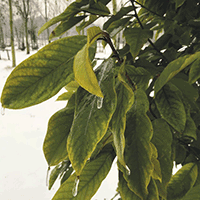
Relationships between leaf physiognomy and sensitivity of photosynthetic processes to freezing for subtropical evergreen woody plants
iForest - Biogeosciences and Forestry, Volume 12, Issue 6, Pages 551-557 (2019)
doi: https://doi.org/10.3832/ifor3196-012
Published: Dec 17, 2019 - Copyright © 2019 SISEF
Research Articles
Abstract
Subtropical and tropical species in high altitude suffer from low temperature more frequently than those from temperate regions. Chlorophyll fluorescence analysis can measure the primary photochemical processes of photosystem II (PSII) and help evaluate the sensitivity of evergreen woody plants to low temperature. Coupled with leaf physiognomy, it has allowed to examine the potential thermal regulation of evergreens in response to extreme coldness. The leaf physiognomy (length, width, thickness and ratio of length/width) and chlorophyll a fluorescence (Fv/Fm, maximum potential photochemical efficiency of PSII; NPQ, non-photochemical quenching of chlorophyll fluorescence; and Y(II), effective photochemical quantum yield of photosystem II) under natural freezing and recovery conditions of nine evergreen woody trees were measured to analyze their relationships. Results showed that the changes of Fv/Fm under freezing versus recovery had a positive relationship with leaf length and width, while a negative relationship with leaf thickness. Similar to leaf size, leaf shape also influenced the photoinhibition levels of evergreens by regulating the leaf boundary layer thickness. Leaves with an oval-like shape suffered less from freezing than leaves with a lanceolate-like shape. A relatively weaker relationship between NPQ and Y(II) was found at freezing than after recovery for species with larger and lanceolate-like leaves. Our findings are helpful to understand the adaptation strategy of evergreen woody species to extreme low temperature in subtropical areas and to provide guidance for the management of evergreen plants introduced in botanical gardens.
Keywords
Leaf Size, Leaf Shape, Chlorophyll a Fluorescence, Photoinhibition, Low Temperature Stress, Evergreens
Introduction
Evergreen woody plants living in the ecosystems of subtropical mountains, where water is not constraining, must retain their foliage amidst chronically large seasonal temperature variations. Subtropical evergreen broad-leaved trees are even able to withstand extreme cold when the winter temperature is as low as -15 °C ([12]). Therefore, the regional distribution of evergreen and deciduous species can overlap in terms of the altitudinal gradient ([35], [36]). Generally, evergreen species grow at low altitude whereas conifers grow at high altitude, and evergreen and deciduous species coexist at an intermediate altitude according to their phenological characteristics. This is also true for the replacement of life forms in different types of forests, i.e., the spatial vegetation shift patterns under various climatic regimes ([39], [31], [8], [12], [7]). More importantly, leaf phenology (evergreen vs. deciduous) provides one of the vital axes for vegetation classification ([42], [6]). As the altitude increases, the evergreens have to adapt to the low temperature environment, even frequently with freezing temperature. One of the survival strategies and adapting mechanisms for evergreen plants is morphometric plasticity, i.e., variation of leaf size. For example, on Mt. Emei of Sichuan in China, the leaf size for 88 evergreen broad-leaved trees was relatively smaller at high altitude than at low altitude ([36]).
The basic molecular, ecophysiological and bioclimatic foundations were previously studied for evergreen plant species under freezing temperatures ([8], [34], [12]). The response of the leaves for evergreens to extreme coldness is tightly related with leaf size ([12], [20]). This relationship is well expressed in the leaf energy balance theory ([29]), which can be summarized as follows: small leaves exposed to radiative cooling on clear nights should chill less than large leaves ([29], [18], [32], [20]). As the main determinant of boundary layer thickness, the effective width of leaves plays a role in convective heat exchange with the surrounding air. However, the physiological function of leaves responding to temperature may not only be related to leaf size, but also to another leaf dimension-leaf thickness ([27], [14], [1]). The thickness of leaves has proven to be closely associated with several climatic variables ([11], [26], [21]), and affects the foliar photosynthetic rates per unit area and accumulation of photosynthetic compounds ([25], [26], [16]). The influence of three dimensions of leaves on the efficiency of photosynthesis under natural freezing is still an open question. Theoretically, leaf shape, just like leaf size, is also linked to photosynthetic rates because of its role in regulating the thermal response of gas exchange for plant growth ([24]). The shape of leaves can affect heat transfer of leaf lamina, again through altering the leaf boundary layer ([37], [17]).
