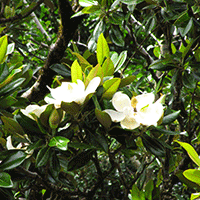
Magnolia grandiflora L. shows better responses to drought than Magnolia × soulangeana in urban environment
iForest - Biogeosciences and Forestry, Volume 13, Issue 6, Pages 575-583 (2020)
doi: https://doi.org/10.3832/ifor3596-013
Published: Dec 07, 2020 - Copyright © 2020 SISEF
Research Articles
Abstract
Drought tolerance is becoming an increasingly important criterion for the selection of tree species, especially in urban areas characterized by low water availability. Apart from drought tolerance, the introduction of non-native species should be considered for new planting programs under such conditions to enhance the resilience of urban forests. The present study is aimed at evaluating the in situ physiological responses of Magnolia grandiflora and Magnolia × soulangeana to severe drought that frequently occurs in urban environments in the Southeastern Europe. Transpiration rate, stomatal conductance, intercellular CO2 concentration, water-use efficiency and intrinsic water-use efficiency showed notable differences both between species and between the measured periods (wet and dry). Among the chlorophyll a fluorescence parameters, effective photochemical quantum yield of PS II, quantum yield of light-induced non-photochemical fluorescence quenching, quantum yield of non-regulated heat dissipation, fluorescence emission and index of susceptibility of leaves to light stress revealed significant differences both between the two species and the periods of measurements. The reduction of net photosynthesis in both magnolia species occurs as the result of non-stomatal limitation obtained by the reduction of electron transport rate coupled with simultaneous increase in intercellular CO2 concentration. Moreover, M. grandiflora was the species less vulnerable to water shortage conditions, while M. soulangeana exhibited a photosynthetic capacity sensitive to drought-induced stress. M. grandiflora can therefore be considered as a promising alternative to M. soulangeana for urban sites under the predicted climate change scenarios.
Keywords
Chlorophyll a Fluorescence, Drought, Leaf Gas Exchange, Photosynthesis, Urban Tree Selection
Introduction
Urban ecosystems pose multiple challenges for trees to cope with ([50]). Among the various environmental factors occurring in urban settlements, drought was being recognized as one of the most frequent and threatening constraint, resulting from the combination of water deficit and high temperatures ([29]), thereby causing serious damages and a premature mortality of urban trees ([59]). Apart from directly influencing the tree by inhibiting its growth through reduced photosynthetic rates, drought has additional indirect impacts as well, e.g., by predisposing trees to pathogens ([12]) and pollutants ([9]).
The severity of the negative effects on plants is related to the intensity, duration, and course of progression of drought stress ([7]). In plants subjected to mild to moderate drought, stomatal control is the major physiological mechanism preventing excessive water loss, causing an overall reduction of photosynthesis and consequently the inhibition of plant growth ([61]). Under more severe drought stress, metabolic and biochemical limitations may occur and reduce CO2 assimilation rates; these processes include the inhibition of Rubisco enzyme activity, damage of chlorophyll or photosystems, and a decrease in the capacity to generate ATP ([6]).
Under drought conditions, PSII photochemistry of plants can be affected, and parameters of chlorophyll a fluorescence were shown to be rapid, highly sensitive and non-destructive tool for detecting species, genotypes and provenances with tolerant or susceptible behavior ([8], [56]). While early phases of drought were observed to impose slight changes on PSII, more progressive drought was noted to cause a depletion of PSII core and inhibit electron transport chain from the donor side of PSII to PSI, indicating non-stomatal inhibition of photosynthesis ([23]).
The responses of plants in such conditions have been observed to be species-specific ([51], [55]) due to differences in leaf morphology and physiology. For example, a significant decrease in photosynthetic activity in Ginkgo biloba L. was detected, which resulted in higher sensitivity to drought compared to Fraxinus chinensis Roxb. ([59]). Furthermore, several studies have evidenced that provenances and species originating from drier climate perform better under drought condition ([42], [58]). For instance, investigation of the drought tolerance of Quercus ilex L., Q. pubescens Willd., and Q. robur L., showed that the Mediterranean oak species, despite a reduction of light-saturated net photosynthesis ([10]), maintained higher photosynthetic activity compared to Central-European oak species ([19]).
In the South-East Europe the climate scenarios, according to the medium-high emission baseline (A1B - [26]), project an increase of average air temperature from 2.4 °C up to 3.4 °C, along with a 30% reduction of average rainfall until the end of the 21st century, and the negative impact of drought will very likely become even more heightened. Under such harsh conditions, the physiology of trees will be affected, and this, in turn, could further impact the broad range of benefits they provide for humans ranging from shading streets to carbon sequestration ([50]).
