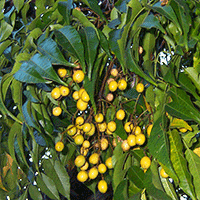
Gas exchange characteristics of the hybrid Azadirachta indica × Melia azedarach
iForest - Biogeosciences and Forestry, Volume 8, Issue 4, Pages 431-437 (2014)
doi: https://doi.org/10.3832/ifor1127-007
Published: Dec 17, 2014 - Copyright © 2014 SISEF
Research Articles
Abstract
The hybrid Azadirachta indica × Melia azedarach is a new plant variety that was obtained through somatic hybridization. The plant could grow normally in regions with an average annual temperature of 15 °C, and it had a higher concentration of active insecticidal substance in its seeds. Therefore, the hybrid will likely become a valuable new tree species in subtropical and warm temperate regions. However, the photosynthetic physiological characteristics of A. indica × M. azedarach remain unknown. The photosynthetic gas exchange of the hybrid at three different ages (one year old (AM1), three years old (AM3), and five years old (AM5)) were measured. The specific leaf mass per area (LMA), leaf N, leaf P, and leaf N/P of each tree sample were measured, and the photosynthetic N and P use efficiencies (PNUE and PPUE, respectively) were also calculated. The maximum leaf net photosynthetic rate Pa (based on area), Pm (based on mass), light saturation point (LSP), light compensation point (LCP), stomatal conductance (gs), and transpiration rate (Tr) of A. indica × M. azedarach decreased with increasing tree age, whereas the instantaneous water use efficiency (WUE) increased with age. The photosynthetic capacity showed no significant differences between AM3 and AM5 but was significantly higher in AM3 and AM5 when compared with AM1.The Pa, Pm, apparent quantum yield (AQY), LSP, gs, and Tr of A. indica × M. azedarach were significantly lower than that of the parental M. azedarach, whereas the dark respiration rate (Rd) and WUE were significantly higher than that of M. azedarach. A reduction in the maximal photosynthetic rate of A. indica × M. azedarach that was observed with increased age was primarily related to the increased LMA and the decline in leaf nitrogen (N) and leaf phosphorus (P) concentrations. Additionally, the decline in stomatal conductance (gs) was also an important factor leading to age-dependent reductions in the photosynthetic rate. These findings suggest that the tree’s age has a significant impact on A. indica × M. azedarach gas exchange during juvenile stages, and the photosynthetic capacity of the hybrid was significantly lower than that of the parental M. azedarach.
Keywords
Photosynthesis, Ontogeny, Stomatal Conductance, Leaf Nitrogen, Leaf Phosphorus, Leaf Mass Per Area
Introduction
Azadirachta indica is a valuable multipurpose tree species producing a variety of active insecticidal substances, such as azadirachtin, which can be found at high concentrations in the seeds. A. indica is internationally recognized as having the greatest potential for the production of biological pesticide ([43], [26]). Therefore, such species has been introduced into many tropical and subtropical countries and regions. However, A. indica exhibits poor growth in regions with an average annual temperature below 20 °C and a minimum temperature below 0 °C ([11], [26]).
Both Melia azedarach and A. indica belong to the Meliaceae family, and the M. azedarach is widely distributed in China within broad latitudinal (18-39°) and altitudinal (600-800 m a.s.l.) ranges ([5]). The active insecticidal substances of M. azedarach are primarily located in its bark, while seeds and leaf contents are very low, thus limiting its further exploitation. The Nanjing Jiukang Biological Development Co., Ltd. successfully cultivated a hybrid of A. indica (from Myanmar) and M. azedarach (from Nanjing) for the first time through somatic hybridization technology. This A. indica × M. azedarach hybrid inherited the excellent features of its parental species, growing normally in Nanjing, which has an average annual temperature of 15 °C, but also producing an active insecticidal substance in its seeds at a markedly higher concentration than that of the local M. azedarach. At present, the hybrid has been planted in a small area in Nanjing, China and has produced viable seeds.
Photosynthesis plays a vital role in tree growth, production and regeneration. Previous studies have indicated that hybrids and their parents exhibit differences in their photosynthetic characteristics ([3], [41]). The difference in photosynthesis between hybrids and their parents primarily depended on differences in parental genotype and habitats ([41]). A. indica × M. azedarach is a new variety, and so far no assessment have been carried out of the photosynthetic physiological characteristics of the hybrid or its differences in terms of gas exchange parameters relative to its parents.