Temperature influences plant photosynthesis in an immediate way ([3]). Low temperature causes a decrease in the effective photochemical quantum yield of photosystem II [Y(II)] at moderate and high light intensity, which can be attributed to the direct effect of temperature on the kinetics of enzymes involved in carbon fixation ([15]). However, during prolonged exposure to low temperature, increased dark-sustained thermal energy dissipation and downregulation of PSII photochemistry occurs ([28], [19]), and mainly depends on the geographical origin of the species ([2]). Chlorophyll fluorescence analysis allows to monitor the sensitivity of photosynthetic processes of plants adapting to extreme low temperatures ([3]), in our case freezing temperature. Cold-induced photoinhibition develops relatively slowly in cold-tolerant species as compared to cold-sensitive species. One remarkable difference between these two kinds of species is the effect of low temperature on photoinhibition as shown by Fv/Fm measurements. Photochemical efficiency of overwintering evergreens under low temperature can be evaluated in terms of Y(II) and non-photochemical quenching of chlorophyll fluorescence (NPQ). These parameters provide a measure of the sensitivity of photochemical processes in PSII for leaves in a simple approximation ([3]), and of the level of non-radiative energy dissipation in the light-harvesting antenna of PSII ([4], [22]). Therefore, the adaptation of evergreen plants to freezing stress could be further assessed by detecting how much the relationship between NPQ and Y(II) differs.
Here we examine the impact of freezing on photochemical processes of PSII for leaves of nine evergreen angiosperms, which could be seriously related to leaf dimension. The effects of leaf size and leaf shape on the vulnerability to freezing could explain the altitudinal patterns of leaf characteristics in subtropical mountain areas where water is not a strong limiting factor. They also provide useful insights into the adaptation capacity of evergreen plants reintroduced into botanical gardens.
Material and methods
Study conditions and plant materials
The experiments were conducted at Poyang Lake Botanical Garden in Jiangxi Province, China (29° 40′ 12″ N, 116° 05′ 24″ E; elevation 25 m a.s.l.) during the transition from winter to spring in 2018. Specifically, two independent experiments were performed on January 30 after a snow fall of three consecutive days, and on April 9 when temperature increased (Fig. 1). The minimum temperatures of these two days were -5.3 °C and 9.2 °C, and the mean temperatures were -1.1 and 18.5 °C, respectively, representing freezing and recovery conditions (Fig. 1). Temperature data were obtained from a meteorological station in the botanical garden.
Fig. 1 - Daily temperature variations from January to April in 2017 and 2018. Maximal and minimal temperatures are represented as red and black lines, respectively. Solid lines and dashed lines represent temperatures in 2018 and 2017. Green arrows and filled circles indicate the days of two independent experiments.
Nine broad-leaved evergreen tree species grown in the botanical garden were studied: Photinia bodinieri, Ligustrum lucidum, Photinia × fraseri, Ormosia henryi, Phoebe zhennan, Ilex latifolia, Eriobotrya japonica, Michelia foveolate and Michelia odora. All trees were grown-up and cultivated for more than eight years. The heights of all species are more than 2 meters, but with variations. At least three mature sun leaves of each tree were chosen for measuring photosynthetic parameters. The minimum mean temperature of the coldest month (MTCOmin) for all evergreens (Tab. 1) are recorded in Fang et al. ([5]).
Tab. 1 - Measurements of leaf three dimensions, distribution and record of minimum MTCO for nine evergreen trees. Leaf three dimensions are shown in mean ± standard deviation. The distribution area and record of minimum mean temperature of the coldest month (MTCOmin) are taken from Fang et al. ([5]). (na): not available.