To mitigate the negative effects of climate change, the evaluation of drought tolerance of tree species should be mainly contemplated for the selection plantation in urban ecosystems ([57], [50]). In this context, the introduction of non-native species should be considered in new planting programs, alongside the use of drought-tolerant autochthonous species, as it has been noted that high species diversity contributes to increasing resilience and reducing the maintenance requirements of urban forests under abiotic and biotic challenges ([28]).
The Magnolia genus of the Magnoliaceae family includes 224 ornamental species ([25]), and its planting in urban areas has increased significantly over the past decade. In Southeast Europe, Magnolia × soulangeana Soul.-Bod., a deciduous hybrid of Magnolia denudata Desr. and Magnolia liliflora Desr., is a very common species in urban parks, while Magnolia grandiflora L., an evergreen species native to the southern United States ([11]), is encountered less often. However, as the distribution of forest tree species is generally expected to shift northwards in response to climate warming ([39]), a similar expansion of the distribution of M. grandiflora could be expected in the future, increasing its frequency in Southeast Europe as well.
As both of these species have a high ornamental value, most literature deals mainly with their aesthetic qualities ([11]). However, the selection of tree species for urban ecosystems should include other important factors, such as the microclimatic conditions of the respective site and the physiological performance of the species concerning stress factors ([57]). Notably, understanding the interaction between the physiology of plants and their surrounding environment is essential for establishing better management strategies to alleviate stress conditions for the species ([50]), especially in urban areas where these stressors are even more amplified and combined. Despite the wide range of research possibilities posed in urban environments, the physiological performance of trees, including the Magnolia genus, remains scarcely explored and understood ([59]). To support tree selection for urban areas in temperate climate and prevent mistakes in planning, which could result in high ecological and economic costs, the Citree database was developed ([57]). However, Vogt et al. ([57]) noted that additional field experiments should be included in the database, especially for aspects that have not been thoroughly investigated, such as the drought tolerance of species.
The present study was aimed to investigate the physiological response to naturally occurring severe drought of M. grandiflora and M. soulangeana planted in the urban area of Novi Sad, Serbia, South-East Europe. Tree responses were determined through accurate, reliable and non-invasive tools by estimating: (i) the photosynthetic function of plants under drought, to analyze the photosynthetic processes and detect water efficient and stress tolerant species ([60]); (ii) the effects of stress on photochemistry ([43]). We hypothesized that drought might (1) negatively affect leaf gas exchange and chlorophyll a fluorescence in both magnolia species, by indicating tolerant and/or vulnerable drought behavior, (2) induce the non-stomatal regulations with consequent inhibition of net photosynthesis, and (3) less affect the photosynthetic performance of a species from drier climate than of a more humid site.
Material and methods
Study area and species
The present study was conducted in the urban green space of Novi Sad, the second largest city of Serbia (45° 15′ 10″ N, 19° 50′ 53″ E), characterized as a pocket park ([45]). At the studied locality, ten adult M. grandiflora L. and M. soulangeana Soul.-Bod. trees were chosen for the evaluation of physiological responses. Tree selection was based on visual impression, namely all chosen trees were vigorous, without dying branches, insect infestation, or mechanical damage ([35]). M. grandiflora trees were planted in a single linear row alongside a walkway in direction of West-East with a planting space of 3 m between them. On the other hand, M. soulangeana trees were arranged in double linear rows alongside a walkway in direction of North-South and a planting space of 4 m. Both species were planted in a common green space covered with grass and in full sunlight. For assessing the in situ physiological status of the two magnolia species in urban environment, leaf gas exchange and chlorophyll a fluorescence parameters were measured in wet (18th of July 2019) and drought (31th of August 2019) periods.
Meteorological data
Monitoring of mean air temperature (°C) and daily precipitation (mm) was carried out at the nearby meteorological station Rimski Šančevi (45° 20′ N, 19° 51′ E, altitude 84 m a.s.l.) from May 1 to September 4, 2019. The soil water potential (SWP) was measured using calibrated gypsum blocks (Delmhorst Inc., Towaco, NJ, USA) positioned at 50 cm soil depth, with measurements at 30 s intervals stored in a MicroLog SP3® datalogger (EMS, Brno, Czech Republic).
The wet period measurement was performed on July 18, 2019 after 10 days of SWP values below -0.4 MPa and mean air temperatures ranging between 19.2 °C and 22.2 °C, with the maximum temperature never reaching 30 °C. The drought period measurement was performed on the August 31, 2019 after six days of SWP values below -1.4 MPa and mean air temperatures between 25.8 °C and 26.9 °C, with the maximum air temperature exceeding 30°C (characterized as severe drought period according to [31] - Fig. 1).
