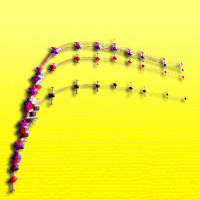
Biochar amendment regulated growth, physiological, and biochemical responses of conifer in red soil
iForest - Biogeosciences and Forestry, Volume 13, Issue 6, Pages 490-498 (2020)
doi: https://doi.org/10.3832/ifor3416-013
Published: Nov 01, 2020 - Copyright © 2020 SISEF
Research Articles
Abstract
The addition of Biochar (BC) into the soil is expected to improve soil physicochemical properties and plant growth. However, few studies have verified such an effect on the growth and physiological characteristics of conifers. The current study aims to assess the efficacy of novel physiological parameters as an indicator for assessing the impact of hardwood biochar (BH) on the development of Fokienia hodginsii seedlings to strengthen our understanding of the impacts of the BH on soil to optimize the achievement of BC-based restoration projects. The BH was applied to the soil under four different levels (0, 5, 20, and 80 g Kg-1 of soil) to assess their influence on the leave’s photosynthetic pigments, photosynthesis (Pn), and biochemical traits of F. hodginsii seedlings in four different seasons, and on biomass and soil physicochemical properties at final harvest under greenhouse conditions for one year. In the first two seasons, BH20 and BH80 amended seedlings responded with an improved photosynthetic rate with more production of photosynthetic pigments and biochemical attributes. However, none of the BC doses increased the Pn of seedlings in the final season. Nonetheless, after one year a rise in soil pH as well as P and K availability resulted in a maximum 25% increase in biomass of F. hodginsii under BH80 amendments. Our findings reveal that the incorporation of BH (20 and 80 g kg-1 of soil) has a substantial positive effect on seedling biomass and soil fertility. However, the application of BH into acidic soils may be effective in restoring degraded soils if initially combined with fertilizers. We recommend a careful approach to the selection of BC because its influence may vary between different soil types, plant species, and BC feedstocks.
Keywords
Fokienia hodginsii, Hardwood Biochar, Restoration, Photosynthesis
Introduction
Soil fertility is a key factor of forest productivity and its long-term maintenance without harming the environment is a crucial and challenging goal. A comprehensive understanding is required to evaluate the soil’s ability to ensure plant growth. Recently, the use of biochar (BC) as an organic amendment has become an alternative to returning biomass into the soil to address numerous agronomic and environmental problems ([33]). BC is a rich carbonaceous material obtained from biomass via the thermochemical process (pyrolysis) under limited or no oxygen ([47]). Owing to its advantageous properties (rich carbon C and larger surface area), its application into the soil is expected to improve the soil fertility and biological activity ([24]). Its compositions make it stable in many soils from a hundred years to potentially a thousand years ([19]).
Fokienia hodginsii (Dunn) A. Henry & H.H. Thomas (Fujian cypress) is a valuable tree species native to southern China, Vietnam, and Laos. It has received much attention in China because of its high-quality timber (export) and aromatic essential oil (Siam-wood essential oil). During the last few decades, its populations have been reduced and recently it is listed as threatened in the red list of IUCN (International Union for Conservation of Nature).
Large areas in tropical and subtropical regions of southern China are characterized by acidic soils, which hinders plant growth by affecting many physiological processes ([20]). The physiological characteristics of a plant are regarded as a primary indicator of its health and fitness. For instance, photosynthetic activity mainly relies on the concentration of chlorophyll pigments ([11]), which is related to the nitrogen (N) status of the plant, and carotenoid concentration is a proxy of plant sensitivity to light intensity ([44]). Biochemical traits such as amino acids, total soluble proteins, etc. are environmentally controlled features that vary in different seasons ([54]). Yet, there is limited information regarding the response of the physiological attributes of forest trees to the BC amendment to develop sustainable strategies for forest management.
Several investigations have been focused on the impact of BC as an amendment on plant growth and soil, mostly on crops rather than forest tree species. For instance, Uzoma et al. ([46]) reported that BC amended soil increased the soil cation exchange capacity and the availability of nutrients which improved maize growth and yield. Moreover, seedlings of Solanum lycopersicum and Oryza sativa emerged rapidly under soil amended with rice husk BC ([3]). Lusiba et al. ([27]) demonstrated that the addition of BC significantly enhanced the biomass of chickpea combined with P fertilizer in clay soil. Recently, BC mixed in growing media promoted the biomass of lettuce and basil ([33]).
To date, there are limited BC studies that support such an influence on the physiology and biochemical of woody plants in forest soils. For example, Choi et al. ([12]) demonstrated that the BC amendment to soil significantly increased photosynthetic activity in Pinus densiflora. Omil et al. ([34]) reported the positive effects of BC and mixed wood ash on growth in Pinus radiata. Tree seedlings growth responded positively under charcoal amended soil with low phosphorus ([37]). Zahedifar & Najafian ([53]) noticed the positive effect of BC on height, leaf area, and nutrient uptake (N, P, and K) of Ocimum basilicum L. grown on calcareous soil. Bu et al. ([8]) suggested that adding 1-2% of BC greatly increased the germination of seeds and the seedling development of Robinia pseudoacacia L. Conversely, the BC amendment to soil gave opposing results on Prunella vulgaris photosynthesis ([45]), while had no effect on the growth of Douglas-fir seedlings ([39]), thus demonstrating that plant responses to the incorporation of BC into the soil are highly inconsistent.
