetric system.
The following parameters were examined by measuring the linear size of samples at oven-dry moisture content: total shrinkage (β
t, β
r) coefficients, covering the entire hygroscopic range; coefficient of anisotropy (Ψ
), which is the ratio between tangential and radial shrinkage; and basic density (ρ
0), which is the ratio between dry weight and maximum volume (over the fiber saturation point).
Dimensional change in specimens was evaluated using two methods: maximum shrinkage determination and swelling coefficient or anti-swelling efficiency (ASE
). ASE
is an approach commonly employed to evaluate dimensional stabilization processes ([35], [36]), however differences in the application to dry and soaked specimens must be considered. Dimensional changes of oven-dried samples compared with maximum water content at room temperature results in the most notable effects.
We therefore applied the protocol of Santos ([44]), and ASE
was calculated by the formula (eqn. 2):
where Su is the linear or volumetric swelling coefficient of untreated wood, and S is the linear or volumetric swelling coefficient of treated wood.
Mechanical properties
Three samples of each species, taken from 40 specimens of each taxon (totalling 240 samples, 120 Corsican pine and 120 Douglas fir), were tested to measure main mechanical properties, and differences between treated and untreated wood.
All test samples were prepared by cutting pieces from the remaining wood strips; and all mechanical tests were performed using a Zwick-Roell universal testing machine.
Three mechanical tests ([42]) were performed to evaluate the following:
- Compression strength in the longitudinal direction, using specimens 2 × 2 × 3 cm in accordance with UNI-ISO-3787 ([56]).
- Modulus of elasticity (MOE), using a three-point bending test with a preload of 50 N and a machine head speed of 8 mm/min. The distance between the support points was 28 cm, and the load was applied in a tangential direction at a point 14 cm from the supports.
- Modulus of rupture (MOR) during bending of 2 × 2 × 32 cm specimens, as per UNI-ISO-13061-3.
- Hardness (Brinell hardness, HB) according to UNI-EN-1534 ([52]), which was carried out using a preload of 50 N and a test speed of 15 mm/min. All samples were tested tangentially and measured 30 × 20 × 20 mm in size.
The treated sample moisture content (≈8%) was below the reference condition, and thus the measured value was adjusted to 12% moisture by adopting the formula provided by the UNI-ISO-3787 ([56]) standards (eqn. 3):
where P
max is the maximum load and MC
t is the moisture content of treated specimen.
Statistical analysis
Pairwise differences in physical and mechanical properties of wood samples were tested by two-sample Kolmogorov-Smirnov test (α = 0.05). Pearson’s correlation coefficients were calculated to assess the relationships between density and physical and mechanical properties of treated and non-treated wood. The relationships between density and other wood properties were further investigated by linear regression analysis.
Results and discussion
The non-treated wood of the Corsican pine and Douglas fir obtained from the Poggio Nibbio site had specific densities (Fig. 1c) that fall within the lower threshold for the two species (467 kg m-3 for Douglas fir and 517 kg m-3 for Corsican pine - [38]), and are even low compared with other sites in Italy ([21], [49]).
Fig. 1 - Physical characteristics of treated (t) and untreated (nt) Corsican pine (CP), Douglas fir heartwood (D-H), and Douglas fir sapwood (D-S). (a) Tangential shrinkage (βt) and radial shrinkage (βr); (b) anisotropy coefficient; (c) density; and (d) basic density. (*), (**), (***): levels of significance between treated (t) and untreated (nt) specimens at P < 0.05, P < 0.01, and P < 0.001, respectively using the Bonferroni test. Vertical bars represent standard error.
Results showed the mechanical properties of non-treated Corsican pine wood (45 MPA for compression strength - Fig. 2a-d, Tab. 1) were comparable to previously published values ([38], [21], [30]), except for MOE which was lower. Douglas fir sapwood showed lower density values compared to heartwood (Fig. 1c), but the mechanical properties revealed little difference between the two wood portions (Fig. 2a-d, Tab. 1). However, sapwood shrinkage (Fig. 1a) was higher than heartwood, which was similarly reported by Metsä-Kortelainen ([34]).