Previous studies showed strong differences in photosynthetic characteristics of forest species at different developmental stages. The photosynthetic rate at the leaf level decreases or increases with increasing tree age or size ([1], [16], [15], [23]). However, most studies only compared the photosynthetic gas exchange between saplings and adults, while few studies focused on the physiology of trees at younger stages ([37], [9], [31]). Photosynthetic characteristics during the juvenile stages are strongly related to early growth and regeneration. Several studies performed at the juvenile stage primarily focused on photosynthetic capacity changes related to age in conifers ([31]). Few studies on broadleaf species showed that the photosynthetic capacity on a mass or leaf area basis increased from sapling to intermediate sized trees ([36]). Considerable research on age-related changes in leaf gas exchange has focused on the “hydraulic limitation hypothesis”, i.e., the reduction of the leaf specific hydraulic conductance as trees grow in height due to an increased path length in roots, stems and branches ([32], [14], [22]). However, recent studies suggested that leaf functional traits (anatomy, morphology, or chemistry) might play important roles in age-related changes in gas exchange ([36], [34]).
The primary purposes of the present study were: (1) to compare the variation in gas exchange parameters of A. indica × M. azedarach at young stages (seedlings, older seedlings, and saplings); (2) to compare differences in gas exchange parameters between the hybrid A. indica × M. azedarach and its parents; and (3) to evaluate the relation between the gas exchange parameters of A. indica × M. azedarach and its leaf mass per area (LMA) and leaf nitrogen (N) and/or phosphorous (P) concentrations with increasing tree age.
Materials and Methods
Plant materials
The A. indica × M. azedarach hybrid and one of its parents (M. azedarach) were studied. The other parent species A. indica was not included in the study because it cannot grow in the study area. The A. indica × M. azedarach hybrid was created by somatic hybridization in 2002. In 2005, the Nanjing Jiukang Biological Development Co., Ltd. began to plant A. indica × M. azedarach hybrid trees in the Qiaoli Village of the Gaochun County. At present, the total planting area covers more than 300 ha in Nanjing. In June 2011, two or three A. indica × M. azedarach stands with one-year-old (AM1), three-year-old (AM3), and five-year-old (AM5) specimens and two stands with three-year-old M. azedarach (MA3) were selected to measure their photosynthetic gas exchange characteristics.
Study site
The study was conducted in the Qiaoli Village, Gaochun County, Jiangsu Province, China (119° 06′ 02″ E, 31° 24′ 38″ N; mean elevation: 26 m a.s.l.). The annual average precipitation at the study site is 1157 mm, annual average temperature 15.4 °C, and the monthly average temperatures in January and July are 4.4 °C and 29 °C, respectively. The annual sunshine total is 1937 h, with 260 frost-free days throughout the year. The study site belongs to the northern subtropical monsoon climate zone. The soil is loamy with a pH ranging from 5.5 to 6.0. The site conditions of AM1, AM3, and AM5 were similar in that they were all planted in farmland. The slopes of the study area varied from 5% to 10%. The basic characteristics of the stands used in the study are shown in Tab. 1. The understory vegetation coverage in these stands was primarily Oplismenus undulatifolius (approximately 80-90%).
Tab. 1 - Average tree height, diameter at breast height (DBH), crown height, and tree density of AM1 (one-year-old A. indica × M. azedarach), AM3 (three-year-old A. indica × M. azedarach), AM5 (five-year-old A. indica × M. azedarach), and MA3 (3-year-old M. azedarach).