Fig. 1 - Mean daily air temperatures (°C), total daily precipitation (mm) and soil water potential (MPa) during the period from May 1 to September 4, 2019.
Leaf gas exchange measurement
The measurements of net photosynthesis (A, μmol m-2 s-1), transpiration rate (E, mmol m-2 s-1), stomatal conductance (gs, mmol m-2 s-1), and intercellular CO2 concentration (Ci, μmol mol-1) were taken using a CIRAS-3® portable photosynthesis system (PP Systems, Amesbury, MA, USA). In order to minimize differences in light exposure, exposed, outward-facing leaves on exterior branches in the upper reaches of the trees were chosen. During the measurements, the photosynthetic active radiation (PAR) was set to 1000 μmol m-2 s-1 while humidity, temperature, and the concentration of CO2 depended on the ambient conditions of study site. Following leaf stabilization in the CIRAS-3 chamber, the abovementioned parameters were recorded from 9:00 a.m. to 11:00 a.m. Water use efficiency (WUE, μmol mmol-1) was computed as the ratio of A to E ([17]), and intrinsic water-use efficiency (WUEi, μmol mol-1) was derived as the ratio of A to gs ([18]). The leaf gas exchange parameters were determined for all ten plants of each species, with measurements taken in the order of 5 specimens of M. grandiflora followed by five specimens of M. soulangeana, etc. The same leaves were subsequently used for assessing the pulse amplitude modulated chlorophyll a fluorescence, with three replications per leaf.
Pulse amplitude modulated (PAM) fluorescence measurements
The photosynthetic activity of PSII was measured using a PAM-2500® portable chlorophyll fluorometer (Walz GmbH, Effeltrich, Germany) between 9:00 and 11:00 a.m. Before taking the measurements, the selected leaves were dark-acclimated for 15 minutes using leaf clips. The rapid light curves (RLC) were derived using nine increasing actinic illumination steps (0, 144, 274, 477, 788, 1163, 1389, 2018, 2443 μmol e m-2 s-1). The duration of each actinic illumination step was 10 s, and the steps were separated by a white saturating flash of ~3000 μmol m-2 s-1 lasting 0.8 s. In addition, the studied PAM fluorescence parameters were computed (Tab. 1). Rapid light-response curves of chlorophyll a fluorescence were constructed by plotting the ФPSII, Y(NPQ), Y(NO), (1-qP)/NPQ, qP and ETR versus the increasing actinic irradiance (from 1 to 2443 μmol e m-2 s-1 - [56]). The RLCs were quantified as follows: (i) maximal electron transport rate ETRmax (µmol e- m-2 s-1) for RLCETR; ETRmax was calculated automatically according to internal equation ([14]) and reflects the light-saturated capacity of the sample; (ii) the area below the curve for RLCΦPSII; (iii) the value records at high light intensity (2443 µmol e- m-2 s-1) for RLCNPQ, RLCY(NPQ), RLCY(NO), RLC(1-qP)/NPQ and RLCqP ([43]).
Tab. 1 - The studied computed pulse amplitude modulated fluorescence parameters.
Abbrev. | Equation | Definition of the derived parameters |
---|---|---|
ΦPSII | Fm′-Ft/Fm′ | Effective photochemical quantum yield of PS II ([21]) |
Y(NPQ) | Ft/Fm′- Ft/Fm | Quantum yield of light-induced non-photochemical fluorescence quenching ([22]) |
Y(NO) | Ft/Fm | Quantum yield of non-regulated heat dissipation and fluorescence emission ([22]) |
(1-qP)/NPQ | (1-qP)/NPQ | Index of susceptibility of leaves to light stress ([36]) |
qP | (Fm′-Ft)/(Fm′-Fo′) | Coefficient of photochemical quenching ([49] as formulated by [54]) |
ETR | PAR · 0.84 · 0.5 | Rate of electron transport ([21]) |
Statistical analysis
Statistical analysis of the leaf gas exchange and chlorophyll a fluorescence parameters was conducted using Statistica v. 13 ([53]) and R v. 3.6.3 (R Core Team, Austria). The differences between the two parameters were tested using two-way ANOVA, with the period (wet or dry) and species (M. grandiflora and M. soulangeana) set as fixed factors. The following model was used (eqn. 1):
where yijk represents the measured value of the phenotype, Pi is the effect of the ith period, Sj is the effect of the jth species, Pi × Sj is the interaction between the period and the species, and εijk is the experimental error. Significant differences between the means were evaluated using Tukey’s honestly significant difference (HSD) test with P ≤ 0.05. Prior to the statistical analysis, the normality of data distribution was tested, with the transformation considered necessary for several parameters. All parameters were shown in the form of a bar diagram with means ± standard errors drawn by Statistica v. 13 ([53]).