Species | Length (cm) |
Width (cm) |
Thickness (mm) |
Distribution Area | MTCOmin (°C) |
---|---|---|---|---|---|
Photinia bodinieri | 10.34 ± 0.34 | 3.72 ± 0.13 | 0.33 ± 0.03 | Mainly subtropical | -6.6 |
Ligustrum lucidum | 11.48 ± 1.25 | 5.18 ± 0.57 | 0.42 ± 0.03 | Temperate to tropical | -9.0 |
Photinia × fraseri | 9.68 ± 0.22 | 4.10 ± 0.32 | 0.34 ± 0.02 | Temperate to subtropical | na |
Ormosia henryi | 11.50 ± 0.94 | 4.64 ± 0.62 | 0.29 ± 0.02 | Subtropical to tropical | 1.1 |
Phoebe zhennan | 17.14 ± 1.30 | 5.86 ± 0.36 | 0.28 ± 0.01 | Mainly subtropical | -2.0 |
Ilex latifolia | 16.18 ± 1.37 | 5.18 ± 0.20 | 0.42 ± 0.03 | Mainly subtropical | 0.6 |
Eriobotrya japonica | 23.94 ± 1.97 | 7.36 ± 0.64 | 0.54 ± 0.06 | Subtropical to tropical | -2.1 |
Michelia foveolata | 17.74 ± 1.17 | 7.60 ± 0.89 | 0.48 ± 0.01 | Subtropical to tropical | 2.1 |
Michelia odora | 20.02 ± 0.41 | 8.34 ± 0.85 | 0.35 ± 0.04 | Subtropical to tropical | 3.8 |
Chlorophyll a fluorescence measurements
Chlorophyll a fluorescence of mature leaves was measured using LI-6800® portable photosynthesis system equipped with a multiphase flash fluorimeter (Li-COR Inc., Lincoln, NE, USA). Leaves were excised from the plants and immediately put in a dark bag, then dark-adapted for around 30 minutes before fluorescence measurements. Fo is the minimal fluorescence in the dark-adapted state. Fm is the maximum fluorescence in the dark-adapted state upon illumination with saturating light (10.000 μmol photons m-2 s-1). The maximum potential photochemical efficiency of PSII is calculated as Fv/Fm = (Fm-Fo) / Fm. The effective photochemical quantum yield of PSII was measured as Y(II) = (Fm′-Fs) / Fm′. Fm′ is the maximum light-adapted fluorescence and Fs is the light-adapted steady-state fluorescence. The non-photochemical quenching of chlorophyll fluorescence (NPQ) is a process in which excess absorbed light energy is dissipated as heat ([22]). It was calculated as (Fm-Fm′)/Fm′. For light response curve measurements, chlorophyll fluorescence parameters were evaluated at 90-120s intervals at increasing photosynthetic photon flux density (PPFD) of 0, 25, 50, 100, 150, 300, 500, 1000, 1500, 2000 μmol photons m-2 s-1 ([33]).
Measurements of leaf length, width and thickness
A ruler with a resolution of 1 mm was used to measure the length and width of leaves. Leaf length is the length from the leaf base (not including the petiole) to leaf tip. As leaf shapes ranged from oval and oblong to lanceolate or cuneate, leaf width is measured at the widest size of the lamina. Electronic caliper was used to measure leaf thickness, i.e., the thickness of the widest part of a leaf. Morphological measurements were made in the laboratory on each leaf after measuring chlorophyll a fluorescence (Tab. 1).
Statistical analysis
A linear regression model was used to detect the relationships between the change of Fv/Fm under two conditions (freezing versus recovery) and leaf morphometric traits (length, width and thickness). Theoretically, leaf length, leaf width and leaf thickness are affecting photosynthetic response to temperature stress through leaf temperature regulation. Therefore, a generalized linear regression model (GLM) was employed to deal with the cofounding factors ([38]). The advantage of this method is that it can show the relationship between the change of Fv/Fm and each leaf dimension after taking into account the others. Both models were run in R software (3.3.1 version). The relationships between NPQ and Y(II) under two temperature conditions were also plotted via R.
Results
Effect of leaf dimension on Fv/Fm differences
Linear regression showed that there was a significant positive relationship between leaf length and changes of Fv/Fm and the same relationship between leaf width and changes of Fv/Fm, but the relationship between leaf thickness and changes of Fv/Fm was negative and non-significant (Fig. 2a-c). Taking each dimension of a leaf into account, generalized linear regression revealed that both the relationship between leaf length and changes of Fv/Fm and the relationship between leaf width and changes of Fv/Fm were positive as well, but non-significant. However, the relationship between leaf thickness and changes of Fv/Fm was significantly negative (Fig. 2d-f). Combining two regression results, the relationships between Fv/Fm differences and individual leaf dimension were in agreement, although not all of the relationships were significant. Both linear regression and generalized linear regression indicated that large and thin leaves had a larger change of Fv/Fm, while small and thick leaves had a smaller change of Fv/Fm (Fig. 2).
Fig. 2 - Relationship of Fv/Fm changes with leaf length, width and thickness of 9 evergreen tree species. Linear model: (a), (b) and (c), and generalized linear model (GLM): (d), (e) and (f). Regression line in red indicates the relationship is significant (p<0.05). Regression line in black indicates the relationship is not significant (p>=0.05). The 95% confidence intervals are also shown as shadows in GLM.