Stand | Tree height (m) |
DBH (cm) |
Crown height (m) |
Tree density (tree ha-1) |
---|---|---|---|---|
AM1 | 1.8 ± 0.2 | 1.2 ± 0.1 | 1.4 ± 0.1 | 1667 ± 72 |
AM3 | 3.6 ± 0.3 | 5.3 ± 0.4 | 2.3 ± 0.2 | 1458 ± 58 |
AM5 | 5.3 ± 0.4 | 8.3 ± 0.5 | 3.6 ± 0.2 | 1046 ± 45 |
MA3 | 3.8 ± 0.4 | 5.7 ± 0.5 | 2.5 ± 0.3 | 552 ± 29 |
Photosynthetic gas exchange measurements
The photosynthetic gas exchange of the leaves was measured with a LI-6400 portable photosynthesis system (Li-Cor, Inc., Lincoln, NE, USA). According to the mean DBH, tree height, and crown height, four typical sample trees of M. azedarach and A. indica × M. azedarach from three differently aged stands were selected. Four fully expanded and exposed leaves (of similar ages) from the middle layer of the tree canopy in each sample tree were selected for photosynthetic gas exchange measurements. The conditions in the leaf chamber were controlled automatically by the instrument with a CO2 concentration of 370 ± 10 μmol mol -1, and the leaf temperature was maintained at 30 ± 1 °C. The air flow rate was set to 500 mL min-1. The photosynthetic light response was obtained by measuring 11 different photosynthetic active radiation (PAR) values (2000, 1500, 1000, 800, 500, 200, 100, 80, 50, 20, and 0 μmol m-2 s-1). Light was provided by a Li-6400 LED red/blue light source. Such measurements were performed from 9:00 to 11:30 a.m. in mid-June 2011. The maximum net photosynthetic rate (Pn), the apparent quantum yield (AQY), and the dark respiration (Rd) were calculated using the method described by Bassman & Zwier ([3]), and the stomatal conductance (gs), transpiration rate (Tr), and instantaneous water use efficiency (WUE, Pn/Tr) were estimated at the maximal photosynthetic rate (eqn. 1):
where Pn is the net photosynthetic rate (μmol m-2 s-1), Pmax is the maximum net photosynthetic rate (μmol m-2 s-1), AQY is the apparent quantum yield (μmol m-2 s-1), PAR is photosynthetically active radiation (μmol m-2 s-1), and Rd is dark respiration (μmol m-2 s-1). The light compensation point (LCP) was obtained by performing a linear regression with data taken at PPFDs of 0, 20 and 50 μmol m-2 s-1 ([13]). The light saturation point (LSP) was estimated according to the trends in the Pn-PAR curves ([42]).
Measurements of the LMA and leaf N and P concentrations
After measuring the photosynthetic gas exchange, all the leaves were collected and scanned to measure the leaf area using a WinFOLIA Analysis System (Regent Instruments Inc., Canada). Sampled leaves were then dried at 65 °C until a constant mass was reached, in order to calculate the specific leaf mass per area (LMA, g m-2). The dried leaves were then ground and passed through a 2-mm sieve. The total nitrogen (N) concentration was determined by Kjeldahl method. The phosphorus (P) concentration was determined by the colorimetric method described by Murphy & Riley ([21]), with the absorbance wave length set at 880 nm. These measurements were used to determine the leaf N/P ratio for A. indica × M. azedarach and M. azedarach. The leaf N and P concentrations are presented on a dry-mass basis. The photosynthetic use efficiencies for N (PNUE) and P (PPUE) were calculated as the ratio of the mass-based maximum photosynthetic capacity (Pm) to the leaf N and P concentrations, respectively.
Statistical analysis
One-way analysis of variance (ANOVA) followed by post-hoc Duncan’s test (α = 0.05) was carried out to test for differences in photosynthetic gas exchange parameters, LMA, leaf N, leaf P, leaf N/P, PNUE, and PPUE between the A. indica × M. azedarach specimens of different ages. Pearson’s correlation between the photosynthetic parameters and the LMA, leaf N, leaf P, and leaf N/P was also calculated. A linear regression analysis was performed to test the contribution of several photosynthetic gas exchange parameters and leaf trait parameters to maximal net photosynthetic rate. All statistical analyses were performed using the software package SPSS® 13.0 (SPSS Inc., Chicago, Illinois, USA).
Results
Photosynthetic gas exchange
The maximal net photosynthetic rate Pa, Pm, LSP, and gs of A. indica × M. azedarach decreased with increasing tree age (Tab. 2). These parameters (except LSP) were significantly higher for AM1 than for AM3 and AM5 (P<0.05). No significant difference were found between AM3 and AM5 (P>0.05). The AQY of AM1 was significantly higher than that of AM3 (P<0.05), whereas that of AM5 was between the values obtained for AM1 and AM3. The Rd of AM1 and AM3 were significantly higher than that of AM5 (P<0.05). The WUEs of AM3 and AM5 were significantly higher than that of AM1 (P<0.05).
Tab. 2 - Photosynthetic gas exchange parameters Pa (based on area), Pm (based on mass), AQY, LSP, LCP, Rd, gs, Tr, and WUE of AM1 (one-year-old A. indica × M. azedarach), AM3 (three-year-old A. indica × M. azedarach), AM5 (five-year-old A. indica × M. azedarach), and MA3 (three-year-old M. azedarach). Different letters between values along the same row indicate significant differences after the post-hoc Duncan’s test (P ≤ 0.05; n=13-16; means ± SE).