Most of the above BC studies focused on the analysis of biomass production. However, to understand how plant growth can be affected by BC, further parameters related to plant physiological status need to be explored. In view of this, the current study aims to assess the efficacy of physiological parameters as an indicator of the impact of BC amended soil on F. hodginsii seedling development.
The main goals of this study were to investigate the influence of varying levels (0, 5, 20, and 80 g Kg-1 of soil) of BH amendment to red soil on: (i) photosynthetic pigments, biochemicals, and gas exchange attributes of F. hodginsii seedlings in four different seasons; (ii) biomass and soil physicochemical properties at final harvest under greenhouse conditions for one year. We hypothesized an overall positive effect of BH addition to soil fertility and key physiological features of F. hodginsii seedlings.
Material and methods
Description of the study area and design
The present work was carried out at the Bamboo Institute of Fujian Agriculture and Forestry University, Fuzhou, China. Hardwood biochar (BH) was produced from Pinus massoniana and Cunninghamia lanceolata at 420 °C, purchased from Nanjing Qinfeng Straw Technology Co. Ltd., China. The physicochemical properties of the soil and BC were both characterized prior to its application (Tab. S1 in Supplementary material). In March 2017, one-year-old seedlings of F. hodginsii were subjected to four different treatments characterized by increasing BC addition to soil, namely: (i) B0 (no biochar/control); (ii) BH5 (5 g kg-1 of soil); (iii) BH20 (20 g kg-1 of soil); and BH80 (80 g kg-1 of soil). Seedlings were collected from forest nursery (Anxi County, Fujian, China). A total of 32 seedlings were grown in PVC pots (height: 18 cm; top circumference: 62 cm; bottom circumference: 52 cm; soil weight: 5 kg per pot) under four different BC levels in a completely randomized design. In order to produce healthy seedlings, all replicates were placed in a glasshouse with natural light and full irrigation. In addition, after 15 days from the establishment, 10 grams per pot of NPK fertilizer (15:15:15) was applied as recommended by the Anxi Forest Nursery. After three months (June 2017), physiological attributes (gas exchange) were recorded and leaf tissues were analyzed for photosynthetic pigments and biochemical composition. Similarly, these parameters were systematically recorded at three-month intervals on September 2017, December 2017, and March 2018. Besides, soil physicochemical properties, plant morphological attributes (height and diameter), and total biomass were determined at the final harvest.
Characterization of biochar and soil physicochemical properties
Before the experiment, both soil and BC were assessed for basic physicochemical properties (see Tab. S2 in Supplementary material). In addition, soil samples were taken from each replicate at the final harvest to assess the physicochemical properties of the soil, including pH, total nitrogen (N), total carbon (C), total and available phosphorus (P), and available potassium (K). The soil samples were air-dried and then passed through a 2/0.149 mm sieve and the fractions were analyzed for selected physicochemical properties. The soil pH (1:2.5 soil/water suspensions) was recorded using a portable pH meter (INESA Science Instrument Co., Ltd., Shanghai, China) as described by Tarin et al. ([44]). Total N and soil TC were determined through the Elemental analyzer (Thermo Scientific™, Waltham, MA, USA). Total and available P were measured using the alkali fusion-Mo-Sb anti-colorimetry method on the spectrophotometer (BioTek, Epoch2, Winooski, VT, USA) as described by [49]. The extraction of available potassium (AK) was carried out using a flame photometer (FP640®, AOPU Analytical Instruments, Shanghai, China) in ammonium acetate solution according to Pansu & Gautheyrou ([36]).
Light-response curves and gas exchange
During each season (June 2017 to March 2018), upper-middle healthy leaves from each replicate were selected for recording gas exchange attributes and constructing light-response curves of Pn. All the measurements were conducted between 09:00 and 11:30 a.m. Before each measurement, photo-induction of 30 mins at 800 µmol m-2 s-1 was ensured as suggested by Chen et al. ([11]). The photosynthetic photon flux density (PPFD) at 15 different concentrations (2000, 1600, 1200, 1000, 800, 600, 400, 200, 100, 80, 60, 40, 20, 10 and 0 µmol m-2 s-1) and single CO2 concentration (400 µmol mol-1) were fixed in a portable Pn system (LI-6400XT®, LI-COR Biosciences, Lincoln, NE, USA). We used a modified model of rectangular hyperbola with highly fitted data (approx. R2 > 0.99) for the light-response curves and photosynthetic parameters as described by Chen et al. ([11]). The detailed information and equations of the modified model of rectangular hyperbola are presented in Appendix 2 (Supplementary material).
Photosynthetic pigment contents and leaf biochemical traits
In each season, healthy leaves from seedlings were collected, washed, and dried on filter paper to assess the concentrations of biochemicals. The photosynthetic pigments were determined immediately after the collection of the samples by retaining leaves in 80% acetone for 48-72 h in darkness at 4 °C until their color changed completely to white. Further absorbance of extractions for chlorophylls (a, b), total, and carotenoids were determined at 663, 645, and 470 nm, respectively, and calculated by using the equations of Lichtenthaler ([25]) (Appendix 1 in Supplementary material).