Results
Leaf gas exchange
Leaf gas exchange parameters were significantly affected by environmental conditions in the considered period and site (Tab. 2). A, Ci, WUE, and WUEi significantly changed between species. Furthermore, the interaction of species × period exhibited a significant effect on all observed leaf gas exchange parameters except for A. These results point to a different drought response of the examined species.
Tab. 2 - Results of two-way ANOVA showing the effects of species (S), period (P), and their interaction (S × P) on the observed leaf gas exchange parameters. (A): net photosynthetic rate (μmol m-2 s-1); (E): rate of transpiration (mmol m-2 s-1); (gs); stomatal conductance (mmol m-2 s-1); (Ci): intercellular CO2 concentration (μmol mol-1); (WUE): water use efficiency (μmol mmol-1); (WUEi): intrinsic water use efficiency (μmol mol-1); (df): degrees of freedom.
Source of variation |
df | Stat | A | E | gs | Ci | WUE | WUEi |
---|---|---|---|---|---|---|---|---|
Species (S) | 1 | F | 101.9 | 2.60 | 3.50 | 41.3 | 30.6 | 23.6 |
P | <0.001 | 0.115 | 0.070 | <0.001 | <0.001 | <0.001 | ||
Period (P) | 1 | F | 140.4 | 9.10 | 12.7 | 70.5 | 27.4 | 16.4 |
P | <0.001 | <0.05 | <0.001 | <0.001 | <0.001 | <0.001 | ||
S × P | 1 | F | 0.44 | 5.60 | 8.30 | 5.80 | 5.10 | 9.50 |
P | 0.510 | <0.05 | <0.05 | <0.05 | <0.05 | <0.05 | ||
Error | 36 | - | - | - | - | - | - | - |
Total | 39 | - | - | - | - | - | - | - |
Drought significantly reduced A and WUE, and increase Ci in both species (Fig. 2a, Fig. 2e, Fig. 2d). In detail, M. grandiflora decreased E and gs (reduction of 38.2% and 47.0%, respectively), whereas M. soulangeana maintained stable but overall low E and gs values (reduction of only 6.1% and 7.6%, respectively) during the drought period (Fig. 2b, Fig. 2c). M. grandiflora exhibited a higher decrease in E and gs, while M. soulangeana showed generally weak performance under both measured periods. Furthermore, M. soulangeana exhibited significantly lower values of WUEi (reduction of 67.65%) during drought in comparison to the wet period, while WUEi values in M. grandiflora were not significantly changed by severe drought (reduction of only 7.47% - Fig. 2f).
Fig. 2 - Leaf gas exchange parameters of M. grandiflora and M. soulangeana measured during the wet period and a drought period. (a) net photosynthetic rate (A, μmol m-2 s-1); (b) rate of transpiration (E, mmol m-2 s-1); (c) stomatal conductance (gs, mmol m-2 s-1); (d) intercellular CO2 concentration (Ci, μmol mol-1); (e) water use efficiency (WUE, μmol mmol-1); (f) intrinsic water use efficiency (WUEi, μmol mol-1). All values are presented as means ± standard errors (n = 10). Different letters indicate significant differences (p ≤ 0.05) between the values after Tukey’s HSD test.
Pulse amplitude modulated (PAM) fluorescence
The result of two-way ANOVA showed significant differences in ΦPSII, (1-qP)/NPQ, qP and ETRmax among the two magnolia species, while no significant changes were detected for Y(NPQ) and Y(NO) (Tab. 3). The analysis of the period (wet and dry) exhibited significant effect on all observed chlorophyll a fluorescence parameters, except for (1-qP)/NPQ. Furthermore, the interaction of the species and period significantly influenced ΦPSII, Y(NPQ), Y(NO) and (1-qP)/NPQ parameters, while no significant effect was observed on qP and ETRmax.
Tab. 3 - Results of two-way ANOVA showing the effect of species, period, and their interaction (species × period) on the observed parameters of chlorophyll a fluorescence at high light intensity (2443 μmol e m-2 s-1). (ΦPSII): effective photochemical quantum yield of PS II (relative units); (Y(NPQ)): quantum yield of light-induced non-photochemical fluorescence quenching (relative units); (Y(NO)): quantum yield of non-regulated heat dissipation and fluorescence emission (relative units); ((1-qP)/NPQ): index of susceptibility of leaves to light stress (relative units); (qP): coefficient of photochemical quenching (relative units); (ETRmax): maximum electron transport rate (µmol e m-2 s-1); (df): degrees of freedom.