Fig. 2 - Mechanical properties of treated (t) and untreated (nt) specimens of Corsican pine (CP), Douglas fir heartwood (D-H) and Douglas fir sapwood (D-S): (a) Brinell test; (b) Compression strength; (c) MOR (modulus of rupture to bending stress); (d) MOE (static bending modulus of elasticity). (*), (**), (***): levels of significance between treated (t) and untreated (nt) specimens at P < 0.05, P < 0.01, and P < 0.001, respectively using the Bonferroni test. Vertical bars represent standard error.
Tab. 1 - Descriptive statistics. (t): treated specimens; (nt): untreated specimens; (MOR): modulus of rupture to bending stress; (MOE): static bending modulus of elasticity; (SD): standard deviation; (n): sample size.
Wood type |
Stats | Compression strength (σ12) |
MOR | MOE | Brinell test (HB) |
||||
---|---|---|---|---|---|---|---|---|---|
nt | t | nt | t | nt | t | nt | t | ||
Douglas fir Heartwood |
mean | 53.17 | 49.11 | 89.16 | 69.73 | 10955.71 | 9976.07 | 17.78 | 13.55 |
SD | 7.82 | 5.92 | 25.39 | 18.23 | 1979.11 | 1381.77 | 7.58 | 6.16 | |
n | 28 | 28 | 28 | 28 | 28 | 28 | 28 | 28 | |
Douglas fir Sapwood |
mean | 53.53 | 46.84 | 91.46 | 73.09 | 11279.17 | 9680.00 | 21.13 | 13.17 |
SD | 5.54 | 8.36 | 14.96 | 19.79 | 1525.39 | 1319.32 | 8.77 | 5.78 | |
n | 12 | 12 | 12 | 12 | 12 | 12 | 12 | 12 | |
Corsican Pine |
mean | 45.52 | 47.16 | 82.43 | 64.83 | 7723.33 | 8628.46 | 24.95 | 23.00 |
SD | 8.28 | 7.80 | 21.94 | 20.12 | 2259.01 | 1625.38 | 9.91 | 11.83 | |
n | 40 | 40 | 40 | 40 | 40 | 40 | 40 | 40 |
The most important heat treatment outcome was an increased dimensional stability. In addition, a significant (P < 0.001) decrease in shrinkage values (approximately 42% in Douglas fir and 40% in Corsican pine) were observed, as compared with non-treated wood (Fig. 1a).
Wood modification significantly (P < 0.05) reduced the anisotropy coefficient in Corsican pine (≈14% - Fig. 1b). Results showed a 12% change in Douglas fir heartwood, though not statistically significant. Militz ([35]) reported similar results for stone pine.
Heat treatment effects were reflected by an ASE
value of 44% (Fig. 3) for Douglas fir and Corsican Pine, which exceeded values reported for hardwoods, including eucalyptus (24% - [44]) and silver oak (<40% - [47]), and some tropical species (9.2-14.8% - [28]). These values were considerably lower compared to Chinese fir (Cunninghamia lanceolata, >60% - [14]). Moreover, the ASE
value in Douglas fir was comparable to the volume swelling obtained by Li et al. ([33]).
Fig. 3 - Anti-swelling efficiency (ASE
) coefficient, as measured in tangential (ASE
t) and radial (ASE
r) directions with regard to volume (ASE
v).
Results showed increased ASE
values in the tangential direction for Corsican pine and Douglas fir heartwood samples, consistent with P. banksiana ([40]), P. sylvestris and P. radiata ([35]). However, despite substantial stability improvement in the tangential direction, the anisotropy coefficient (Fig. 1b) remained quite high in heat-treated specimens, congruent with results observed by Esteves et al. ([16], [17], [19]). This minor effect of the treatment in the radial direction might be due to the higher lignin content, which is less sensitive to heat ([22]).