The total soluble protein (TSP) at absor-bance 595 nm and amino acids at 650 nm was determined following the procedures of Bradford ([7]). The concentration of ascorbic acid (absorbance at 536 nm) was determined as described by Keller & Schwager ([23]). Soluble sugars (absorbance at 620 nm) were estimated with the Anthrone reagent ([10]). The equations and formulas used for calculations are described in Appendix 1 (Supplementary material).
Determination of morphology and biomass
Before the final harvest, the height and diameter of seedlings were determined using a diameter tape and a vernier caliper, respectively. To determine biomass, all the seedlings were uprooted, washed with care, dried on filter paper, and fresh weight was examined. These seedlings were then tagged and oven-dried at 80 °C for 48 h to record dry weight.
Statistical analysis
All the data are expressed as means and standard errors. Analysis of variance (ANOVA) was performed using SPSS® ver. 17.0 (SPSS Inc., Chicago, IL, USA) to determine the effect of soil BH treatments, and Tukey-HSD test was used to identify significant differences between mean treatments. The softwares Origin® v. 8.5 (OriginLab Corp., Northampton, MS, USA) and Prism v. 8.0.1 (GraphPad, San Diego, CA, USA) were used to fit the light response curves and produce graphs, respectively.
Results
Influence of BC on physicochemical properties of soil
The increase in BC application rates resulted in a significant (p < 0.05) rise in soil pH under BH20 and BH80 treatments compared with B0 and BH5. Total nitrogen (TN), total carbon (TC), and organic matter (OM) content increased significantly (p < 0.05) in all BH amended soils over B0 (Fig. 1). Moreover, among each BH levels, there was no significant difference (p < 0.05) for total phosphorus (TP) and available phosphorus (AP) contents. However, compared to B0, TP, AP, and AK contents increased significantly (p < 0.05) under BH20 and BH80 amendments.
Fig. 1 - Physicochemical properties of soil treated with different levels of hardwood biochar (BH). (B0): control soil without hardwood biochar amendment; (BH5): hardwood biochar-amended soil at 5 g kg-1; (BH20): hardwood biochar-amended soil at 20 g kg-1; (BH80): hardwood biochar-amended soil at 80 g kg-1, respectively. (TN): total nitrogen; (TP): total phosphorous; (AP): available phosphorous; (AK): available potassium; (OM): organic matter; (TC): total carbon. Significant differences (p < 0.05) among various treatments are shown by various letters. Vertical bars represent the standard error of the mean (n=4).
Influence of BC on photosynthetic pigments and photosynthesis
Overall, seedlings treated with BH80 showed a significant impact on total chlorophyll (TC) concentrations throughout the year as compared to B0 (Fig. 2). At first season (June 2017), all BH amended seedling’s TC concentrations were significantly (p < 0.05) higher than B0, and BH80 treated seedlings effectively increased their TC concentrations, with an increase of 34% compared to B0 (Fig. 2a). During the second season (September 2017), except for BH80, none of the other BC levels significantly affected TC concentrations (Fig. 2b), and an increase of 9% was observed in TC concentrations for BH80 treated seedlings relative to B0. In the third season (December 2017), there was a reduction in photosynthetic pigment concentrations for all seedlings, but the seedlings in BH20 and BH80 treatments showed significant influence (p < 0.05) compared to B0, and there was an increase of 3% in TC concentrations under BH80 amended seedlings (Fig. 2c). Likewise, in the fourth season (March 2018), the highest dose of BC (BH80) influenced the TC concentration significantly (p < 0.05) compared to other levels and an increase of 13% was observed for BH80 seedlings as compared to B0 (Fig. 2d). In BC amended seedlings, carotenoids concentration was significantly impacted (p < 0.05) in the first season. Whereas in the third and fourth seasons, none of the BC rates influenced the concentration of carotenoids compared with B0.
Fig. 2 - Photosynthetic pigments concentrations in four seasons under various level of hardwood biochar. TC and CARO indicate total chlorophyll and carotenoid, respectively. (B0): control soil without hardwood biochar amendment; (BH5): hardwood biochar-amended soil at 5 g kg-1; (BH20): hardwood biochar-amended soil at 20 g kg-1; (BH80): hardwood biochar-amended soil at 80 g kg-1, respectively. (a): 1st season (June 2017); (b): 2nd season (September 2017); (c): 3rd season (December 2017); (d): 4th season (March 2018). Different letters indicate significant differences (p < 0.05) among treatments. Vertical bars represent the standard error of the mean (n=4).
The values of photosynthetic parameters such as initial slope (α), maximum photosynthetic rate (Pn-max), light saturation point (LSP), light compensation point (LCP), rate of dark respiration (Rd), and adjusted R2 are depicted in Tab. 1. Photosynthetic rate (Pn-max) increased with a rise in gradient (PPFD), as shown in Fig. 3and reached LSP, while with further increase in gradient, Pn started to decrease due to photo-inhibition. Based on the results of the modified model of rectangular hyperbola, Pn-max, and LSP both were decreased while LCP was increased (Tab. 1). In the first season, BC amended seedlings showed significantly (p < 0.05) higher Pn-max values (5.42, 6.32, and 6.72 CO2 µmol m-2 s-1 for BH5, BH20, and BH80, respectively) compared to B0 (3.48 CO2 µmol m-2 s-1). During the second season, seedlings under BH20 and BH80, the Pn-max values (6.94 and 7.24 CO2 µmol m-2 s-1, respectively) were significantly higher compared to B0 and BH5 (Tab. 1). A similar trend was noticed for all BC amended seedlings in the third season, when a reduction in the Pn-max range for all amended seedlings compared to the previous season was recorded. Nevertheless, BC altered seedlings, especially BH20 and BH80, showed significantly higher Pn-max values (3.86 and 4.04 CO2 μmol m-2 s-1) compared to B0, respectively. During the fourth season, no significant differences were noticed under the BC treated and B0 seedlings for Pn-max. Additionally, conductance to H2O (Cond), intercellular CO2 concentration (CI), and transpiration rate (Trmmol) were also recorded at different PPFD, where greater Cond and Trmmol increased with Pn and decreased CI (see Fig. S1, Fig. S2, and Fig. S3 in Supplementary material).
