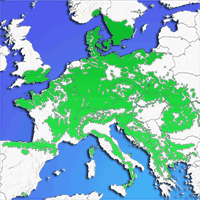
Adaptive variation in physiological traits of beech provenances in Central Europe
iForest - Biogeosciences and Forestry, Volume 11, Issue 1, Pages 24-31 (2018)
doi: https://doi.org/10.3832/ifor2291-010
Published: Jan 09, 2018 - Copyright © 2018 SISEF
Research Articles
Abstract
Current climate changes can led to a decline of local beech populations fully adapted to previous climate conditions. In this context, the issue of variation in adaptive traits becomes important. A field experiment with 18-year-old trees of Fagus sylvatica L. was conducted on provenance plot located in Tále (Central Slovakia), where physiological responses of five beech provenances originating from contrasting sites along an altitudinal gradient from 55 to 1100 m a.s.l. across the range of the natural beech distribution were studied. Stomatal characteristics, parameters of chlorophyll a fluorescence and gas exchange parameters were determined. Overall, we observed a significant increase in physiological performance at the leaf level with increasing altitude of origin. Provenances from the higher altitudes showed higher CO2 assimilation rate, stomatal density, potential conductance indices and photochemical efficiency, and lower capability for dissipation of energy by heat. A similar pattern of response was recorded in relation to the precipitation regime of sites of origin. Moreover, in the context of the temperature regime, several negative trends were observed.
Keywords
Adaptation, Provenance Trial, Fagus sylvatica L., Chlorophyll a Fluorescence, Stomatal Traits, Gas Exchange
Introduction
Global change will exert strong evolutionary pressure on trees over the upcoming decades. As populations are largely genetically adapted to the local climate, rapid changes may challenge the ability of tree species to adapt, and migration to new locations might be problematic ([40]). Because of the current climate change and its consequences to forest ecosystems, foresters and naturalists think about future ecotypes of woody species with functional traits allowing them to grow and adapt to a changing environment ([32]). Therefore, for the stability of future forests, selection of proper tree populations is particularly important, whereas genetic variability and phenotypic plasticity are the key factors for the identification of useful genotypes ([10]).
What is the natural capacity of tree species to cope with rapid environmental changes such as the current climate change and what are the limits of human interference in this respect is still debated. The rate of adaptation of trees to environmental change depends on successful recruitment events during their lifetime, whereas only morphological and physiological adjustments allow individuals to persist in a stressful environment ([18]). In this context, it is desirable to better understand the physiological processes that underlie growth and production, focusing on traits strongly related to biological fitness, such as photosynthesis and respiration, as well as traits relevant in the context of climate change, such as water balance, drought and heat resistance, etc. ([16], [47], [42]). As changes in environmental conditions occur along altitudinal gradients even over short distances, such gradients offer a powerful experimental setup for studying the ecological response of plants to changing environments. Moreover, spatial variation in functional traits along an altitudinal gradient can also illustrate the trends associated with plant responses to climate changes ([27], [37]). Several studies have recorded the gradual changes of ecophysiological (photosynthetic capacity, stomatal conductance, water use efficiency, etc.) and morphological traits of leaves (stomatal density, leaf mass per area, thickness of leaf lamina, etc.) along an altitudinal gradient, as well as the morphologically and physiologically distinctiveness of high-altitude populations in comparison to related populations at lower altitudes ([1], [41], [8], [20], [33], [45], [11]).
Provenance analysis represent a potential approach to simulate the shift of climatic characteristics, allowing to assess the phenotypic responses of populations to transfer and the identification of well growing and adapted populations with higher resistance against adverse environmental factors. The aim of provenance trials is to minimize differences among different populations due to large-scale environmental effects by growing plant material of different origins under common conditions ([12]). Because in provenance experiments trees grow in a different environment compared to that to which they were originally adapted, such experiments could help predict the responses of tree species to changing climates. Provenance experiments have shown that tree species are able to cope with a wide range of environmental conditions. It is likely that seasonal acclimation dynamics is an important element of adaptation ([6]).