Parameters | AM1 | AM3 | AM5 | MA3 |
---|---|---|---|---|
Pa (μmol m-2 s-1) |
14.94 ± 0.37 b | 12.71 ± 0.25 a | 11.89 ± 0.31 a | 16.82 ± 0.46 c |
Pm (μmol g-1 s-1) |
312.08 ± 7.77 b | 200.93 ± 3.95 a | 179.32 ± 4.68 a | 376.29 ± 9.13 c |
AQY (μmol mol-1) |
0.055 ± 0.002 b | 0.051 ± 0.001 a | 0.053 ± 0.002 a b | 0.056 ± 0.001 b |
Rd (μmol m-2 s-1) |
1.249 ± 0.025 b | 1.287 ± 0.031 b | 1.146 ± 0.018 a | 1.119 ± 0.021 a |
LSP (μmol m-2 s-1) |
1284.8 ± 21.6 b | 1195.1 ± 15.8 ab | 1049.9 ± 17.2 a | 1425.5 ± 25.3 c |
LCP (μmol m-2 s-1) |
28.54 ± 1.62 a | 27.94 ± 1.87 a | 27.31 ± 1.49 a | 33.87 ± 2.05 a |
gs (mol m-2 s-1) |
0.065 ± 0.005 b | 0.044 ± 0.003 a | 0.040 ± 0.003 a | 0.077 ± 0.006 c |
Tr (mmol m-2 s-1) |
2.496 ± 0.213 b | 1.538 ± 0.156 a | 1.435 ± 0.138 a | 2.417 ± 0.251 b |
WUE (μmol mmol -1) |
5.986 ± 0.305 a | 8.264 ± 0.231 c | 8.286 ± 0.294 c | 6.959 ± 0.263 b |
In comparison with MA3, the maximal net photosynthetic rate (Pa) of AM3 was only 75.6% of that observed for MA3. In addition, the AQY, LSP, gs and Tr of AM3 were significantly lower (P<0.05), and the Rd and WUE were significantly higher than those of MA3 (P<0.05). No significant differences in the LCP were detected between AM3 and MA3 (P>0.05).
Changes in the LMA, leaf N, and leaf P
As the age of A. indica × M. azedarach increased, the leaf N and leaf P concentrations decreased gradually, whereas the leaf N/P and LMA gradually increased (Fig. 1A-F). The leaf N, leaf P, PNUE, and PPUE of AM1 were significantly higher than those of AM3 and AM5 (with the exception of the PPUE in AM5 - P<0.05), whereas the leaf N/P and LMA of AM1 were significantly lower than those of AM3 and AM5 (P<0.05), with the exception of the leaf N/P of AM3. The Leaf P, LMA, PNUE, and PPUE exhibited no significant difference between AM3 and AM5 (P>0.05). The leaf N of AM3 was significantly higher than that of AM5 (P<0.05), whereas the leaf N/P of AM3 was significantly lower than that of AM5 (Fig. 1A and Fig. 1E - P<0.05). The leaf N, leaf P, PNUE, and PPUE of AM3 were significantly lower than those of MA3 (P<0.05), and the LMA of AM3 was significantly higher than that of MA3 (P<0.05). No significant difference in the leaf N/P were observed between AM3 and MA3 (P>0.05).
Fig. 1 - Comparison of leaf N (A), leaf P (B), PNUE (C), PPUE (D), leaf N/P (E), and LMA (F) among AM1 (one-year-old A. indica × M. azedarach), AM3 (three-year-old A. indica × M. azedarach), AM5 (five-year-old A. indica × M. azedarach), and MA3 (three-year-old M. azedarach). Different letters indicate significant differences across age classes (AM1-AM5) and MA3 after the Duncan’s test (P ≤ 0.05). Error bars represent standard errors (n=4).
Correlation analysis
Correlations between the photosynthetic gas exchange parameters and leaf traits with age for A. indica × M. azedarach are shown in Tab. 3. The Pa, LSP, and LCP were significantly and positively correlated with the leaf N concentration. The Pa, Pm, LCP, gs, and Tr were significantly and positively correlated with the leaf P concentration. Significantly negative correlations were observed between the LMA and Pa, Pm, gs, and Tr. The WUE was significantly and positively correlated with the LMA. The LSP and LCP were significantly and positively correlated with the PNUE, and the LSP was negatively correlated with the PPUE. No significant correlation was observed between the other gas exchange parameters and PPUE or PNUE.
Tab. 3 - Correlations between photosynthetic gas exchange parameters and leaf traits with tree age. (*): P ≤0.05; (**): P ≤0.01.