Tab. 1 - Season-wise comparison in photosynthetic parameters of seedlings treated with varying levels of hardwood biochar (BH). (α): initial slope; (Pn-max): maximum photosynthetic rate; (LSP): light saturation point; (LCP): light compensation point; (Rd): rate of dark respiration; (R2): adjusted R2; (B0): control soil without hardwood biochar amendment; (BH5): hardwood biochar-amended soil at 5 g kg-1; (BH20): hardwood biochar-amended soil at 20 g kg-1; (BH80): hardwood biochar-amended soil at 80 g kg-1. Significant differences (p < 0.05) among treatments are indicated by different letters. Values are means ± standard errors (n=3).
Date | Treatments | α | Pn-max (CO2 µmol m-2 s-1) |
LSP (µmol m-2s-1) |
LCP (µmol m-2s-1) |
Rd (CO2 µmol m-2 s-1) |
R2 |
---|---|---|---|---|---|---|---|
Jun-17 | B0 | 0.06 ± 0.01 a | 3.48 ± 0.38 a | 745.17 ± 79.82 a | 16.01 ± 4.88 b | 0.81 ± 0.07 a | 0.98 ± 0.07 a |
BH5 | 0.10 ± 0.01 ab | 5.42 ± 0.34 b | 948.51 ± 6.86 ab | 8.57 ± 2.55 b | 0.89 ± 0.28 a | 0.98 ± 0.01 a | |
BH20 | 0.09 ± 0.01 ab | 6.32 ± 0.08 bc | 856.35 ± 22.38 ab | 3.61 ± 1.36 a | 0.35 ± 0.13 a | 0.99 ± 0.01 a | |
BH80 | 0.10 ± 0.01 b | 6.72 ± 0.34 c | 1111.30 ± 131.27 b | 5.025 ± 0.95 a | 0.53 ± 0.12 a | 0.99 ± 0.01 a | |
Sep-17 | B0 | 0.08 ± 0.03 a | 5.12 ± 0.15 a | 729.30 ± 31.42 a | 5.46 ± 1.77 a | 0.47 ± 0.16 a | 0.98 ± 0.02 a |
BH5 | 0.08 ± 0.04 a | 5.53 ± 0.49 a | 853.07 ± 88.36 ab | 4.873 ± 1.91 a | 0.42 ± 0.17 a | 0.99 ± 0.04 a | |
BH20 | 0.09 ± 0.04 a | 6.94 ± 0.32 b | 1030.46 ± 62.91 bc | 3.97 ± 1.31 a | 0.35 ± 0.12 a | 0.99 ± 0.02 a | |
BH80 | 0.08 ± 0.04 a | 7.24 ± 0.25 b | 1102.68 ± 92.00 c | 3.21 ± 0.61 a | 0.28 ± 0.04 a | 0.99 ± 0.01 a | |
Dec-17 | B0 | 0.06 ± 0.01 a | 3.01 ± 0.24 a | 733.42 ± 30.58 a | 15.05 ± 0.09 a | 0.93 ± 0.13 a | 0.98 ± 0.03 a |
BH5 | 0.07 ± 0.02 a | 3.30 ± 0.18 ab | 780.39 ± 69.98 a | 12.60 ± 0.80 a | 0.95 ± 0.03 a | 0.98 ± 0.04 a | |
BH20 | 0.08 ± 0.01 a | 3.86 ± 0.27 b | 819.21 ± 122.80 a | 12.16 ± 2.36 a | 0.92 ± 0.08 a | 0.98 ± 0.05 a | |
BH80 | 0.08 ± 0.01 a | 4.04 ± 0.19 b | 805.57 ± 129.43 a | 11.66 ± 2.46 a | 0.90 ± 0.09 a | 0.99 ± 0.01 a | |
Mar-18 | B0 | 0.08 ± 0.01 a | 3.95 ± 0.36 a | 711.93 ± 63.01 a | 11.87 ± 1.41 a | 0.94 ± 0.07 b | 0.99 ± 0.06 a |
BH5 | 0.06 ± 0.01 a | 4.32 ± 0.069 a | 752.32 ± 65.55 a | 12.25 ± 0.76 a | 0.85 ± 0.02 b | 0.99 ± 0.01 a | |
BH20 | 0.08 ± 0.01 a | 4.37 ± 0.13 a | 809.25 ± 108.94 a | 7.34 ± 3.05 a | 0.56 ± 0.17 ab | 0.99 ± 0.01 a | |
BH80 | 0.08 ± 0.01 a | 4.43 ± 0.37 a | 975.94 ± 74.84 a | 6.16 ± 1.81 a | 0.51 ± 0.15 a | 0.99 ± 0.02 a |
Fig. 3 - Response of net photosynthetic rate at different photosynthetic photon flux density levels (PPFD) under different BH levels. (B0): control soil without hardwood biochar amendment; (BH5): hardwood biochar-amended soil at 5 g kg-1; (BH20): hardwood biochar-amended soil at 20 g kg-1; (BH80): hardwood biochar-amended soil at 80 g kg-1, respectively. (a): 1st season (June 2017); (b): 2nd season (September 2017); (c): 3rd season (December 2017); (d): 4th season (March 2018). Vertical bars represent the standard error of the mean (n=3).