European beech (Fagus sylvatica L.) is the main species of deciduous forests in Central Europe. It represents the most competitive tree species on sites with moderate soil moisture and acidity, widespread across this region ([7]). Because beech is an important timber species in Central Europe, it is essential to guarantee the vitality and productivity of future beech stands. In the context of the predicted changes in annual distribution and amount of precipitation, beech stands are considered endangered due to their relatively high drought sensitivity.
The main aim of this study was to assess the adaptive variation in physiological traits of beech provenances in relation to environment of their origin, which refers to adaptation of provenances by natural selection over generations.
Material and methods
Experimental material
A large provenance experiment with European beech (Fagus sylvatica L.) was established in 1998 under the coordination of the Federal Forest Research Centre, Institute of Forest Genetics in Grosshansdorf, Germany, using two-year-old seedlings. In situ measurements and samplings were conducted in July and August 2014 on the trial plot located in Tále (Central Slovakia - 48° 38′ N, 19° 02′ E, elevation 810 m a.s.l.). The plot is situated in a region characterized by moderate climate conditions, at the natural climatic optimum of beech distribution (Tab. 1). The design of the experiment included three completely randomized and continuous blocks. Each block included initially 50 plants planted at 2×1 m spacing ([17]).
Tab. 1 - List of beech populations with geographical and climatic characteristics. (TA): mean annual temperature (°C); (T59): mean temperature during vegetation season (°C); (PA): mean annual precipitation (mm); (P59): mean amount of precipitation during vegetation season (mm).
Label | Country | Name | Longitude N |
Latitude E |
Elevation (m a.s.l.) |
TA | T59 | PA | P59 |
---|---|---|---|---|---|---|---|---|---|
Trial plot | Slovakia | Tále | 19°03′ | 48°63′ | 850 | 6.6 | 14.1 | 842 | 441 |
26DE | Germany | Farchau | 10°40′ | 53°39′ | 55 | 8.3 | 15 | 678 | 319 |
30DE | Germany | Belzig | 12°25′ | 52°03′ | 140 | 8.6 | 15.6 | 558 | 282 |
39PL | Poland | Jaworze | 19°10′ | 49°50′ | 450 | 6.3 | 13.9 | 903 | 533 |
55SI | Slovenia | Postojna Javor | 14°21′ | 45°44′ | 1040 | 8.1 | 15 | 1345 | 586 |
36AT | Austria | Eisenerz | 14°51′ | 47°32′ | 1100 | 2.9 | 10 | 1168 | 648 |
Five provenances of European beech originating from different climatic regions were selected for the trial (Tab. 1, Fig. 1). All the selected provenances originated from the Slovenian glacial refugium to minimize the differences caused by the neutral evolutionary processes. All of the measurements were done on the same set of three individuals from two randomly selected blocks for each provenance. The experiment was carried out to study the physiological status of beech provenances via photosynthesis efficiency (using gasometry and chlorophyll a fluorescence kinetics) and stomatal characteristics.
Fig. 1 - Origin of the tested provenances (circles) and the trial plot Tále (Central Slovakia, indicated by a star). The grey area represents the potential natural range of Fagus sylvatica in Europe (adapted from EUFORGEN 2015 - ⇒ http://www.euforgen.org).
Chlorophyll a fluorescence/Rapid Light Curves (RLCs)
Parameters of chlorophyll a fluorescence were recorded on three leaves per provenance in two replicates, using a portable chlorophyll fluorometer MINI-PAM® (Heinz Walz GmbH, Germany). RLCs were measured automatically under the control of an internal programme. The actinic illumination was applied in eight steps, with light intensities increasing from 38 to 616 µmol (photon) m-2 s-1 and duration of 10 s. The illumination periods were separated by a 0.8-s saturating flash from a white halogen lamp (2000-3000 µmol [photon] m-2 s-1). The following parameters were determined and/or calculated in RLCs measurements: (i) effective quantum yield of the PSII (Φ
PSII=ΔF/F′m); (ii) coefficient of photochemical quenching (qP - [15], [50]); (iii) coefficient of non-photochemical quenching (qN); and (iv) non-photochemical quenching (NPQ). All above parameters are indicators of excess light energy and heat-dissipation of excitation energy in the antenna system ([50]). The RLCs were quantified using: (i) the initial slope for RLCs of Φ
PSII and qP - slopes were evaluated by direct calculation using linear regression of four initial data points; and (ii) value of the end point - measured at 616 µmol (photon) m-2 s-1 PAR - for RLCs of NPQ and qN.