- | P a | P m | AQY | R d | LSP | LCP | g s | T r | WUE | PNUE | PPUE | LN | LP | N/P | LMA |
---|---|---|---|---|---|---|---|---|---|---|---|---|---|---|---|
P a | 1.000 | 0.963** | 0.426 | 0.287 | 0.858** | 0.433 | 0.948** | 0.972** | -0.964** | 0.820** | 0.315 | 0.900** | 0.987** | -0.965** | -0.975** |
P m | - | 1.000 | 0.574 | 0.411 | 0.933** | 0.591* | 0.974** | 0.962** | -0.940** | 0.726** | 0.203 | 0.945** | 0.974** | -0.908** | -0.976** |
AQY | - | - | 1.000 | 0.100 | 0.498 | 0.827** | 0.644* | 0.557 | -0.527 | 0.057 | -0.286 | 0.469 | 0.411 | -0.287 | -0.489 |
R d | - | - | - | 1.000 | 0.584* | 0.308 | 0.329 | 0.281 | -0.143 | 0.162 | 0.029 | 0.574 | 0.402 | -0.228 | -0.274 |
LSP | - | - | - | - | 1.000 | 0.631* | 0.884** | 0.851** | -0.805** | 0.533 | -0.004 | 0.970** | 0.906** | -0.784** | -0.879** |
LCP | - | - | - | - | - | 1.000 | 0.685* | 0.577* | -0.542 | -0.076 | -0.562 | 0.612* | 0.476 | -0.298 | -0.498 |
g s | - | - | - | - | - | - | 1.000 | 0.984** | -0.966** | 0.647* | 0.091 | 0.919** | 0.951** | -0.875** | -0.954** |
T r | - | - | - | - | - | - | - | 1.000 | -0.983** | 0.720** | 0.188 | 0.892** | 0.963** | -0.912** | -0.956** |
WUE | - | - | - | - | - | - | - | - | 1.000 | -0.739** | -0.204 | -0.849** | -0.943** | 0.922** | 0.967** |
PNUE | - | - | - | - | - | - | - | - | - | 1.000 | 0.775** | 0.605* | 0.792** | -0.881** | -0.792** |
PPUE | - | - | - | - | - | - | - | - | - | - | 1.000 | 0.094 | 0.269 | -0.386 | -0.280 |
LN | - | - | - | - | - | - | - | - | - | - | - | 1.000 | 0.945** | -0.815** | -0.915** |
LP | - | - | - | - | - | - | - | - | - | - | - | - | 1.000 | -0.956** | -0.976** |
N/P | - | - | - | - | - | - | - | - | - | - | - | - | - | 1.000 | 0.942** |
LMA | - | - | - | - | - | - | - | - | - | - | - | - | - | - | 1.000 |
Regression analysis
Based on the result of the correlation analysis, nine variables showing significant correlations were further analyzed as possible predictors of Pa using a simple linear regression analysis. Linear regression parameters for these relations are shown in Tab. 4. All the models between Pa and LSP, gs, Tr, WUE, PNUE, TN, TP, N/P or LMA were significant (P<0.001). The standardized partial regression coefficient was highest for LMA, followed by gs and TP, the Tr and TN concentration also had important effects on Pa, and other variables had a relatively small impact on Pa.
Tab. 4 - Linear regression analysis between the maximal net photosynthetic rate (based on area) and LSP, gs, Tr, WUE, PNUE, TN, TP, N/P or LMA with tree age. (Coefficient): partial regression coefficient; (Std coeff.): standardized partial regression coefficient.
Variable | Coefficient | SE | Std coeff. |
Whole model | |||
---|---|---|---|---|---|---|---|
P | F | r2 | P | ||||
LSP | 0.012 | 0.001 | 0.933 | <0.001 | 66.789 | 0.857 | <0.001 |
Constant | -1.067 | 1.751 | 0.000 | ||||
g s | 107.890 | 7.942 | 0.974 | <0.001 | 184.552 | 0.943 | <0.001 |
Constant | 7.828 | 0.407 | 0.000 | ||||
T r | 2.538 | 0.227 | 0.962 | <0.001 | 124.526 | 0.918 | <0.001 |
Constant | 8.550 | 0.432 | 0.000 | ||||
WUE | -1.131 | 0.130 | -0.940 | <0.001 | 76.134 | 0.872 | <0.001 |
Constant | 21.739 | 0.993 | 0.000 | ||||
PNUE | 1.156 | 0.145 | 0.930 | <0.001 | 63.828 | 0.851 | <0.001 |
Constant | 5.408 | 0.986 | 0.000 | ||||
TN | 0.366 | 0.035 | 0.958 | <0.001 | 112.219 | 0.910 | <0.001 |
Constant | 0.729 | 1.182 | 0.000 | ||||
TP | 2.595 | 0.191 | 0.974 | <0.001 | 184.238 | 0.943 | <0.001 |
Constant | 6.800 | 0.481 | 0.000 | ||||
N/P | -0.846 | 0.123 | -0.908 | <0.001 | 47.202 | 0.808 | <0.001 |
Constant | 25.168 | 1.755 | 0.000 | ||||
LMA | -0.159 | 0.011 | -0.976 | <0.001 | 197.31 | 0.947 | <0.001 |
Constant | 22.557 | 0.675 | 0.000 |
Discussion