Source of variation |
df | Stat | ΦPSII | Y(NPQ) | Y(NO) | (1-qP)/NPQ | qP | ETRmax |
---|---|---|---|---|---|---|---|---|
Species (S) | 1 | F | 7.66 | 2.09 | 2.22 | 10.1 | 96.6 | 21.0 |
P | <0.01 | 0.157 | 0.144 | <0.01 | <0.001 | <0.001 | ||
Period (P) | 1 | F | 6.83 | 17.7 | 5.14 | 0.58 | 56.2 | 24.9 |
P | <0.05 | <0.001 | <0.05 | 0.45 | <0.001 | <0.001 | ||
S × P | 1 | F | 11.2 | 52.1 | 37.8 | 27.0 | 2.01 | 0.18 |
P | <0.01 | <0.001 | <0.001 | <0.001 | 0.166 | 0.669 | ||
Error | 36 | - | - | - | - | - | - | - |
Total | 39 | - | - | - | - | - | - | - |
Observing the RLC of ΦPSII, M. grandiflora showed higher values (until 477 μmol e m-2 s-1) during drought compared to wet period, after which it has declined, while M. soulangeana exhibited lower values in drought, throughout all observed light intensities (Fig. 3a). Drought induced reduction of ΦPSII was observed in M. soulangeana, while no differences were found in M. grandiflora (Fig. 4a). In case of Y(NPQ), M.grandiflora showed higher values, while M. soulangeana lower values during drought (Fig. 3b). The values at high light intensity of RLC showed proportionally greater increase of Y(NPQ) during drought period in M. grandiflora, compared to the decrease for M. soulangena.
Fig. 3 - Chlorophyll a fluorescence parameters generated by RLCs as a function of photosynthetically active radiation (PAR, µmol e m-2 s-1) measured in M. grandiflora and M. soulangeana during the wet period (blue lines) and the drought period (red lines). (a) Effective photochemical quantum yield of PS II (ΦPSII, relative units); (b) quantum yield of light-induced non-photochemical fluorescence quenching (Y(NPQ), relative units); (c) quantum yield of non-regulated heat dissipation and fluorescence emission (Y(NO), relative units); (d) index of susceptibility of leaves to light stress ((1-qP)/NPQ, relative units); (e) coefficient of photochemical quenching (qP, relative units); (f) electron transport rate (ETR, µmol e- m-2 s-1).
Fig. 4 - The parameters of chlorophyll a fluorescence at high light intensity (2443 μmol e m-2 s-1) for M. grandiflora and M. soulangeana during the wet period and the drought period. (a) Effective photochemical quantum yield of PS II (ΦPSII, relative units); (b) quantum yield of light-induced non-photochemical fluorescence quenching (Y(NPQ), relative units); (c) quantum yield of non-regulated heat dissipation and fluorescence emission (Y(NO), relative units); (d) index of susceptibility of leaves to light stress ((1-qP)/NPQ, relative units); (e) coefficient of photochemical quenching (qP, relative units); (f) maximum electron transport rate (ETRmax, µmol e m-2 s-1). All values are presented as means ± standard errors (n = 10). Different letters indicate significant differences (p ≤ 0.05) between means after Tukey’s HSD test.
Furthermore, Y(NO) values of M. grandiflora were lower than in M. soulangeana during drought (Fig. 3c). According to the highest light intensities assessed, the reduction of Y(NO) in M. grandiflora was statistically significant, while the increase in M. soulangeana was shown to be less significant (Fig. 4c). Considering (1-qP)/NPQ, M. grandiflora exhibited higher values (until 477 μmol e m-2 s-1) during drought period compared to wet period, after which it has declined, while M. soulangeana showed increased values in drought over the irradiance range (Fig. 3d, Fig. 4d). A reduction of qP was observed in both magnolia species under severe drought as compared to the wet period (Fig. 3e, Fig. 4e). However, the reduction in values of the parameter qP in M. grandiflora during drought were similar to the values of M. soulangeana during wet period. Furthermore, drought reduced ETRmax in M. soulangeana, while in M. grandiflora higher values were found until light intensity of 788 μmol e m-2 s-1 compared to wet period, after which it showed the opposite ranking (Fig. 3f). At high intensities, the reduction of ETRmax was found in both magnolia species, where M. grandiflora during drought and M. soulangeana during wet period showed the same range of values (Fig. 4f).