Stomatal characteristics
Leaf samples were harvested from the sunny part of the upper third of the crown. Stomatal imprints were taken from six leaves per provenance using the colloid method. A thin coat of colourless nail polish was applied on the abaxial leaf side. After drying, the polish was peeled off using transparent tape and was stuck on a microscopic slide. All of the imprints were taken from the middle part of the leaf to avoid possible variation between the leaf base and tip ([9]). The imprints were visualized using the light microscope Olympus BX50® connected with the digital camera Canon DS126491® (Canon Inc., Japan) and were evaluated using the software for picture analyses NIS Elements AR 3.0 (Laboratory Imaging, Czech Republic). Stomatal density (SD), length of guard cells (LA) and potential conductance index (PCI) were determined. Stomatal density was counted from two 500×500 μm leaf areas at 20×10 magnification and converted into mm2 of leaf area. For the assessment of guard cells length, 15 stomata per imprint were chosen at the magnification of 40×10. The potential conductance index was calculated according to Holland & Richardson ([20]) using the following relationship: PCI= LA2·SD·10-4.
Gas exchange
Leaf gas exchange measurements were carried out using the gasometric system Li-6400XT® equipped with the chamber fitted with 6400-02B LED light source (LI-COR Biosciences, USA). Measurements were performed on three leaves per three trees per provenance in two replicates (18 leaves per provenance). All of the measurements were taken before the midday depression of photosynthesis appeared, on fully expanded leaves around 2 m above the ground. The leaves were exposed to a CO2 concentration of 385 µmol mol-1 and the saturating PAR of 1300 µmol m-2 s-1. Gas exchange was measured at the air temperature in the chamber at 20.5 ± 0.5 °C. The values were evaluated after a short adaptation period when the CO2 assimilation values remained stable. CO2 assimilation rate (A), stomatal conductance (gS) and transpiration rate (E) were determined.
Data analysis
The obtained data were processed using the statistical program STATISTICA® (StatSoft Inc., Tulsa, OK, USA). Prior to the statistical tests, the normal distribution of the data was tested. Because the distributions of several physiological parameters deviated from the normal distribution, data were square-root transformed. Differences in the measured parameters among provenances were tested using ANOVA, and pairwise contrasts were tested using Tukey’s post-hoc tests with α
= 0.05. For the assessment of altitudinal and climatic trends, provenance averages were fitted to linear regression models with the altitude and climate characteristics of their sites of origin.
Results
Chlorophyll a fluorescence/RLCs
We found differences among the studied provenances related to the course of RLCΦPSII (slope of RLCΦPSII - Fig. 2a, Fig. 3a) as well as RLCqP (slope of RLCqP - Fig. 2b, Fig. 3b). From the point of view of altitude, the response of the provenance originating from the highest altitude (36AT, 1100 m a.s.l.) deviated from those of the other studied provenances (Fig. 2a, Fig. 2b, Fig. 3a, Fig. 3b), but the responses were not consistent along the altitudinal gradient. However, in connection with the dissipation of energy by heat (NPQ), the provenances from high altitudes 36AT and 55SI (1100 and 1040 m a.s.l., respectively) showed the lowest capability. A higher ability for the dissipation of energy by heat was recorded for populations 26DE and 39PL originating from the lower part of the altitudinal range of the studied provenances (Fig. 2c, Fig. 2d, Fig. 3c, Fig. 3d). The effect of precipitation regime at the sites of origin was also not confirmed. However, slight increases of the Φ
PSII and qP along the gradient of the mean annual temperature and the temperature during the vegetation season were recorded.
Fig. 2 - Rapid Light Curves (RLCs) of the tested provenances. RLCs for the effective quantum yield of PSII (Φ
PSII - panel a), coefficient of photochemical quenching (qP - b), nonphotochemical quenching (NPQ - panel c) coefficient of nonphotochemical quenching (qN - panel d) are displayed. The data presented are the means.