The photosynthetic gas exchange is affected by ontogeny. The Pa, Pm, LSP, LCP, gs, and Tr of A. indica × M. azedarach decreased with increasing tree age, whereas the instantaneous WUE increased. In general, the photosynthetic gas exchange parameters of AM3 and AM5 were significantly lower than the parameters of AM1 (P<0.05), and no significant differences in these parameters were observed between AM3 and AM5 (P>0.05). Thorton et al. ([37]) reported that the Pm of high-elevation red spruce (Picea rubens) was reduced by 32% from seedling to sapling. Greenwood et al. ([9]) also showed that the Pm of the 12-year age class of red spruce trees exhibited a significant decrease compared with the three-year-old trees, and the Pa and gs were similar between the three-year age and 12-year age classes. Reinhardt et al. ([31]) found that the photosynthetic gas exchange parameters at the leaf level in Abies fraseri exhibited no significant difference between seedlings (<3 cm in height), older seedlings (>three years of age and ~0.15-1 m in height), and saplings (>10 years of age and ~1-2 m in height). However, Pa or Pm of Acer saccharum, Tsuga canadensis, and Betula alleghaniensis increased from the sapling (~1 cm dbh) to later stages of larger size (~2-6 cm - [36]). Many studies have indicated that a reduction of photosynthesis with increasing tree age (or size) is primarily caused by the increase in the structural complexity and tree height, which lead to hydraulic constraints and further results in lower stomatal conductance ([4], [6]). However, age-related (or size-related) hydraulic limitations in younger trees do not act on photosynthesis as a key driving variable. Other factors, such as leaf characteristics (anatomy, morphology or chemistry), may also play a key role in age-related (or size-related) decline ([34]).
In this study, a decline in the maximum photosynthetic rate of A. indica × M. azedarach with increasing tree age may result in part from the decreased stomatal conductance, leading to a reduction in available CO2 in the leaf cells. A positive correlation between the photosynthetic rate (Pa or Pm) and the gs was observed (Tab. 3). Previous studies have also shown that the gs typically decreases with tree age or height ([34]). When the stomata are open and CO2 diffuses into the leaves, moisture evaporates through transpiration. The decline in stomatal conductance with increasing tree age leads to a decline in the transpiration rate. Although the photosynthesis rate also decreased with age, the magnitude of the decrease in gs was higher than that of Pa, which indicates that increased WUE occurs in older saplings. Nabeshima & Hiura ([22]) also confirmed that the increased WUE with an increase in plant size is related to the relatively higher magnitude of the decrease in stomatal conductance.
The photosynthesis of plants varied among different genotypes and habitats. Therefore, hybrids showing a photosynthetic physiology that differs from their parental species are better suited to certain habitats ([19]). Some studies reported that the physiological traits of hybrids had parental-like and transgressive traits ([19], [41], [33]). In this study, the maximal net photosynthetic rate (14.94 μmol m-2 s-1, based on area) of one-year-old A. indica × M. azedarach trees was close to that of one-year-old A. indica (15.13 μmol m-2 s-1, provenance from Myanmar - [18]). The introduced A. indica (from India) in the southwestern region of China showed that the maximal Pa, AQY, LSP, LCP, and Rd of 10-year-old A. indica varies from 9.46 to 11.58 μmol m-2 s-1, from 0.044 to 0.056 μmol m-2 s-1, from 1021.82 to 1205.31 μmol m-2 s-1, from 36.69 to 38.90 μmol m-2 s-1, and from 1.19 to 1.34 μmol m-2 s-1 depending on its source ([44]). In the current study, the gas exchange parameters of five-year-old A. indica × M. azedarach were close to the results of the above-described study, but the maximal net photosynthetic rate and LSP were significantly lower than those of local M. azedarach (P<0.05). This finding suggests that the gas exchange characteristics of A. indica × M. azedarach are more similar to those of the parental A. indica, and its light use efficiency is lower than that of the local parental species M. azedarach. Bassman & Zwier ([3]) showed that the values of gas exchange parameters in Populus trichocarpa × P. deltoides were in the range between those of the two parent species. The photosynthetic rate of the hybrid poplar Populus × euramericana Dode (Guinier) was generally higher than that of eastern cottonwood (Populus deltoides Bartr - [28]). Wu & Campbell ([41]) revealed that the gas exchange traits in Ipomopsis can be both intermediate and transgressive relative to those in the parental species. The performance of hybrids in terms of their photosynthetic physiology depended on the parental cytotype ([41]).