Discussion
The present study showed the adversely effects of the naturally occurring severe drought period on net photosynthetic rate (A) in both magnolia species grown in urban environment. Even though A was significantly reduced in both species, M. grandiflora managed to maintain higher rates. This is advantageous on multiple counts, allowing an increased metabolic activity like the synthesis of sugars, amino acids, proteins, nucleic acids and lipids, all of them being essential for the growth and repair of damaged tissues ([40]). Similarly to our findings, many studies observed significantly reduced A rates in urban areas, which are characterized by limited water availability ([40], [37]) and sealed surfaces ([59]).
The reduction of A under drought has been addressed to the protective mechanism of plants to avoid damage due to dehydration and hydraulic failure ([37]). During water shortage the roots are unable to penetrate in the deeper layers to find water resources and are left with low water supply, especially in urban areas where the soil is more compacted than in natural sites ([33]). The prompt response of stomata to environment constraints through stomatal regulation, the disruption in the CO2 supply caused by stomatal closure, is being addressed as the main cause of decreased photosynthesis under mild to moderate drought ([61]).
A reduction of gs and increase of Ci were observed during severe drought conditions for both magnolia species examined in the present study. As drought becomes more severe and long in time an increase in Ci values was also reported, evidencing the predominance of non-stomatal limitation to A ([5], [15], [6]). The reduction of A under prolonged water shortage was related to diffusive (reduced mesophyll conductance) and metabolic (photochemical and enzymatic limitations) processes ([20]). Metabolic limitations to A were most frequently associated with the reduction of Rubisco activity and regeneration, resulting from reduced ATP or NADPH supply (regarded as photochemical limitation), or as the consequence of low enzymatic activity of the Polymerase Chain Reaction cycle ([30]). Prolonged periods of water deficit can also increase the oxygenase activity of Rubisco, reducing consequently the carboxylation efficiency, causing in such a way another commonly encountered non-stomatal limitation to A ([34]). In addition, the inhibition of A might be attributed to the reduction of chlorophyll content, as a common symptom of oxidative stress under severe drought condition, resulting from pigment photooxidation and photosynthetic pigment degradation ([27]). A marked increase in Ci under more progressed drought period and the proportional decrease in A rate was found to be less significant in comparison to the decline of gs in M. grandiflora, resulting in high WUE values (according to [41]). On the other hand, the net photosynthetic rate was more sensitive to soil water shortage than gs in M. soulangeana. Under the studied environmental constrain the significant decrease of WUEi in M. soulangeana was driven by a pronounced decline in A, while M. grandiflora maintained high WUEi values. Species characterized with higher WUEi values have specific water-saving strategy to avoid greater water losses and maintain higher assimilation rates, particularly important under the conditions of predicted climate changes ([3]). Taking into account the effectiveness of WUEi as an indicator of drought sensitivity, the importance of identification of traits that could be utilized to improve WUEi and hence enhance drought adaptation in breeding programmes was noted ([52]).
M. grandiflora exhibited a higher ability to tolerate the prolonged period of drought compared to M. soulangeana, by maintaining greater values of A and WUEi. This assumption is further supported by the slightly low rate of transpiration (E) in M. grandiflora, as noted for drought tolerant genotypes ([46]). For instance, isohydric species show a lower water transpiration by retaining a greater amount of water, and then they are believed to be less vulnerable to drought ([2]). As the process of E is facilitated through the stomata, stomatal control under drought condition is of key importance for a successful adaptation to environmental changes ([61]).
By maintaining higher ΦPSII and ETRmax values during water shortage, M. grandiflora exhibited a higher efficiency in transporting electrons and potentially could fix more CO2 in comparison to M. soulangeana, as reported by Estrada et al. ([16]) in drought-tolerant blueberry genotypes. In addition, high values of ETR parameter give a great advantage in the reduction of ROS in photosynthesis and in maintaining normal physiological functions ([32]). On the other hand, the decrease of ΦPSII in M. soulangeana during drought period indicated the physiological regulation of electron transport by increasing excitation energy quenching process in the PSII antennae, according to Ashraf & Harris ([1]). Furthermore, the decrease of Y(II) points to the decrease in the carboxylation efficiency, which affects the rate of consumption of ATP and NADPH ([44]).
Environmental stresses, such as water shortage and high light intensities, cause the saturation of electron transfer chain and the increase of the accumulation of proton, resulting in increase of Y(NPQ) parameter ([60]). High values of Y(NPQ) under drought found in M. grandiflora could be addressed to the good ability of plants to mitigate the negative effects of the severe drought at the chloroplast level ([24]). Consequently, the decline of Y(NPQ) in M. soulangeana indicated a loss of capacity to generate the trans-thylakoid proton gradient in chloroplast. A possible explanation for this phenomenon could be associated with the damage/decrease of PSI activity ([4]). The simultaneous reduction of Y(NPQ) and increase of Y(NO) under drought in M. soulangeana further suggested the damage of PSII instead of the protective mechanism under the observed abiotic stress ([47]). In accordance with these results, the increase of water deficit leads to damaging of the oxygen-evolving complex of PSII ([47]). The inhibited synthesis and accelerated degradation of D1 proteins causes further separation of electron transport complex QB and D1 within chloroplasts and results in the inability to accept electrons ([32]).