Fig. 3 - Quantification of Rapid Light Curves (RLCs) of the tested provenances. The RLCs for the effective quantum yield of PSII (Φ
PSII - panel a) and the coefficient of photochemical quenching (qP - panel b) were quantified by the derivation of curve slopes. For the quantification of RLCs of non-photochemical quenching (NPQ - panel c) and the coefficient of non-photochemical quenching (qN - panel d) values of the end points - measured at 616 µmol (photon) m-2 s-1 PAR - were used. The data are presented as provenance means and standard errors of the mean (whiskers). Different letters indicate significant differences among provenances after Tukey’s test (p<0.05).
Stomatal characteristics
Overall, we found a significant effect of altitude on all of the stomatal traits (Tab. 2). The mean stomatal density (SD
) gradually increased with altitude (R2=0.782; P≤0.05), however, the differences between the provenances were not statistically significant due to a high variability (Fig. 4a). The Slovenian provenance 55SI (1040 m a.s.l.) reached the highest value of the SD
(on average, 258.7 ± 33.7 stomata mm-2). A significant increase along the altitudinal gradient (R2=0.932; P≤0.01) was also observed in the potential conductance indices increasing from 12.7 ± 2.0 for 26DE to 16.7 ± 3.1 for 36AT. A marginally significant increase in the guard cell lengths was found (R2=0.7327; P≤0.10), with values ranging from 23.9 ± 2.5 μm for 30DE up to 25.9 ± 4.4 μm for 36AT. Moreover, analysis of variance confirmed that the provenance from Austria 36AT achieved the significantly highest values for both parameters (Fig. 4b, Fig. 4c). A similar pattern of reaction was also observed in relation to precipitation regime of the sites of origin (Tab. 2). Significant or at least marginally significant effects of the annual precipitation or the precipitation during the vegetation season on all of the monitored parameters were observed. Moreover, we noticed a negative effect of increasing mean annual temperature and mean temperature during the vegetation season on the length of guard cells (R2=0.823; P≤0.05 and R2=0.873; P≤0.05, respectively).
Tab. 2 - Linear regressions of the provenance averages of measured parameters against the altitude and climatic traits of their origin. (A): assimilation rate (µmol m-2 s-1); (gs): stomatal conductance (µmol m-2 s-1); (E): transpiration rate (µmol m-2 s-1); (SD): stomatal density (stomata mm-2); (LA): length of guard cells (μm); (PCI): potential conductance index; (RLCΦPSII): initial slope of RLCΦPSII; (RLCqP): initial slope of RLCqP; (RLCNPQ): NPQ measured at PAR of 616 µmol (photon) m-2 s-1; (RLCqN): qN measured at PAR of 616 µmol (photon) m-2 s-1; (ALT): altitude; (PA): mean annual precipitation; (P59): mean amount of precipitation during vegetation season; (TA): mean annual temperature; (T59): mean temperature during vegetation season; (*): p≤0.05; (**): p≤0.01; (1): marginally significant at p≤0.10; (ns): non-significant.
Variable | Parameter | Gas Exchange | Stomatal Traits | RLCs Initial Slope | RLCs End Point | ||||||
---|---|---|---|---|---|---|---|---|---|---|---|
A | g s | E | SD | LA | PCI | RLCΦPSII | RLCqP | RLCNPQ | RLCqN | ||
ALT (m a.s.l.) |
Slope | 0.0018 | ns | ns | 0.0351 | 0.0015 | 0.004 | ns | ns | ns | ns |
R2 | 0.877 | ns | ns | 0.787 | 0.733 | 0.932 | ns | ns | ns | ns | |
Prob | 0.0192* | ns | ns | 0.045* | 0.0641 | 0.008** | ns | ns | ns | ns | |
PA (mm) |
Slope | 0.0028 | ns | ns | 0.052 | ns | 0.0056 | ns | ns | ns | ns |
R2 | 0.897 | ns | ns | 0.775 | ns | 0.902 (1) | ns | ns | ns | ns | |
Prob | 0.014* | ns | ns | 0.049* | ns | 0.036* | ns | ns | ns | ns | |
P59 (mm) |
Slope | 0.0057 | 0.0001 | 0.001 | ns | 0.0043 | 0.0106 | ns | ns | ns | ns |
R2 | 0.924 | 0.676 | 0.697 | ns | 0.7083 | 0.708 | ns | ns | ns | ns | |
Prob | 0.009** | 0.0881 | 0.0781 | ns | 0.0741 | 0.0741 | ns | ns | ns | ns | |
TA (°C) |
Slope | ns | -0.0086 | -0.0729 | ns | -0.3197 | ns | -0.0006 | -0.0008 | ns | ns |
R2 | ns | 0.948 | 0.857 | ns | 0.823 | ns | 0.922 | 0.89 | ns | ns | |
Prob | ns | 0.005** | 0.024* | ns | 0.034* | ns | 0.010** | 0.016* | ns | ns | |
T59 (°C) |
Slope | ns | -0.0087 | -0.0727 | ns | -0.3458 | ns | -0.0006 | -0.0007 | ns | ns |
R2 | ns | 0.886 | 0.772 | ns | 0.873 | ns | 0.846 | 0.798 | ns | ns | |
Prob | ns | 0.017* | 0.050* | ns | 0.020* | ns | 0.027* | 0.041* | ns | ns |