Heat treatment did not significantly affect specific density in Douglas fir and Corsican pine. A marked reduction in specific density of heat-modified wood was previously detected in hardwoods ([58], [28]) and conifers, including P. elliottii ([45]), P. sylvestris and Picea abies ([9]). A lower reduction in treated wood specific density, comparable to our experimental results, was observed in P. sylvestris ([60]).
Following heat treatments, we observed a general decrease in mechanical performance in Douglas fir sapwood and heartwood. This result is consistent with most conifers treated at approximately 200 °C ([8], [18], [4]) or higher (280 °C - [48], [18]). We also observed a reduction in Brinell hardness previously detected in spruce, beech, and red oak ([4]) treated at 190 °C, and P. brutia
treated at temperatures exceeding 180 °C ([3]). As for Corsican pine, a behavior similar to Douglas fir heartwood was observed, though differences between treated and untreated samples were less evident. The Douglas fir examined in this study showed differences in hardness, an 8% reduction in sapwood, and a 28% significant decrease (P < 0.05) in heartwood.
MOR value results showed a decrease of approximately 28% following heat treatment for both species, suggesting MOR was the most sensitive property to heat treatment. Several studies similarly reported decreased MOR in softwood and hardwood species ([41], [32], [18], [3], [47]). Bal ([6]) demonstrated the modification in bending strength (MOR) was due to mass loss following heat treatment, and although it was not possible to measure the mass loss in this experiment, some general comments can be made based on the knowledge that MOR typically exhibits a positive relationship to wood density ([62], [39], [43]). In the present study, the density reduction following heat treatment was not as high as expected, particularly when compared to the observed change in strength. However, the ratio between the mechanical properties and untreated wood density would be expected to change following heat treatment due to chemical degradation of woody components, such as hemicelluloses and cellulose, which play a major role in determining wood properties in relationship to density ([18]). Furthermore, Corsican pine sapwood (516.8 kg m-3) had a higher density than Douglas fir (457.3 kg m-3), therefore a major decrease in Corsican pine sapwood mechanical properties after heat treatment should have been observed due to higher thermal conductivity of denser wood ([5], [6]). The differences detected might be explained by the volatile resin amounts in the two species’ sapwood.
Lower mechanical performance in compression strength is usually found in heat treated hardwoods and softwoods ([3], [28]). In our study, we observed a significant reduction (P < 0.05) in axial compression strength in Douglas fir after heat treatment in both heartwood and sapwood. Corsican pine however, showed significantly higher axial compression strength (4%, P < 0.05) in the treated samples. This is reflected in the Corsican pine bending MOE values, which were also higher in treated samples (12%) and were consistent with other studies ([44], [9], [6]).
Results showed the reduction in mechanical properties due to heat treatment was in some cases lower than that observed in Pinus banksiana ([27]) and in P. nigra ([23], [6]). In our study, the bending MOE was not notably affected by heat treatment in Corsican pine or Douglas fir, particularly when compared with results reported in hardwoods, including aspen ([51]) and birch ([46]).
Special attention must be given to the load-deflection curves (Fig. 4, Fig. 5) when assessing MOR and axial compression strength. Heat-treated Corsican pine exhibited abrupt failures in MOR values when it reached a deformation of approximately 4 mm, and a considerably larger deflection at maximum load for untreated samples. The curve shape typically reflects the behavior of heat-treated wood, which was reported in other wood species ([2]) and ascribed to transverse fracture surfaces of tension-stressed fibers, or transverse ruptures in tracheid cell walls ([2]). Unsal & Ayrilmis ([57]) showed compression strength was reduced by heat treatment, even under relatively low temperature conditions. Many Corsican pine control samples under compression exhibited a residual deformation up to 6 mm (Fig. 4b) after reaching maximum load, whereas the mechanical behavior demonstrated by the load-deformation graph differed in Douglas fir (Fig. 5b). Specifically, the MOR value following the maximum load shows a residual deformation lower than that observed in control (nt) samples (Fig. 5a, Fig. 5c), with the same result observed in compression strength.