A pronounced decrease of ΦPSII and qP in M. soulangena suggested that the capability of photochemical conversion and the linear electron flux were both sensitive to the severeness and duration of drought ([60]). Beside a pronounced reduction of ΦPSII and qP, a sharper decline of ETR is a commonly observed characteristic of drought-susceptible genotypes ([48]), and reflects a reduced capability of the transport of electrons to fix CO2. The decrease of photosynthetic electron-transport rates along with lower trans-thylakoid pH gradient resulted in the accumulation of “excess photons” ((1-qP)/NPQ) in M. soulangeana, suggesting that this species is more sensitive to light stress than M. grandiflora ([38]). The observed reduction of qP coupled with the proportional increase Y(NPQ), showed that M. grandiflora was able to maintain the capacity to regulate dissipation of light energy under the observed drought. Moreover, it might be assumed that the xanthophyll’s cycle of the examined species was efficient in providing a vent to the excess electrons flow generated for the increase in light intensity in the chloroplasts ([13]).
Our results highlight the overall higher drought-resistance of M. grandiflora compared to M. soulangeana. The sensitivity of M. soulangeana to drought is in line with the description provided for species selection in Citree, the urban tree database (⇒ https://citree.de/db-names.php?language=en). On the other hand, our findings revealed a drought-tolerant behavior in M. grandiflora that is contradictory to the information in the Citree database. In addition, our findings correspond with the studies reporting high drought tolerance of species and provenances from dry climates under water shortage conditions ([42], [58]). The good adaptation potential of M. grandiflora to severe water deficits was assumed by Sjöman et al. ([51]) for urban street sites in North America and Europe.
Conclusion
This case study supports decision-making processes for the selection of trees in drought-affected urban areas by comparing two common ornamental tree species with similar aesthetic value. Measurements of leaf gas exchange parameters along with chlorophyll a fluorescence showed M. grandiflora as a drought-tolerant species, while M. soulangeana exhibited susceptibility to drought, with a photosynthetic capacity sensitive to drought-induced stress. The decrease of A in both magnolia species were related to non-stomatal factors, as evidenced by the decrease of ETRmax and a simultaneous increase of Ci. Most of the observed parameters of leaf gas exchange, namely E, gs, Ci, WUE, and WUEi were proven to be highly sensitive to drought. In regard to chlorophyll a fluorescence, parameters related to the photochemical dissipation of light energy (ΦPSII) as well as associated with the heat dissipation of light energy (Y(NPQ), Y(NO)) appear to be useful indicators for the selection of drought-tolerant species, and are suggested for application in the assessment of plant responses in urban environments. Considering the predicted future climate change scenarios, M. grandiflora could be a good alternative to M. soulangeana for urban sites. The significance of the present study lies in the evaluation of the in situ physiological responses and selection of appropriate ornamental species for drought-prone urban environments.
List of abbreviations
A: net photosynthetic rate; Ci: intercellular CO2 concentration; E: rate of transpiration; ETR: electron transport rate; gs: stomatal conductance; ΦPSII: effective photochemical quantum yield of PS II; qP: coefficient of photochemical quenching; WUE: water use efficiency; WUEi: intrinsic water use efficiency; Y(NPQ): quantum yield of light-induced non-photochemical fluorescence quenching; Y(NO): quantum yield of non-regulated heat dissipation and fluorescence emission; (1-qP)/NPQ: index of susceptibility of leaves to light stress.
Acknowledgments
This study was financed by the Ministry of Education, Science and Technological Development of the Republic of Serbia (contract number: 451-03-68/2020-14/200197). Furthermore, this work was carried out under the project “Establishing Urban FORest based solutions In Changing Cities” (EUFORICC), supported by the Ministry of Education, University and Research (MIUR) of Italy (PRIN 20173RRN2S).