Fig. 4 - Stomatal traits of the tested provenances. Stomatal density (SD - panel a), guard cell lengths (LA - panel b) and potential conductance indices (PCI - panel c) are displayed. The bar chart represents the provenance means and standard errors of the mean. The different letters indicate that values differ significantly among provenances after Tukey’s test (P<0.05).
Gas exchange characteristics
Based on the analysis of variance, we recorded statistically significant differences among provenances (Fig. 5a, Fig. 5b, Fig. 5c) in terms of CO2 assimilation rate values, stomatal conductance and transpiration. Overall, we found a linear increase along the altitudinal gradient for all of the monitored traits. However, a significant linear trend of altitude was found only for the CO2 assimilation rate (R2=0.877; P=0.05). We also recorded similar trends in relation to mean annual precipitations of the sites of origin (R2=0.897; P≤0.05) and to the precipitation during the vegetation season (R2=0.7327; P≤0.10). However, increasing mean annual temperature and mean temperature during the vegetation season had significant negative effects only on the stomatal conductance (R2=0.948; P≤0.01 and R2=0.886; P≤0.05, respectively) and the transpiration (R2=0.857; P≤0.05 and R2=0.772; P≤0.05, respectively). The Austrian provenance 36AT (1100 m a.s.l.) generally displayed the highest values of CO2 assimilation rate (6.92 µmol CO2 m-2 s-1), stomatal conductance (0.15 mol CO2 m-2 s-1) and transpiration (1.58 mol H2O m-2 s-1). In comparison, the least-vital provenance 30DE (140 m a.s.l.) reached approximately 34% lower values of A (4.55 µmol CO2 m-2 s-1) and gs (0.1 mol CO2 m-2 s-1), and approximately 28% lower values of E (1.13 mol H2O m-2 s-1).
Fig. 5 - Parameters of gas exchanges of the tested provenances. CO2 assimilation rate (A - panel a), stomatal conductance (gs - panel b) and transpiration (E - panel c) are displayed. The bar chart represents the provenance means and standard errors of the mean. The different letters indicate that values differ significantly among provenances after Tukey’s test (P<0.05).
Discussion
Provenance trials provide the basis to observe the response of provenances to different environmental conditions ([30]). In our study, we try to determine levels of adaptation of beech provenances to climate conditions at the trial site in relation to the climate of origin. Several recent studies have focused on the suitability of environmental conditions at different altitude ranges of plant species ([39], [20]) and also on the effect of stress factors differing among plots ([23]). The divergence between populations is driven by the differences in the selective pressure imposed by different ecological environments, neutral evolutionary processes or both ([41]); in our case, when the distances between the studied populations are quite large, the role of neutral factors such as isolation by distance or genetic drift may be important, but we tried to minimize such effects by choosing populations originating from the same glacial refugium (Slovenia/Istria - [29]). The dynamic and thermo-dynamic processes at high altitudes lead to the formation of regional-scale atmospheric circulation with wind systems, temperatures and precipitation regimes, etc., differing from general precipitation and negative temperature gradient along the gradient of altitude. Our results showed that beech provenances originating from altitudinal extremes are substantially different in most ecophysiological and morphological traits. The provenances originating from localities with lower amounts of annual precipitation and higher temperatures, i.e., 26DE and 30DE, responded very sensitively to the conditions of the trial plot. On the contrary, the provenances 55SI and 36AT originating from cool and wet sites show favourable values of the measured physiological parameters. As reported by Bohn ([7]) and Rose et al. ([38]), this result may be influenced by a smaller shift in terms of climate between the site of origin and the trial plot. These observations are also very closely related to increasing continentality influencing water availability under the conditions of the trial plot, where the populations originating from areas with a subcontinental climate (i.e., 26DE, 30DE, 39PL) appear as highly responsive. Several monitored parameters showed an increase with increasing altitude and relations to climatic gradients. These reaction patterns are most probably acquired by genetically-driven responses to conditions of the origin sites and are fixed across the life span of each individual ([34]).