Fig. 4 - Load-deformation graph for Corsican pine showing the relationship between MOR (a, c) and axial compression strength (b, d) in treated (t) and untreated specimens (nt).
Fig. 5 - Load-deformation graph for Douglas fir sapwood showing the relationship between MOR (a, c) and axial compression strength (b, d) in treated (t) and untreated specimens (nt).
Sapwood and heartwood results before and after treatment requires special consideration. For example, heat treatment appeared to mitigate the differences between the two tissues (heartwood and sapwood) compared to the physical and mechanical properties of non-treated wood material, with the exception of the compression strength ([14]). Heat treatment also affected heartwood specific density and volumetric shrinkage; however, bending MOE, compression strength, and hardness was primarily impacted in sapwood. It was notable that bending strength and MOE decrease in sapwood was observed, but a significant reduction was not detected. This result indicated it is possible to obtain an improvement in dimensional stability in Douglas fir sapwood, with a far less substantial loss of mechanical performance than would otherwise be expected.
Few studies have evaluated the different behaviors of sapwood and heartwood after thermowood treatment. A recent paper examined EMC in Turkey oak ([50]), however the outcome of this and other studies were not congruent with our results. In fact, Burgos & Rolleri ([13]) observed increased shrinkage in heat-treated Douglas fir wood, with decreased extractives, although the result might be comparable, as Douglas fir sapwood contains fewer extractives than heartwood. A separate examination of radial and tangential directions in the present study indicated ASE
was very similar to shrinkage values, with the exception of heartwood vs. sapwood radial shrinkage. Sapwood substances might be chemically modified into new polyphenols, which could affect the expected physical and mechanical behavior of sapwood ([1]). A linear regression analysis was performed between density and shrinkage for Corsican pine and Douglas fir, and results showed that both species exhibited very low R2 values (Fig. 6, Fig. 7). It can be hypothesized that the absence of a relationship between the variables could be due to juvenile wood in the conifer tissue, which weakened the mechanical properties as compared with mature wood. Increased shrinkage can be explained by orientation of the microfibril angle; and decreased wood density did not make as great a contribution ([61], [31], [6]). Following treatment, density did not exhibit an increased relationship to physical properties (Fig. 6), and its relationship to mechanical properties was reduced (Fig. 7). Increased understanding can be inferred from the correlation coefficient between density and the physical and mechanical properties (Tab. 2), where the correlation was often significant in Corsican pine. However, Douglas fir showed an absence of significant results, with the exception of sapwood density-compression strength and density-anisotropy coefficients. In addition, a remarkable result showed the correlation coefficient between density, MOR, and radial shrinkage was increased by heat treatment.
Fig. 6 - Linear regression between density and the measured physical properties in treated (t) and untreated (nt) specimens for Corsican pine (CP) and Douglas fir (D).
Fig. 7 - Linear regression between wood density and mechanical properties in treated (t) and untreated (nt) samples for Douglas fir. The Douglas fir sapwood and heartwood specimens were grouped together. (MOR): bending strength; (MOE): bending modulus of elasticity.
Tab. 2 - Pearson’s correlation analysis between density and physical-mechanical properties. (MOR): bending strength; (σc): axial compression strength; (HB): Brinell hardness; (MOE): bending modulus of elasticity; (β
V): volumetric shrinkage; (β
r): radial shrinkage; (β
t): tangential shrinkage; (Ψ
): coefficient of anisotropy; (CP): Corsican pine; (D-S): Douglas fir sapwood; (D-H): Douglas fir heartwood, (nt): untreated; (t): treated. (*), (**), (***): levels of significance between treated (t) and untreated (nt) specimens at P < 0.05, P < 0.01, and P < 0.001, respectively using the Bonferroni’s test.