Influence of BC on biochemical attributes
The effect of BC as soil amendment on the biochemical attributes of F. hodginsii seedlings is reported in Tab. 2. Seedlings showed significant differences (p < 0.05) for amino acid under BH20 and BH80 amendments in the first two consecutive seasons, compared to B0. Nevertheless, none of the BC doses influenced amino acid concentrations during the final two seasons (Tab. 2). Ascorbic acid concentrations decreased significantly (p < 0.05) by increasing the level of BC. During the whole study period, BH20 and BH80 treated seedlings showed significant differences over B0 (Tab. 2). Likewise, compared with B0, concentrations of soluble sugar under BH20 and BH80 amended seedlings were significantly higher (p < 0.05) in the first and third seasons (June and December 2017). Total soluble protein (TSP) concentrations were influenced significantly (p < 0.05) for all BH amended seedlings in the second season. However, during the last two seasons, the addition of BC did not affect TSP concentrations in comparison with B0 (Tab. 2).
Tab. 2 - Season-wise biochemical attributes in seedlings treated with different levels of biochar (BC). (B0): control soil without hardwood biochar amendment; (BH5): hardwood biochar-amended soil at 5 g kg-1; (BH20): hardwood biochar-amended soil at 20 g kg-1; (BH80): hardwood biochar-amended soil at 80 g kg-1. Significant differences (p < 0.05) among treatments are shown by different letters. Values are means ± standard errors (n=4).
Date | Treatments | Amino acid (µg g-1) |
Ascorbic acid (µg g-1) |
Soluble sugar (µg g-1) |
Total Soluble Protein (g L-1) |
---|---|---|---|---|---|
Jun-17 | B0 | 4.04 ± 0.38 a | 19.58 ± 1.22 b | 11040.97 ± 495.12 a | 0.23 ± 0.02 ab |
BH5 | 5.17 ± 0.26 ab | 19.01 ± 0.90 b | 12458.47 ± 134.26 b | 0.20 ± 0.03 a | |
BH20 | 7.01 ± 0.26 b | 14.57 ± 2.28 b | 12558.13 ± 76.72 b | 0.28 ± 0.04 b | |
BH80 | 8.76 ± 1.00 b | 9.52 ± 1.06 a | 15049.83 ± 191.81 c | 0.40 ± 0.02 c | |
Sep-17 | B0 | 1.00 ± 0.29 a | 186.16 ± 17.36 b | 24316.80 ± 3953.43 a | 0.42 ± 0.01 a |
BH5 | 2.29 ± 0.15 b | 124.36 ± 20.30 a | 35241.51 ± 3130.46 a | 0.64 ± 0.01 b | |
BH20 | 2.70 ± 0.23 b | 113.50 ± 4.45 a | 35561.35 ± 6541.47 a | 0.69 ± 0.02 b | |
BH80 | 2.96 ± 0.28 b | 130.31 ± 13.90 a | 34843.34 ± 2321.57 a | 0.83 ± 0.01 c | |
Dec-17 | B0 | 1.44 ± 0.35 a | 76.84 ± 6.55 c | 34623.27 ± 392.65 a | 0.16 ± 0.06 a |
BH5 | 2.83 ± 0.52 a | 41.31 ± 0.99 b | 36870.68 ± 1845.46 a | 0.16 ± 0.05 a | |
BH20 | 2.89 ± 0.67 a | 7.93 ± 0.95 a | 47086.20 ± 1037.59 b | 0.17 ± 0.04 a | |
BH80 | 2.64 ± 0.52 a | 6.17 ± 0.24 a | 51801.72 ± 2485.96 b | 0.17 ± 0.03 a | |
Mar-18 | B0 | 1.32 ± 0.47 a | 37.74 ± 0.50 c | 36000.00 ± 1076.49 a | 0.17 ± 0.04 a |
BH5 | 1.32 ± 0.47 a | 17.02 ± 1.12 b | 37028.45 ± 486.15 a | 0.17 ± 0.03 a | |
BH20 | 1.51 ± 0.38 a | 5.08 ± 0.14 a | 37045.97 ± 521.88 a | 0.18 ± 0.04 a | |
BH80 | 2.02 ± 0.45 a | 4.61 ± 0.16 a | 37568.96 ± 180.55 a | 0.20 ± 0.02 a |
Influence of BC on plant morphological features and plant biomass
In terms of morphological attributes, seedlings of F. hodginsii established under BH20 treatment significantly (p < 0.05) influenced the plant height compared to B0. However, no significant differences were observed for seedling’s diameter, under varying levels of the BC (Fig. 4a). Compared to B0, the total biomass of seedlings treated with BH20 (21%) and BH80 (25%) was significantly higher. Similarly, seedlings treated with BH80, BH20, and BH5 enhanced the plant dry weight (5.38%, 4.79%, and 0.154% respectively) over B0 with no significant differences (Fig. 4b).