The methods of evaluation of chlorophyll a fluorescence (fast and slow kinetics of chlorophyll a fluorescence) are rapid, nondestructive and non-invasive, and helpful in the detection of changes in the photosynthetic process caused by abiotic stress before visible signs of deterioration are apparent to the naked eye ([43]). These methods are generally used to assess plant vitality and performance in relation to different conditions ([14], [51], [36], [24]). Despite the fact that none of the chlorophyll a fluorescence parameters exhibited a smooth trend along the altitudinal gradient, some clear climatic trends or differences between contrasting groups of provenances were observed. These trends correspond well with the observed photosynthetic performance (CO2 exchange) and stomatal traits. The results of chlorophyll a fluorescence assessment indicated the best photosynthetic performance in the conditions of the trial plot for the provenances 55SL and 36AT originating from cooler climates. Moreover, substantial downregulation of Φ
PSII and qP was recorded for the 26DE provenance originating from warmer and drier climate compared to the 36AT provenance, which means that a large fraction of the absorbed irradiance was not utilized via photochemical reaction in the 26DE provenance. This result is confirmed by increasing NPQ levels for the 26DE provenance, indicating reduced electron flow ([11]). However, it is also known that higher values of NPQ indicate the ability of plants to mitigate the negative impact of stress conditions at the chloroplast level through the dissipation of excessive excitation energy ([22], [19]). Plants display a variety of physiological adaptations to prevailing changing environmental conditions in different ways at the chloroplast level.
The ability of plants to regulate the exchange of water vapour and CO2 through stomata is an important feature characterizing the status of plants in their environment. Effective stomata control is important for plant growth and survival, especially when water supply is limited, but also in terms of their competitive capabilities and the expansion of space in which they are able to grow ([44], [4], [2]). Moreover, stomatal control in European beech is often unable to maintain the shoot water potential above the critical limit, thereby preventing the loss of hydraulic conductivity ([3], [13]). The increased stomatal density and stomatal size are considered to improve the plant efficiency of CO2 uptake. However, their decrease might reflect a decrease in the relative air humidity and hence it could be associated with plant water-saving strategy ([39], [31]).
Several studies reported changes in stomatal traits with increasing altitude in various plant species including beech; PCI, guard cell length or stomatal density were observed to increase with altitude ([21], [26], [8], [33], [45]), presumably compensating for a decrease of CO2 partial pressure, which limits photosynthetic potential across altitudinal gradients, shorter growing seasons and/or increasing UV radiation ([46], [26], [45]). The increase in stomatal density and index with altitude could be also result of opening up of the higher altitude landscape, which increases the amount of intercepted radiation ([21]). Lower precipitation and water availability in lower altitudes strongly influence stomatal density and epidermal cell density as well ([28]). This was confirmed by Pyakurel & Wang ([35]) who recorded higher stomatal density by birch provenances originating from areas with higher annual precipitation and lower temperatures. However, mentioned studies focused on phenotypic stomatal variation along vertical transects within one mountain range. Therefore, in contrast with provenance trials, the conditions of growth are unequal, and the differences among populations might be influenced by large-scale variation of environmental factors. Although a study on the stomatal analysis of beech in a provenance trial was also published ([42]), the authors focused on how stomatal traits (stomatal density, width of stomatal pore, potential conductance index, relative stomatal pore surface and length of guard cells) of beech provenances react to drought stress during two years characterized by contrasting weather conditions. Similarly, Xu & Zhou ([48]) studied the response of stomatal traits in Leymus chinensis to a large range of water statuses. Although these studies suggest the possibility of a plastic response of populations toward changes in soil water regime influenced by the prevailing weather, the absence of geographic and climatic trends did not provide an answer to the question whether stomatal characteristics are affected only by the current environmental conditions (acclimation to higher precipitation regime and lower temperatures at higher altitudes), or whether environmental conditions of origin drive adaptation at the population level. However, significant climatic trends in stomatal traits revealed in our study support the assumption that genetic effects on stomata traits do exist.