Parameter | MOR (MPa) |
σ12 (MPa) |
HB (MPa) |
MOE (MPa) |
β V max(%) |
β r max(%) |
β t max(%) |
Ψ
|
---|---|---|---|---|---|---|---|---|
CP nt (n=40) | 0.385 | 0.767*** | 0.135 | 0.468* | 0.610*** | 0.554** | 0.487* | -0.381 |
CP t (n=40) | 0.636*** | 0.695*** | 0.127 | 0.358*** | 0.630*** | 0.726*** | 0.441 | -0.392 |
D-S nt (n=12) | 0.231 | 0.643*** | 0.353 | 0.416 | -0.143 | 0.400 | -0.540 | -0.885*** |
D-S t (n=12) | 0.274 | 0.603** | 0.264 | 0.460 | -0.347 | 0.358 | -0.649 | -0.681 |
D-H nt (n=28) | 0.152 | 0.418** | 0.209 | 0.228 | 0.190 | 0.331 | 0.032 | -0.344 |
D-H t (n=28) | 0.245 | 0.284 | 0.365 | 0.150 | 0.236 | 0.356 | 0.143 | -0.390 |
The most marked result, however, was the change in the correlation trend between density and radial shrinkage after heat treatment. The hornification process might serve to explain this result, which in spruce promoted irreversible hydrogen bonding between wood elements during drying that accompanied heat treatment ([11]). Hornification is a process term often applied to wood pulp when polymeric structures in lignocellulosic materials stiffen during drying ([20]). Hornification in heat-treated wood results in a mass reduction without decreasing mechanical properties, and in spruce studies demonstrated increased MOR and MOE up to a 3% mass loss, depending on the relative humidity of the drying process ([10], [11], [12]). Although mass loss could not be determined in our experiment, based on the slight decrease in Corsican Pine density (≈2%), we speculated it was at least within the range determined by Borrega & Kärenlampi ([10]).
In untreated samples, density was strongly related to MOR and compression strength. In comparison, heat treatment did not substantially effect the correlation between density and MOE, which can be explained by the hornification mechanism, which in spruce dominated the influence of mass loss on strength and stiffness ([11]). Epmeier & Kliger ([15]) previously demonstrated the correlation between bending MOE and density in P. sylvestris was significant in raw material, but was lost following wood modification, a characteristic also observed in Cedrus libani sapwood ([5]). This was confirmed in our Corsican pine sapwood, and can be considered an advantage because such mass loss does not have a pronounced effect on mechanical strength.
Density impacted compression strength in untreated Douglas fir sapwood and heartwood specimens. Following treatment, the correlation was maintained and significant compression strength results were revealed, but values were reduced. In Corsican pine sapwood, heat treatment reduced the density and MOR/MOE correlation. It also decreased the effect on shrinkage values, although the results remained significant.
Conclusion
Corsican pine heat treatment significantly reduced the anisotropy coefficient and shrinkage, whereas in Douglas fir sapwood specific density was most impacted by heat treatment. Overall, P. nigra subsp. laricio results suggested this material has more potential as a valuable wood product than biomass for energy or to manufacture pallets, which indicates silvicultural options should be explored. Treatment had a decreased effect on wood density and subsequent physical and mechanical properties, which could promote silviculture with high growth rates. In fact, even with a wider ring width and increased amount of earlywood, and therefore lower density, the reduction does not noticeably compromise the physical and mechanical characteristics of the treated wood. Douglas fir results indicated heat treatment was notably less beneficial in terms of mechanical performance. Furthermore, this species has naturally good wood durability, and the increased heartwood presence was reported to be associated with high growth rates. These characteristics therefore discourage further investment into P. menziesii heat processing, as treatment is only required if the need is evident for more dimensional stabilization in specific wood products.