Changes in the gas exchange with increasing tree age (or size) are closely related to the leaf structure and biochemical properties. It is well known that the maximal photosynthetic rate is strongly affected by various leaf characteristics, such as the LMA, N and P concentrations, and N/P ([30], [12]). In the current study, the leaf N and P concentrations of the hybrid exhibited a decreasing trend with increasing tree age, whereas the leaf N/P and LMA increased with age. The maximum photosynthetic rates (Pa or Pm) were positively correlated with the leaf N and leaf P concentrations and were negatively correlated with the leaf N/P and LMA (Tab. 3). A linear regression analysis also indicated that leaf traits (i.e., LMA, TP and TN) played a leading role in age-dependent changes in hybrid photosynthesis (Tab. 4). A reduction in the nutrient availability with increasing tree age has been suggested to affect the age-dependent decrease in photosynthesis ([8]). Some studies have indicated that leaf N concentrations decrease as the tree size increases ([20], [39]). In contrast, other studies suggested that leaf N concentrations do not vary with increasing tree age (or size - [24], [23]) or may even decrease with increasing tree age (or size - [22]). A reduced N content clearly limits the formation of a high-capacity photosynthetic apparatus, and this effect is partly responsible for the size-dependent reductions in photosynthetic potentials ([20], [38]).
Although the LMA typically increases with increasing tree age, marked increases in LMAs were observed across age classes of less than 40 years, and the rate of change decreases across the mature age classes ([34]). A similar result was observed in this study. In conifers, the age- and size-dependent increase in LMA (LMA = leaf density × thickness) is primarily associated with enhanced leaf density, whereas both the thickness and density are increased in broad-leaved species ([7], [15], [25]). Many studies have reported that age-dependent (or size) increases in the LMA were possibly caused by greater water stress in the leaves of large trees ([2], [25]). The photosynthetic capacity is also influenced by the increased LMA, and this effect has been related to changes in the ratios of internal air space and mesophyll area to the total area. In addition, a larger LMA may also imply an increased respiration rate per leaf surface area, which is associated with a reduction in the net carbon gain per unit leaf area ([17]).
The PNUE and PPUE of the A. indica × M. azedarach were also found to decrease with increasing tree age. No significant differences in these two parameters were observed between AM3 and AM5 (P>0.05), but they were significantly higher in AM1 (P<0.05). On a global scale, the PPUE of trees increases when P availability decreases (soil P and leaf N/P), whereas the PNUE significantly increases with the decline in the photosynthetic rate through a larger LMA ([40]). When the N and P availabilities are low, a higher LMA would lead to a decline in the PNUE ([12]) because a larger proportion of N is invested in non-photosynthetic compounds. As a result, the N allocated to photosynthesis enzymes is reduced, leading to a decline in photosynthesis ([27], [35]). A higher LMA may also reduce photosynthesis through another mechanism, i.e., by extending the CO2 diffusion path from the stomata to mesophyll cells and then to chloroplasts, leading to a decrease in the light received by the chloroplast and a decline in the photosynthetic rate ([29], [10]). Our results show a decline in the maximal photosynthetic rate of A. indica × M. azedarach with increasing tree age, which is consistent with the above-mentioned processes of age-related reductions in stomatal conductance, leaf N, and leaf P and increased LMA.
Conclusions
The present study was aimed at investigating the photosynthetic physiological characteristics of A. indica × M. azedarach in its juvenile stages. Our results indicate that most photosynthetic gas exchange parameters of A. indica × M. azedarach decreased with increasing tree age, whereas the instantaneous water use efficiency (WUE) increased with age. The photosynthetic gas exchange parameters of AM3 and AM5 were significantly lower than the AM1 parameters . The reduced maximal photosynthetic rate of A. indica × M. azedarach with age was primarily related to the increase in the leaf mass per area (LMA) and a decrease in the leaf nitrogen (N) and leaf phosphorus (P) concentrations. Additionally, the decline in stomatal conductance (gs) was also an important factor leading to age-dependent reductions in the photosynthetic rate. The gas exchange characteristics of A. indica × M. azedarach were more similar to those of the parental A. indica, and its light use efficiency was lower than that of the local parental species M. azedarach.
These results may be useful in the management of cultivated stands of the hybrid A. indica × M. azedarach. For example, pruning and thinning must be conducted after the stands reach three years of age in order to enhance tree growth and seed output. Indeed, the high photosynthetic rate also implies that the hybrid needs higher light conditions in its juvenile stages and may be unsuitable for growth in the understory.