Fig. 4 - (a) Height and diameter (b) biomass and dry weight of seedlings treated with different levels of hardwood biochar. (B0): control soil without hardwood biochar amendment; (BH5): hardwood biochar-amended soil at 5 g kg-1; (BH20): hardwood biochar-amended soil at 20 g kg-1; (BH80): hardwood biochar-amended soil at 80 g kg-1, respectively. A significant difference (p < 0.05) among treatments is indicated by different letters. Vertical bars represent the standard error of the mean (n=4).
Discussion
The amendment of BC has been suggested to enhance soil nutrients dynamics, quality, and plant productivity ([16]). In this study, under BC addition, soil physicochemical properties were improved after one year in the glasshouse pot experiment. BC incorporation influenced the soil pH significantly (p < 0.05) under BH20 and BH80 amendments. The great liming effect of BC has also been reported in previous studies on acidic soils. In this study, the rise in soil pH improved the availability of nutrients, particularly P and K, which is consistent with previous findings ([8]). BC amendment significantly affected the TN and TC of soil by increasing the level, which is attributed to the presence of N and C contents in the BC feedstock. Biederman et al. ([6]) stated that increased TN retention in BC amended soil is due to nitrates recycling because of the higher availability of C. Soil P and K availability were significantly improved under higher doses of BC (BH20 and BH80), due to the greater concentrations of available P and K in the BC being released into acidic soils (Fig. 1). Prendergast-Miller et al. ([38]) showed that BC particles not only hold the soil N in the form of nitrate but also supply P to the soil and plant, as observed in this study. Moreover, Agegnehu et al. ([2]) stated that ash contents in BC alleviate the soil pH which improves the availability of the nutrients (K, Ca, and Mg).
Biochemical leaf traits and photosynthetic pigments are the key indicators of plant metabolism that are mainly influenced by the availability of resources (nutrients) and environmental conditions. BC increases the absorption of nutrients by enhancing the physiological state of the entire plant ([4]). Overall, the seedlings treated with BC showed higher concentration of amino acids in the first season, whereas TSP concentrations were greater in the second season. One possible reason for this rise in amino acid concentration was owing to the addition of NPK fertilizer applied to all seedlings in the first season. Indeed, previous studies on BCs proved that BC efficacy can be improved if combined with fertilizers. Greater concentrations of TSP at the second season under BC amended seedlings are attributed to allocations of nutrients. The highest photosynthetic pigment concentrations (Fig. 2b) under BC treated seedlings indicates the N status of plants compared to B0, as confirmed in previous studies ([30]). Besides, the growing season of this conifer is July-September where plants produce higher concentrations of biochemicals. Similar to our findings, Gezelius & Hallen ([18]) revealed that the concentration of needle TSP in Scots pine started to decline in April and then increase in July. In addition, in the current research, seedlings were able to grow under normal conditions. However, there were plenty of differences in air temperature in four seasons (Fig. S4 in Supplementary material). Zemanova et al. ([54]) stated that both amino acids and TSP are environmentally dependent factors as their concentrations vary with fluctuation in temperature, and the plant produces high concentrations of amino acids under favorable conditions.
The variations in concentration of soluble sugars and ascorbic acids were also due to temperature fluctuations in various seasons. Ascorbic acid is an antioxidant molecule which plays a key role in the mechanisms of defense against environmental stress due to temperature variations (Fig. S4 in Supplementary material). The most effective function of ascorbic acid is to protect lipids and proteins against salinity or oxidative adverse reactions caused by drought ([32]). Plants exposed to environmental stress have the tendency to accumulate osmotic substances in cells ([22]), such as proline, soluble sugar, ascorbic acid, and various osmoprotectants. Besides, the application of BC to the soil can also substantially alters the soil structure and field capability and exhibit the antioxidant activity by decreasing the membrane permeability and contents of ascorbic acid. BC incorporation at higher levels reduced the concentrations of ascorbic acid, which is in accordance with previous studies ([48]).
Based on biochemical and molecular research, soluble sugars play a key role in controlling plant metabolism. In our experiment, BC amended seedlings accumulated more soluble sugars at first and third season compared to B0. Increased concentrations of soluble sugars enable plants to cope with cold stress in winters ([41]). Similar to our findings, Yuanyuan et al. ([52]) confirmed that soluble sugars concentrations under low-temperature (winters) increase (Dec-17) and decrease (Jun-17) in summers.
Several environmental factors affect Pn, including light, temperature, air CO2 concentration, water supply, the vapor pressure differential between leaves and air, soil fertility, salinity, toxins, applied chemicals, insects, diseases, and various interactions between them. Among them, light and temperature are the most influential factors involved in species survival, development, and reproduction. Their responses usually cause physiological changes, which are decisive for assimilating CO2 and optimizing gas exchange ([9]). In the current study, variations in Pn and photosynthetic pigments in different seasons might be due to variation in temperature and daylight ([39]). For instance, all the BC treated seedlings enhanced their photosynthetic pigments and Pn-max during the first season (Fig. 2, Tab. 1). The addition of BC to soil has been suggested as a way to increase soil water holding capacity, nutrient retention, and soil aeration and respiration ([40]). Higher concentrations of photosynthetic pigment under BC treated seedlings, particularly in the first season, was due to the availability of essential nutrients. As stated before, NPK fertilizer was applied to all seedlings and the combined application of BC with fertilizer improved the concentration of photosynthetic pigments and thus Pn-max. Chen et al. ([11]) demonstrated that Pn is highly dependent on the concentration of photosynthetic pigments. Another possible reason for the improved Pn in BC amended seedlings might be the presence of available P. The P availability through BC is likely to improve the Pn in red soil, owing to its vital role in the regulation of electron transport ([51]).