References
Gscholar
Gscholar
Gscholar
Gscholar
Gscholar
Online | Gscholar
Gscholar
Gscholar
Gscholar
Gscholar
Gscholar
Gscholar
Gscholar
Gscholar
Gscholar
Authors’ Info
Authors’ Affiliation
DAFNE, University of Tuscia, Viterbo (Italy)
DIBAF, University of Tuscia, Viterbo (Italy)
Margaritelli Farm, Perugia (Italy)
Agroselviter, Torino University (Italy)
GEESAF, Firenze University (Italy)
Corresponding author
Paper Info
Citation
Romagnoli M, Cavalli D, Pernarella R, Zanuttini R, Togni M (2015). Physical and mechanical characteristics of poor-quality wood after heat treatment. iForest 8: 884-891. - doi: 10.3832/ifor1229-007
Academic Editor
Giacomo Goli
Paper history
Received: Dec 31, 2013
Accepted: Apr 01, 2015
First online: May 22, 2015
Publication Date: Dec 01, 2015
Publication Time: 1.70 months
Copyright Information
© SISEF - The Italian Society of Silviculture and Forest Ecology 2015
Open Access
This article is distributed under the terms of the Creative Commons Attribution-Non Commercial 4.0 International (https://creativecommons.org/licenses/by-nc/4.0/), which permits unrestricted use, distribution, and reproduction in any medium, provided you give appropriate credit to the original author(s) and the source, provide a link to the Creative Commons license, and indicate if changes were made.
Web Metrics
Breakdown by View Type
Article Usage
Total Article Views: 46975
(from publication date up to now)
Breakdown by View Type
HTML Page Views: 39689
Abstract Page Views: 2486
PDF Downloads: 3701
Citation/Reference Downloads: 22
XML Downloads: 1077
Web Metrics
Days since publication: 3264
Overall contacts: 46975
Avg. contacts per week: 100.74
Article Citations
Article citations are based on data periodically collected from the Clarivate Web of Science web site
(last update: Feb 2023)
Total number of cites (since 2015): 21
Average cites per year: 2.33
Publication Metrics
by Dimensions ©
Articles citing this article
List of the papers citing this article based on CrossRef Cited-by.
Related Contents
iForest Similar Articles
Research Articles
The physicomechanical and thermal properties of Algerian Aleppo pine (Pinus halepensis) wood as a component of sandwich panels
vol. 15, pp. 106-111 (online: 21 March 2022)
Short Communications
Influence of thermo-vacuum treatment on bending properties of poplar rotary-cut veneer
vol. 10, pp. 161-163 (online: 13 June 2016)
Research Articles
Physical, chemical and mechanical properties of Pinus sylvestris wood at five sites in Portugal
vol. 10, pp. 669-679 (online: 11 July 2017)
Research Articles
Mechanical and physical properties of Cunninghamia lanceolata wood decayed by brown rot
vol. 12, pp. 317-322 (online: 06 June 2019)
Research Articles
Behavior of pubescent oak (Quercus pubescens Willd.) wood to different thermal treatments
vol. 8, pp. 748-755 (online: 16 February 2015)
Research Articles
Heat treatment of poplar plywood: modifications in physical, mechanical and durability properties
vol. 16, pp. 1-9 (online: 09 January 2023)
Technical Advances
Technical properties of beech wood from aged coppices in central Italy
vol. 8, pp. 82-88 (online: 04 June 2014)
Research Articles
Validation of visual and machine strength grading for Italian beech with additional sampling
vol. 14, pp. 260-267 (online: 29 May 2021)
Research Articles
Acoustic evaluation of wood quality with a non-destructive method in standing trees: a first survey in Italy
vol. 10, pp. 700-706 (online: 17 July 2017)
Research Articles
Testing common hornbeam (Carpinus betulus L.) acetylated with the Accoya method under industrial conditions
vol. 10, pp. 948-954 (online: 19 December 2017)
iForest Database Search
Search By Author
Search By Keyword
Google Scholar Search
Citing Articles
Search By Author
Search By Keywords
PubMed Search
Search By Author
Search By Keyword