Relationships between leaf size, shape and Fv/Fm differences
Species with narrower and shorter leaves appeared to have smaller Fv/Fm changes under freezing and recovery conditions, while species with wider and longer leaves have larger Fv/Fm changes (Fig. 3a). There was a non-significant positive relationship between Fv/Fm differences and the ratio of length to width for nine evergreen tree species, i.e., species with lower length/ width ratio had smaller changes of Fv/Fm and species with higher length/width ratio had larger changes of Fv/Fm (Fig. 3b).
Fig. 3 - Changes of Fv/Fm in leaf dimension space of width vs. length and its relationship to the ratio of length/width for 9 evergreen tree species. The size of yellow solid circle is proportional to the changes of Fv/Fm (left).
Relationship between NPQ and Y(II) under freezing and recovery conditions
There was a significant negative relationship between NPQ and Y(II) both under freezing and recovery conditions for all species (Fig. 4). The relationship became weaker during freezing than that during recovery for most of the species, except for Photinia bodinieri, Photinia × fraseri and Ilex latifolia. The absolute change of the slope of the relationship was more than 0.5 for Ormosia henryi, Eriobotrya japonica, Phoebe zhennan and Michelia foveolata, while it was less than 0.5 for the remaining species.
Fig. 4 - Relationship between NPQ and Y(II) responding to PPFD. Black and red solid circles represent data of freezing and recovery conditions, respectively. The size of circles increases with increasing PPFD (0, 25, 50, 100, 150, 300, 500, 1000, 1500, 2000 μmol photons m-2 s-1).
Discussion
We have shown that the photoinhibition of photosynthesis for nine evergreen tree species is correlated to three dimensions of their leaves. The univariate method isolated the relationship between changes of Fv/Fm and single dimension of leaves in a simple way. On one hand, it is in agreement with the conclusions of the leaf energy balance theory ([29]), which states that the leaf width is the main determinant of boundary layer thickness when responding to low temperature ([20]). On the other hand, it illustrated that leaf length, as a dimension of leaf size, is also playing a vital role in regulating the thermal dynamics. The multivariate method (GLM), taking the three dimensions of a leaf into account together, further confirmed the relationships between the photoinhibition of evergreens and the basic leaf morphometry. Although the relationships are not always significant with two statistical frameworks, they both indicate the same relations, i.e., longer, wider and thinner leaves have a larger change of Fv/Fm, while shorter, narrower and thicker leaves have a smaller change of Fv/Fm. The results of larger (longer and wider) leaves being more vulnerable to low temperature stress than small (smaller and narrower) leaves is consistent with the conclusions drawn by Lusk et al. ([20]). However, the experimental materials they employed were seedlings of 15 New Zealand evergreens. The duration of freezing for evergreen leaves varied with leaf thickness, which alters leaf photosynthetic capacity through the regulation of the nadir temperature of leaves ([25], [1]). In the current study, lasting freezing temperatures (snowing for three successive days) cause sustained photoinhibition to a larger extent for thinner leaves and less for thicker leaves. Furthermore, Ball et al. ([1]) suggested that the leaf tip and margins are more vulnerable to freezing damage because they are thinner than the central part of a leaf. This also mirrors the similar relationship between leaf thickness and the tolerance of leaves to extreme coldness, which is reflected by the vulnerability to photoinhibition. Of course, the degree of the ultimate freezing damage for evergreen leaves is not only dependent on the leaf three dimensions, but also on other factors, such as water content of leaves ([1]).
According to the experiments conducted by using artificial metal leaves, the theory proves that leaf size and shape strongly influence leaf thermal dynamics through the boundary layer thickness of a leaf ([10], [9]). This theory does hold up under natural conditions ([17], [23]). Our results suggest that evergreen plants bearing small leaves with an oval-like shape (low length/width ratio) most likely function better in tolerating the freezing temperature than plants with large leaves with lanceolate or cuneate-like shapes (high length/width ratio). Though the relationship between the changes of Fv/Fm and the length/width ratio is not significant, the positive relationship more or less indicates that leaf shape indeed matters for regulating photoinhibition. As the ratio of length/ width increases from 2.2 to 3.2, the change of Fv/Fm for evergreen leaves responding to freezing temperature increases from about 0.05 to 0.3. This indicates that a unit change of the length/width ratio could lead to significant variation of photoinhibition due to different leaf geometry in evergreens.