Early studies noted a positive correlation between the leaf stomatal density and stomatal conductance gs ([46], [48]). Stomatal conductance is a primary determinant of net carbon balance and growth of plant species, and because of its influence on water loss through transpiration, stomatal conductance determines the water balance of a plant. Moreover, plants with higher gs values may be able to assimilate CO2 more rapidly than those with a lower conductance ([9]). Our results showed that CO2 assimilation rate (A), stomatal conductance (gs) and transpiration (E) increased with increasing altitude. These trends were documented by Kohout & Read ([25]), Premoli & Brewer ([34]) and Zhang et al. ([49]) as well. Körner ([27]) suggested that trees carry out a relatively high level of photosynthesis to compensate for extreme environmental conditions and short growing season at high altitudes. This hypothesis was confirmed also by Bresson et al. ([8]), who noted that the CO2 assimilation rate increased significantly with increasing altitude, approximately 2.8 µmol CO2 m-2 s-1 per 1000 m for beech. The small increase in maximum assimilation rate at high altitude may be attributed to a slight increase in stomatal conductance, implying an increase in CO2 diffusion into the leaf. Premoli & Brewer ([34]) showed that CO2 assimilation rates were 40% higher for high-altitude plants in the field than those for low-altitude plants in Nothofagus pumilio, although they assumed that assimilation was under genetic control. Moreover, our results pointed to positive effect of increasing amount of precipitation and negative effect of increasing temperature to gas exchange. Also, the highest values of Amax and gs were recorded in Picea glauca trees growing on the site with the higher precipitation and lower mean annual temperature ([5]).
Conclusions
For the stability and productivity of future forests, it is particularly important to choose suitable provenances that could be used as seed sources for future reforestation programmes. Based on our results, we may conclude that provenances from cooler and wetter climate, originating from the central part of beech distribution (i.e., conditions roughly similar to the environment of the trial plot Tále) showed higher physiological performance under the conditions of Central Europe compared to the provenances that have experienced a larger shift in climate between the site of origin and the trial plot. The knowledge of physiological responses of beech to environmental conditions in direct response to its geographical origin will help to evaluate the impact of climate changes on populations of the forest tree and mitigate these changes.
Acknowledgements
LD, DG conceived the ideas and designed the study; JK, DK, GJ, EP, AK, KS carried out the measurements, processed and interpreted of data; JK, AK wrote the first version of manuscript, and all of the authors contributed critically to the drafts and gave final approval for publication.
We would like to thank Tibor Priwitzer and Peter Petrík for their technical assistance during this experiment and K. Willingham for language correction.
The experiment was supported by the grant of the Slovak Agency for Research and Development APVV-0135-12 (0.2), the grant of the Slovak Grant Agency for Science VEGA 0034-14 (0.8).
List of Abbreviations
The following abbreviations have been used throughout the paper:
- RLC: rapid light curve of chlorophyll a fluorescence;
Φ
PSII: effective quantum yield of PSII (yield, ΔF/F′m) ;- qP: coefficient of photochemical quenching;
- qN: coefficient of non-photochemical quenching;
- NPQ: non-photochemical quenching;
- RLCΦPSII: rapid light curve of the effective quantum yield of PSII;
- RLCqP: rapid light curve of the coefficient of photochemical quenching;
- RLCqN: rapid light curve of the coefficient of non-photochemical quenching;
- RLCNPQ: rapid light curve of the non-photochemical quenching;
- SD: stomatal density;
- LA: length of stomata guard cells;
- PCI: potential conductance index, calculated as PCI= LA2·SD·10-4;
- A: assimilation rate;
- gS: stomatal conductance;
- E: transpiration rate.