References
Gscholar
CrossRef | Gscholar
CrossRef | Gscholar
Gscholar
Gscholar
Gscholar
Gscholar
CrossRef | Gscholar
Gscholar
Authors’ Info
Authors’ Affiliation
Department of Fruit Growing, Viticulture, Horticulture and Landscape Architecture, Faculty of Agriculture, University of Novi Sad, Trg Dositeja Obradovića 8, 21000 Novi Sad (Serbia)
Saša Orlović 0000-0002-2724-1862
Lazar Kesić 0000-0003-2643-9727
Srdan Stojnić 0000-0001-5014-7244
Institute of Lowland Forestry and Environment, University of Novi Sad, Antona Cehova 13d, 21000 Novi Sad (Serbia)
Daniel Kurjak 0000-0002-2489-8463
Department of Integrated Forest and Landscape Protection, Faculty of Forestry, Technical University in Zvolen, T. G. Masaryka 24, 960 01 Zvolen (Slovakia)
Department of Agriculture, Food, Environment and Forestry, University of Florence, 50145 Florence (Italy)
Department of Plant Ecophysiology, Institute of Forest Ecology, Slovak Academy of Sciences, Štúrova 2, 960 53 Zvolen (Slovakia)
Department of Forest Growth and Silviculture, Protection Forest and Natural Forest Reserves, Federal Research and Training Centre for Forest, Natural Hazards and Landscape, Seckendorff-Gudent-Weg 8, 1131 Vienna (Austria)
Corresponding author
Paper Info
Citation
Vastag E, Orlović S, Konôpková A, Kurjak D, Cocozza C, Pšidová E, Lapin K, Kesić L, Stojnić S (2020). Magnolia grandiflora L. shows better responses to drought than Magnolia × soulangeana in urban environment. iForest 13: 575-583. - doi: 10.3832/ifor3596-013
Academic Editor
Werther Guidi Nissim
Paper history
Received: Jul 21, 2020
Accepted: Oct 01, 2020
First online: Dec 07, 2020
Publication Date: Dec 31, 2020
Publication Time: 2.23 months
Copyright Information
© SISEF - The Italian Society of Silviculture and Forest Ecology 2020
Open Access
This article is distributed under the terms of the Creative Commons Attribution-Non Commercial 4.0 International (https://creativecommons.org/licenses/by-nc/4.0/), which permits unrestricted use, distribution, and reproduction in any medium, provided you give appropriate credit to the original author(s) and the source, provide a link to the Creative Commons license, and indicate if changes were made.
Web Metrics
Breakdown by View Type
Article Usage
Total Article Views: 37651
(from publication date up to now)
Breakdown by View Type
HTML Page Views: 31312
Abstract Page Views: 3510
PDF Downloads: 2269
Citation/Reference Downloads: 6
XML Downloads: 554
Web Metrics
Days since publication: 1666
Overall contacts: 37651
Avg. contacts per week: 158.20
Article Citations
Article citations are based on data periodically collected from the Clarivate Web of Science web site
(last update: Mar 2025)
Total number of cites (since 2020): 11
Average cites per year: 1.83
Publication Metrics
by Dimensions ©
Articles citing this article
List of the papers citing this article based on CrossRef Cited-by.
Related Contents
iForest Similar Articles
Research Articles
Wintertime photosynthesis and spring recovery of Ilex aquifolium L.
vol. 12, pp. 389-396 (online: 31 July 2019)
Research Articles
Calibration of a multi-species model for chlorophyll estimation in seedlings of Neotropical tree species using hand-held leaf absorbance meters and spectral reflectance
vol. 9, pp. 829-834 (online: 17 May 2016)
Research Articles
Relationships between leaf physiognomy and sensitivity of photosynthetic processes to freezing for subtropical evergreen woody plants
vol. 12, pp. 551-557 (online: 17 December 2019)
Research Articles
Light acclimation of leaf gas exchange in two Tunisian cork oak populations from contrasting environmental conditions
vol. 8, pp. 700-706 (online: 08 January 2015)
Research Articles
Adaptive variation in physiological traits of beech provenances in Central Europe
vol. 11, pp. 24-31 (online: 09 January 2018)
Research Articles
Photosynthetic parameters of urban greening trees growing on paved land
vol. 12, pp. 403-410 (online: 13 August 2019)
Research Articles
Gas exchange characteristics of the hybrid Azadirachta indica × Melia azedarach
vol. 8, pp. 431-437 (online: 17 December 2014)
Research Articles
Drought tolerance in cork oak is associated with low leaf stomatal and hydraulic conductances
vol. 11, pp. 728-733 (online: 06 November 2018)
Research Articles
Gas exchange, biomass allocation and water-use efficiency in response to elevated CO2 and drought in andiroba (Carapa surinamensis, Meliaceae)
vol. 12, pp. 61-68 (online: 24 January 2019)
Research Articles
Links between phenology and ecophysiology in a European beech forest
vol. 8, pp. 438-447 (online: 15 December 2014)
iForest Database Search
Search By Author
Search By Keyword
Google Scholar Search
Citing Articles
Search By Author
Search By Keywords
PubMed Search
Search By Author
Search By Keyword