The above mentioned beneficial effects of soil BC amendments together lead to a significant increase in plant physiological and biochemical efficiency. The use of BC in vineyards enhanced the soil water content and then improved the plant supply of water and Pn activity in the leaves of Vitis vinifera L. ([5]). Plant water status plays an important role in plant growth and photosynthetic efficiency. Our research confirmed the findings that the addition of BC to soil increased the availability of water, which is responsible for increasing Pn and Cond. A similar impact of BC on the availability of soil water has also been determined by previous studies ([1], [21]). However, at the final harvest, seedlings treated with BH80 showed a noticeable influence on photosynthetic pigments (TC) but no effect on Pn-max over B0.
During the third and fourth seasons, suppression in Pn-max in plants was caused by the combined effects of daylight and temperature (Fig. S4 in Supplementary material). As reported in previous studies, low temperature inhibits Pn by reducing the activity of Calvin cycle enzymes ([50]), whereas the absorption of light energy beyond that which could be used in low-temperature Pn induces photo-inhibition of Photosystem II (PSII - [43]). Similar to our findings, various studies have seen fluctuations in the Pn capacity of other conifers (spruce and pine species) and demonstrated that changes in Pn capability are closely related to seasonal variations in air temperature ([26], [50]). Moreover, the activity of Ribulose-1.5-biphosphate carboxylase (Rubisco), the enzyme responsible for the fixation of CO2 in chloroplasts, varies with the season, with significantly higher activity during summer than in winter ([31]). A strong correlation has been reported in several cases between Rubisco activity and TSP concentration in leaves ([50]). In addition, chlorophyll concentrations have been shown to decrease in winter, under low temperature and short-day conditions ([11], [44]). Therefore, the observed decrease in Pn-max during the third season may also be associated with a decrease in chlorophyll concentration.
The process of acclimatization to high-irradiance environments is very complex, involving both water and nutrient availability ([15]). The physiological activity of F. hodginsii plants exposed to various PPFD in this study indicates that their Pn apparatus is more effective when subjected to medium light intensity conditions, between 700 and 1100 μmol m-2 s-1 (Tab. 1). The rate of Pn also increases rapidly with an increase in incidental PPFD before the plateau is reached. This initial linear relationship reflects the photochemical efficiency of plants, i.e., the ability to absorb light (LUE). The Pn rate increases linearly with increased irradiance since CO2 fixation and electron transport processes are proportional to the photons captured ([42]). Therefore, these findings suggest that, during F. hodginsii early growth period, intermediate light conditions are more suitable for this species to achieve higher rates of C assimilation and accumulation of biomass.
In the short term (days to weeks) the environmental conditions affect Pn by controlling stomatal conductance and photosynthetic ability of mesophylls. We found great differences in Cond and Trmmol among BC applications in diverse seasons except for Ci (Fig. S1, Fig. S2, Fig. S3 in Supplementary material). The increase in Ci indicates a reduction in the activity of CO2 assimilation, with limited carboxylation efficiency ([17]). It seems appropriate to refer to Ci as an effective concentration of CO2 controlling stomata ([29]). However, in the current study, all the seedlings received the same amount of water. A sufficient supply of water is an advantage in terms of limited response to Ci, as this restricts the reluctance of the stomata to limit the supply of CO2 to Pn. However, under minimal water supply, the priority of the plant shifts from maximizing assimilation to limiting transpiration while retaining as much assimilation as possible ([14]).
Numerous BC studies have demonstrated beneficial effects on biomass increase ([13], [8]). Likewise, we also found a positive influence of BC on the biomass of F. hodginsii particularly under BH20 and BH80 amendments. BC supplements to soil provide greater water availability, increase nutrient absorption, provide better conditions for the synthesis of organic solutes, and minimize oxidative stress through high water performance ([35]). The improved photosynthetic performance of seedlings resulted in a greater capacity to accumulate biomass. This improvement in biomass was collectively associated with increased physiological parameters particularly Pn and the availability of nutrients (P and K) because of the rise in the soil pH. Altogether, these improvements in soil quality lead to a major enhancement of the physiological status of seedlings and thus to their biomass production. Nonetheless, abiotic factors (air temperature, light, water, CO2, and nutrients) and physiologically mediated factors (Cond and Trmmol) may have a significant effect on this process ([15]). Our findings are in line with previous authors who suggested that BC containing high amount of of available P, can have a positive effect on tree seedling growth ([37]). Makoto et al. ([28]) also demonstrated that BC significantly contributed to the amelioration of P availability and water availability for pine seedlings.