References
CrossRef | Gscholar
Gscholar
Gscholar
CrossRef | Gscholar
Gscholar
Authors’ Info
Authors’ Affiliation
Eva Pšidová
Gabriela Jamnická
Kristína Slugenová
Lubica Ditmarová
Institute of Forest Ecology, Slovak Academy of Sciences, Zvolen (Slovakia)
Daniel Kurjak
Dušan Gömöry
Faculty of Forestry, Technical University in Zvolen, Zvolen (Slovakia)
Corresponding author
Paper Info
Citation
Kučerová J, Konôpková A, Pšidová E, Kurjak D, Jamnická G, Slugenová K, Gömöry D, Ditmarová L (2018). Adaptive variation in physiological traits of beech provenances in Central Europe. iForest 11: 24-31. - doi: 10.3832/ifor2291-010
Academic Editor
Angelo Nolè
Paper history
Received: Nov 23, 2016
Accepted: Oct 12, 2017
First online: Jan 09, 2018
Publication Date: Feb 28, 2018
Publication Time: 2.97 months
Copyright Information
© SISEF - The Italian Society of Silviculture and Forest Ecology 2018
Open Access
This article is distributed under the terms of the Creative Commons Attribution-Non Commercial 4.0 International (https://creativecommons.org/licenses/by-nc/4.0/), which permits unrestricted use, distribution, and reproduction in any medium, provided you give appropriate credit to the original author(s) and the source, provide a link to the Creative Commons license, and indicate if changes were made.
Web Metrics
Breakdown by View Type
Article Usage
Total Article Views: 46717
(from publication date up to now)
Breakdown by View Type
HTML Page Views: 39983
Abstract Page Views: 2750
PDF Downloads: 3063
Citation/Reference Downloads: 30
XML Downloads: 891
Web Metrics
Days since publication: 2508
Overall contacts: 46717
Avg. contacts per week: 130.39
Article Citations
Article citations are based on data periodically collected from the Clarivate Web of Science web site
(last update: Feb 2023)
Total number of cites (since 2018): 10
Average cites per year: 1.67
Publication Metrics
by Dimensions ©
Articles citing this article
List of the papers citing this article based on CrossRef Cited-by.
Related Contents
iForest Similar Articles
Research Articles
Magnolia grandiflora L. shows better responses to drought than Magnolia × soulangeana in urban environment
vol. 13, pp. 575-583 (online: 07 December 2020)
Research Articles
Wintertime photosynthesis and spring recovery of Ilex aquifolium L.
vol. 12, pp. 389-396 (online: 31 July 2019)
Research Articles
Tracing the acclimation of European beech (Fagus sylvatica L.) populations to climatic stress by analyzing the antioxidant system
vol. 14, pp. 95-103 (online: 01 March 2021)
Research Articles
Light acclimation of leaf gas exchange in two Tunisian cork oak populations from contrasting environmental conditions
vol. 8, pp. 700-706 (online: 08 January 2015)
Research Articles
Relationships between leaf physiognomy and sensitivity of photosynthetic processes to freezing for subtropical evergreen woody plants
vol. 12, pp. 551-557 (online: 17 December 2019)
Research Articles
Calibration of a multi-species model for chlorophyll estimation in seedlings of Neotropical tree species using hand-held leaf absorbance meters and spectral reflectance
vol. 9, pp. 829-834 (online: 17 May 2016)
Research Articles
Juvenile growth response of European beech (Fagus sylvatica L.) to sudden change of climatic environment in SE European trials
vol. 2, pp. 213-220 (online: 22 December 2009)
Short Communications
Effect of four levels of shade on survival, morphology and chlorophyll fluorescence of Nothofagus alessandrii container-grown seedlings
vol. 8, pp. 638-641 (online: 08 January 2015)
Research Articles
Links between phenology and ecophysiology in a European beech forest
vol. 8, pp. 438-447 (online: 15 December 2014)
Research Articles
Drought tolerance in cork oak is associated with low leaf stomatal and hydraulic conductances
vol. 11, pp. 728-733 (online: 06 November 2018)
iForest Database Search
Search By Author
Search By Keyword
Google Scholar Search
Citing Articles
Search By Author
Search By Keywords
PubMed Search
Search By Author
Search By Keyword