Species from diverse origins have various adaptability to stress environments ([2]). Thus, the range of distribution of both deciduous and evergreen species could cover both favorable and unfavorable regions ([8], [12]). From the corresponding relationship between distribution and minimum MTCO for evergreen species in the current study, it seems likely that those originated from tropical or subtropical origin have high minimum MTCO, whereas those from temperate to subtropical areas have low minimum MTCO (Tab. 1). For example, Michelia odora only can tolerate the extreme MTCOmin, equivalent to 3.8 °C. In contrast, Ligustrum lucidum can grow in temperate to tropical areas with an MTCOmin of -9 °C, possibly because of a very different leaf texture ([13]). In January of 2018, it continuously snowed for three days and definitely caused freezing damage to most of our investigated evergreens when the lowest temperature was -5.3 °C.
For the NPQ and Y(II) relationship, the slope changes of Ligustrum lucidum and Michelia odora were 0.14 and 0.47, separately, under freezing vs. recovery conditions. This suggests that the negative relationship between NPQ and Y(II) for species with tropical characteristics will change more than that for species with temperate characteristics. Coincidently, like Ligustrum lucidum, also Photinia bodinieri and Photinia × fraseri have oval-like leaf shapes (low length/width ratio) with a relatively small leaf size, and display a higher photochemical activity as well as an obvious photoprotective mechanism in terms of NPQ and YII relationship. In contrast to the above evergreen species, Eriobotrya japonica and Michelia foveolata have a similar leaf shape as Michelia odora, i.e., lanceolate-like with a relatively large leaf size and display a lower photochemical activity and non-obvious photoprotective mechanism. Ormosia henryi, Phoebe zhennan and Ilex latifolia have a seemingly transitional leaf shape type between oval-like and lanceolate-like. These observations demonstrate that leaf physiognomy components (size and shape) play an important role in regulating the leaf temperature when experiencing freezing stress. The mechanism of this thermal regulation for leaves is explained by the well-known energy balance theory ([29]), which is confirmed by many studies ([30], [17], [40], [20]). However, the slope change of the NPQ and Y(II) relationship for Ormosia henryi is 1.19, lower than that of Eriobotrya japonica, which has a figure of 3.02. This might be related to the leaf characteristics of compound for Ormosia henryi, because the factor of leaf size can be compromised through either a deeply dissected leaf or an entire, narrow leaf ([17]).
Visually, the leaves of nine evergreen trees showed more or less freezing damage as reflected by the development of necrotic areas, a similar symptom as observed for Eucalyptus pauciflora through freezing ([1]). Michelia odora is one of the typical species suffering from freeze stress (see Fig. S1 in Supplementary material for necrotic areas of the leaves). Firstly, this species has large thin leaves, which is not favourable for regulating leaf temperature under freezing temperature. The reason is that a large leaf is less closely coupled to ambient air temperature than a small leaf, as their thicker boundary layer impedes convective heat exchange ([20]). Secondly, the cultivated site of this species is beyond the northern boundary of its natural distribution ([5]). This species originating from subtropical to tropical areas, can only potentially tolerate the minimum MTCO with 3.8 °C. Snowing accompanied with temperatures as low as -5.3 °C represents a freezing disaster for it. Thirdly, from the photochemical perspective, the NPQ and Y(II) relationship for Michelia odora under freezing becomes less strong than that under recovery conditions. All of these observations indicate that Michelia odora is an evergreen plant sensitive to freezing stress.
Conclusions
The positive relationships between leaf length or width and changes of Fv/Fm and the negative relationship between leaf thickness and changes of Fv/Fm under freezing and recovery demonstrate that three dimensions of a leaf greatly influence sensitivity of photosynthetic processes, i.e., primary photochemical processes of PSII for evergreen plants. Small and thick leaves with an oval-like shape are less sensitive than large and thin leaves with a lanceolate-like shape when enduring freezing. Our findings advance the understanding of the role of leaf size and leaf shape for evergreens facing low temperature stress. The resistance of evergreen trees responding to low temperature can be monitored by the variation of the relationship between NPQ and Y(II) from freezing to recovery. We propose that evergreen broad-leaved trees originating from tropical or subtropical areas have higher minimum MTCO and are less resistant to low temperature. Finally, because leaf physiognomy displays a global pattern along with the temperature gradient ([41], [20]), this potentially implies that various measures should be taken for protecting introduced evergreen species with different leaf sizes and shapes in botanical gardens.