Acknowledgments
This project was funded by the Key Science and Technology Program of the Jiangsu Province “Processing Technology of Azadirachtin Pesticides” (BE2010618) and the Nanjing University Program “Research on Neem, Azadirachtin Pesticides, and Bio-energy Application” (2011320001010380). The authors give thanks to the Nanjing Jiukang Biological Development Co., Ltd. for supplying the study materials.
References
Gscholar
Gscholar
CrossRef | Gscholar
Gscholar
Gscholar
Authors’ Info
Authors’ Affiliation
Mukui Yu
Institute of Subtropical Forestry, Chinese Academy of Forestry, East China Research Station of Coastal Shelter Forest Ecosystem, Fuyang, Zhejiang 311400 (P.R. China)
College of Life Science, Nanjing University, Nanjing 210093 (P.R. China)
College of Forest Resources and Environment, Nanjing Forestry University, Nanjing 210037 (P.R. China)
Corresponding author
Paper Info
Citation
Cheng X, He Z, Yu M, Yin Z (2014). Gas exchange characteristics of the hybrid Azadirachta indica × Melia azedarach. iForest 8: 431-437. - doi: 10.3832/ifor1127-007
Academic Editor
Francesco Ripullone
Paper history
Received: Sep 17, 2013
Accepted: Aug 18, 2014
First online: Dec 17, 2014
Publication Date: Aug 02, 2015
Publication Time: 4.03 months
Copyright Information
© SISEF - The Italian Society of Silviculture and Forest Ecology 2014
Open Access
This article is distributed under the terms of the Creative Commons Attribution-Non Commercial 4.0 International (https://creativecommons.org/licenses/by-nc/4.0/), which permits unrestricted use, distribution, and reproduction in any medium, provided you give appropriate credit to the original author(s) and the source, provide a link to the Creative Commons license, and indicate if changes were made.
Web Metrics
Breakdown by View Type
Article Usage
Total Article Views: 43659
(from publication date up to now)
Breakdown by View Type
HTML Page Views: 37163
Abstract Page Views: 2419
PDF Downloads: 2909
Citation/Reference Downloads: 19
XML Downloads: 1149
Web Metrics
Days since publication: 3419
Overall contacts: 43659
Avg. contacts per week: 89.39
Article Citations
Article citations are based on data periodically collected from the Clarivate Web of Science web site
(last update: Feb 2023)
Total number of cites (since 2015): 1
Average cites per year: 0.11
Publication Metrics
by Dimensions ©
Articles citing this article
List of the papers citing this article based on CrossRef Cited-by.
Related Contents
iForest Similar Articles
Research Articles
Drought tolerance in cork oak is associated with low leaf stomatal and hydraulic conductances
vol. 11, pp. 728-733 (online: 06 November 2018)
Research Articles
Successional leaf traits of monsoon evergreen broad-leaved forest, Southwest China
vol. 10, pp. 391-396 (online: 16 March 2017)
Research Articles
Gas exchange, biomass allocation and water-use efficiency in response to elevated CO2 and drought in andiroba (Carapa surinamensis, Meliaceae)
vol. 12, pp. 61-68 (online: 24 January 2019)
Research Articles
Relationships between leaf physiognomy and sensitivity of photosynthetic processes to freezing for subtropical evergreen woody plants
vol. 12, pp. 551-557 (online: 17 December 2019)
Research Articles
Light acclimation of leaf gas exchange in two Tunisian cork oak populations from contrasting environmental conditions
vol. 8, pp. 700-706 (online: 08 January 2015)
Research Articles
A comparative study of growth and leaf trait variation in twenty Cornus wilsoniana W. families in southeastern China
vol. 10, pp. 759-765 (online: 02 September 2017)
Research Articles
Calibration of a multi-species model for chlorophyll estimation in seedlings of Neotropical tree species using hand-held leaf absorbance meters and spectral reflectance
vol. 9, pp. 829-834 (online: 17 May 2016)
Research Articles
Effect of environmental gradients on leaf morphological traits in the Fandoghlo forest region (NW Iran)
vol. 13, pp. 523-530 (online: 13 November 2020)
Research Articles
Leaf transpiration of drought tolerant plant can be captured by hyperspectral reflectance using PLSR analysis
vol. 9, pp. 30-37 (online: 05 October 2015)
Research Articles
First vs. second rotation of a poplar short rotation coppice: leaf area development, light interception and radiation use efficiency
vol. 8, pp. 565-573 (online: 27 April 2015)
iForest Database Search
Search By Author
Search By Keyword
Google Scholar Search
Citing Articles
Search By Author
Search By Keywords
PubMed Search
Search By Author
Search By Keyword