Conclusions
The findings of the present research suggest that BH amendments can be recommended as soil ameliorants into acidic soil. The addition of BH20 and BH80 to red soil had a significantly positive impact on the biomass (21% and 25%, respectively) of F. hodginsii. BC supplements to soil provided increased nutrients concentrations, better conditions for the synthesis of photosynthetic pigments, and gas exchange attributes. Altogether such improvement of soil quality contributed to a significant enhancement of the physiological status of plants, thereby increasing the biomass of F. Hodginsii seedlings. Besides, it could open up the possibility of sequestering CO2 and generating higher wood productivity and high-quality biomass on degraded soils in China. As BC effects may vary across different soil types and plant species, we recommend to accurately consider soil pH and N requirements of species selected for maximizing the achievement of BC-based restoration projects. In compliance with the present study, further research is needed on older trees with much slower growth considering the interaction between soil microbial community and soil physiochemical properties to confirm the outcomes of these pot trials.
Acknowledgments
This work was supported by Science and Technology Major Projects of Fujian Province [2018NZ0001-1] and Fujian Seedling Science and Technology Research Project, P. R. China.
References
CrossRef | Gscholar
CrossRef | Gscholar
CrossRef | Gscholar
Gscholar
Authors’ Info
Authors’ Affiliation
Yueqin Cai 0000-0002-0684-9342
Lingyan Chen
Tianyou He
Yushan Zheng 0000-0001-9545-0984
College of Arts & College of Landscape Architecture, Fujian Agriculture and Forestry University, Fuzhou 350002 (P.R. China)
Jundong Rong 0000-0002-8733-8500
Yushan Zheng 0000-0001-9545-0984
College of Forestry, Fujian Agriculture and Forestry University, Fuzhou, Fujian 350002 (P.R. China)
College of Crop Science, Fujian Agriculture and Forestry University, Fuzhou, Fujian 350002 (P.R. China)
Corresponding author
Paper Info
Citation
Tarin Muhammad Waqqas K, Fan L, Cai Y, Tayyab M, Chen L, He T, Rong J, Zheng Y (2020). Biochar amendment regulated growth, physiological, and biochemical responses of conifer in red soil. iForest 13: 490-498. - doi: 10.3832/ifor3416-013
Academic Editor
Daniela Baldantoni
Paper history
Received: Mar 31, 2020
Accepted: Aug 15, 2020
First online: Nov 01, 2020
Publication Date: Dec 31, 2020
Publication Time: 2.60 months
Copyright Information
© SISEF - The Italian Society of Silviculture and Forest Ecology 2020
Open Access
This article is distributed under the terms of the Creative Commons Attribution-Non Commercial 4.0 International (https://creativecommons.org/licenses/by-nc/4.0/), which permits unrestricted use, distribution, and reproduction in any medium, provided you give appropriate credit to the original author(s) and the source, provide a link to the Creative Commons license, and indicate if changes were made.
Web Metrics
Breakdown by View Type
Article Usage
Total Article Views: 34491
(from publication date up to now)
Breakdown by View Type
HTML Page Views: 30490
Abstract Page Views: 1811
PDF Downloads: 1730
Citation/Reference Downloads: 1
XML Downloads: 459
Web Metrics
Days since publication: 1702
Overall contacts: 34491
Avg. contacts per week: 141.85
Article Citations
Article citations are based on data periodically collected from the Clarivate Web of Science web site
(last update: Mar 2025)
Total number of cites (since 2020): 9
Average cites per year: 1.50
Publication Metrics
by Dimensions ©
Articles citing this article
List of the papers citing this article based on CrossRef Cited-by.
Related Contents
iForest Similar Articles
Research Articles
Soil fauna communities and microbial activities response to litter and soil properties under degraded and restored forests of Hyrcania
vol. 14, pp. 490-498 (online: 11 November 2021)
Research Articles
Effect of restoration methods on natural regeneration in the Brazilian Atlantic Forest
vol. 18, pp. 23-29 (online: 15 February 2025)
Research Articles
Can the dynamics of forest restoration reduce landscape fragmentation in the Atlantic forest?
vol. 18, pp. 61-68 (online: 04 April 2025)
Research Articles
Effect of plant species on P cycle-related microorganisms associated with litter decomposition and P soil availability: implications for agroforestry management
vol. 9, pp. 294-302 (online: 05 October 2015)
Research Articles
Soil stoichiometry modulates effects of shrub encroachment on soil carbon concentration and stock in a subalpine grassland
vol. 13, pp. 65-72 (online: 07 February 2020)
Research Articles
Photosynthesis of three evergreen broad-leaved tree species, Castanopsis sieboldii, Quercus glauca, and Q. myrsinaefolia, under elevated ozone
vol. 11, pp. 360-366 (online: 04 May 2018)
Research Articles
Wood-soil interactions in soil bioengineering slope stabilization works
vol. 2, pp. 187-191 (online: 15 October 2009)
Research Articles
Soil respiration along an altitudinal gradient in a subalpine secondary forest in China
vol. 8, pp. 526-532 (online: 01 December 2014)
Research Articles
Stem profile of red oaks in a bottomland hardwood restoration plantation forest in the Arkansas Delta (USA)
vol. 15, pp. 179-186 (online: 19 May 2022)
Research Articles
Impact of deforestation on the soil physical and chemical attributes, and humic fraction of organic matter in dry environments in Brazil
vol. 15, pp. 465-475 (online: 18 November 2022)
iForest Database Search
Search By Author
Search By Keyword
Google Scholar Search
Citing Articles
Search By Author
Search By Keywords
PubMed Search
Search By Author
Search By Keyword