Acknowledgements
We would like to thank two anonymous reviewers for their helpful comments and advice. We also thank Prof. Jean-David Rochaix at the University of Geneva for his assistance with English language and grammatical editing of the manuscript. CD and ZZ designed the experiments, carried out the field measurements, performed the statistical analysis and drafted the manuscript. ZS, PY and ZL helped to carry out the measurements in the laboratory. This work was financially supported by the National Natural Science Foundation of China (41861003) and Special Project of “Lushan Plants” (2019ZWZX03).
References
Gscholar
CrossRef | Gscholar
Gscholar
CrossRef | Gscholar
Gscholar
Authors’ Info
Authors’ Affiliation
Zhiyong Zhang
Saixia Zhou
Yansong Peng
Li Zhang
Lushan Botanical Garden, Chinese Academy of Sciences, 332900, Jiangxi (China)
Corresponding author
Paper Info
Citation
Cheng D, Zhang Z, Zhou S, Peng Y, Zhang L (2019). Relationships between leaf physiognomy and sensitivity of photosynthetic processes to freezing for subtropical evergreen woody plants. iForest 12: 551-557. - doi: 10.3832/ifor3196-012
Academic Editor
Claudia Cocozza
Paper history
Received: Jul 19, 2019
Accepted: Oct 14, 2019
First online: Dec 17, 2019
Publication Date: Dec 31, 2019
Publication Time: 2.13 months
Copyright Information
© SISEF - The Italian Society of Silviculture and Forest Ecology 2019
Open Access
This article is distributed under the terms of the Creative Commons Attribution-Non Commercial 4.0 International (https://creativecommons.org/licenses/by-nc/4.0/), which permits unrestricted use, distribution, and reproduction in any medium, provided you give appropriate credit to the original author(s) and the source, provide a link to the Creative Commons license, and indicate if changes were made.
Web Metrics
Breakdown by View Type
Article Usage
Total Article Views: 40675
(from publication date up to now)
Breakdown by View Type
HTML Page Views: 35634
Abstract Page Views: 2732
PDF Downloads: 1713
Citation/Reference Downloads: 10
XML Downloads: 586
Web Metrics
Days since publication: 1977
Overall contacts: 40675
Avg. contacts per week: 144.02
Article Citations
Article citations are based on data periodically collected from the Clarivate Web of Science web site
(last update: Mar 2025)
Total number of cites (since 2019): 1
Average cites per year: 0.14
Publication Metrics
by Dimensions ©
Articles citing this article
List of the papers citing this article based on CrossRef Cited-by.
Related Contents
iForest Similar Articles
Research Articles
Calibration of a multi-species model for chlorophyll estimation in seedlings of Neotropical tree species using hand-held leaf absorbance meters and spectral reflectance
vol. 9, pp. 829-834 (online: 17 May 2016)
Research Articles
Wintertime photosynthesis and spring recovery of Ilex aquifolium L.
vol. 12, pp. 389-396 (online: 31 July 2019)
Research Articles
Magnolia grandiflora L. shows better responses to drought than Magnolia × soulangeana in urban environment
vol. 13, pp. 575-583 (online: 07 December 2020)
Research Articles
Links between phenology and ecophysiology in a European beech forest
vol. 8, pp. 438-447 (online: 15 December 2014)
Research Articles
Successional leaf traits of monsoon evergreen broad-leaved forest, Southwest China
vol. 10, pp. 391-396 (online: 16 March 2017)
Research Articles
Adaptive variation in physiological traits of beech provenances in Central Europe
vol. 11, pp. 24-31 (online: 09 January 2018)
Research Articles
Salinity strongly drives the survival, growth, leaf demography, and nutrient partitioning in seedlings of Xylocarpus granatum J. König
vol. 10, pp. 851-856 (online: 26 October 2017)
Research Articles
Gas exchange characteristics of the hybrid Azadirachta indica × Melia azedarach
vol. 8, pp. 431-437 (online: 17 December 2014)
Research Articles
A comparative study of growth and leaf trait variation in twenty Cornus wilsoniana W. families in southeastern China
vol. 10, pp. 759-765 (online: 02 September 2017)
Research Articles
Leaf transpiration of drought tolerant plant can be captured by hyperspectral reflectance using PLSR analysis
vol. 9, pp. 30-37 (online: 05 October 2015)
iForest Database Search
Search By Author
Search By Keyword
Google Scholar Search
Citing Articles
Search By Author
Search By Keywords
PubMed Search
Search By Author
Search By Keyword