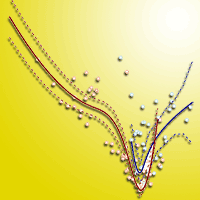
Oak often needs to be promoted in mixed beech-oak stands - the structural processes behind competition and silvicultural management in mixed stands of European beech and sessile oak
iForest - Biogeosciences and Forestry, Volume 13, Issue 2, Pages 80-88 (2020)
doi: https://doi.org/10.3832/ifor3172-013
Published: Mar 01, 2020 - Copyright © 2020 SISEF
Research Articles
Abstract
Forest ecosystems nowadays provide multiple ecosystem goods and services at a time and throughout all development phases. Species mixing is considered an effective measure to gain benefits beyond purely additive effects. However, the complex structural processes behind interspecific competition and temporal and spatial facilitative effects through mixing are still far from being understood and predictable. In particular the mixture of European beech (Fagus sylvatica L.) and sessile oak (Quercus petraea [Matt.] Liebl.) is gaining even more importance due to the fact that forests from these species are considered more tolerant to climatic effects and are expected to expand their natural range to the north due to global warming. The 30 long-term experimental plots analysed in this study reveal the structural processes in mixed beech-oak stands based on data at the tree and stand level. Using spatial and non-spatial structural indices, we can show an increasing dominance of beech over oak in unmanaged stands and the effectiveness of thinning operations to support oak. Those processes are representative for other light-demanding tree species in mixtures with shade-tolerant species. Improving the knowledge on the structural processes in mixed-species stands is particularly relevant when trying to modify forest structure in order to adapt forest management to shifting environmental conditions and the increasing demand for ecosystem services.
Keywords
Ecosystem Services and Functions, Facilitation and Competition, Mixing Regulation, Silvicultural Prescriptions, Spatial Distribution, Stand Development, Structural Complexity, Thinning
Introduction
The European beech (Fagus Sylvatica L.) and the sessile oak (Quercus petraea [Matt.] Liebl.) are two of the most prevalent broad-leaved tree species in Central Europe and they occur mostly in Central Europe, ranging from the north of Spain up to southern Scandinavia ([3]). As soon as the canopy is closed in mixed stands of oak and beech, there is only a low amount of light penetration ([10]). The shade-tolerant European beech saplings therefore seem to out-compete the light-demanding sessile oak saplings in mixed stands in all light conditions ([23]). The morphological plasticity of European beech additionally allows a restriction or even penetration of the crowns of neighbouring oak trees. On most sites, European beech also reaches much higher maximum tree heights and diameters than sessile oak, and consequently overgrows and impedes sessile oak severely. A warmer and drier climate can reduce the height growth of beech ([5]). Sessile oak, on the contrary, is expected to adapt better to a warmer and drier climate than European beech ([41]), which will therefore modify the competitive relationship between the two species.
Silvicultural management can modify the forest structure and interactions between tree species, and can foster not only wood production but also other ecosystem goods and services beyond the additional effects of mixing ([2]). However, specific silvicultural guidelines for mixed-species stands are often lacking. Experience in the field of silviculture shows that the cultivation of oak in mixed stands requires a considerate management in order to establish and maintain beneficial forest structures to support the regeneration and development of oak ([48]), e.g., through thinning. The production of high quality oak wood and the need for silvicultural intervention are therefore often in conflict with conservation interest groups, aiming at an increase in unmanaged forest area ([13]).
Despite the potential interspecific competition and the need for frequent management interventions, mixed-species stands have in many cases shown benefits compared to pure forest stands ([15]). These benefits mostly result from a functional diversity ([25]) and include a general reduction in the risk of mortality, higher stability, above-ground carbon storage ([25]), and productivity ([22]). One reason for the superior growth in beech-oak stands may be the hydraulic lift of water ([34]). European beech also shows a higher number of fine roots compared to sessile oak ([21]), which may result in a more efficient use of below-ground resources. A more efficient vertical and horizontal exploitation of space due to the different needs in light intensities may be an additional reason for overyielding.
As stand structure plays an important role in the effective and sustainable management of mixed forest stands, it may be specifically modified with the aim of supporting certain ecosystem functions. By thinning from above, for example, structural heterogeneity can be increased compared to thinning from below, which can make stands more homogeneous ([31]). The structural heterogeneity of a stand can then enhance stand productivity ([8]), diminish it ([6]) or both. The structural effect may be positive or negative, depending on the site conditions and on the development phase of the forest stand ([49]). In addition, enhanced forest biodiversity and stand structural complexity are assumed to be positively related ([50]), which in turn can positively influence stand productivity. In order to modify and benefit from those structural effects, effective silvicultural management has to consider not only species-mixing and changes in environmental conditions, but also the initial stand structures and how they are modified over time by species identity and management ([26]).
By analysing 30 long-term experimental forest plots of European beech and sessile oak in southern Germany, the aim of this study is to demonstrate the structural processes behind facilitation, competition and silvicultural management in mixed beech and oak stands over time. The experimental plots (between 0.2 and 1 hectare in size) were subjected to different intensities and types of thinning. We applied structural indices at stand and tree level that are commonly used to quantify stand structure ([29]). The effects of both thinning and natural mortality on the structural indices were compared with each other.
This study contributes to the understanding of the structural processes behind silvicultural management with regard to the competition in mixed beech-oak stands. It will improve forest management in terms of controlling species mixtures in the long term, and of adapting forests to future challenges and demands by addressing the question: how are the structure and competition of mixed European beech and sessile oak stands modified by thinning type and intensity, compared to natural mortality? In this context, we hypothesised that natural mortality and thinning have similar effects on the structure and competition in mixed European beech and sessile oak stands.
Materials and methods
Study area
For the current study, we used inventory data of long-term experimental plots dominated by sessile oak and European beech, which are all located in Bavaria, Germany, and have similar climatic conditions. The water and nutrient supply is favourable for both species. Additional species occurring in small numbers were European hornbeam (Carpinus betulus), birch (Betula pendula and Betula pubescens), lime (Tilia platyphyllos and Tilia cordata) and European Larch (Larix decidua). The experimental plots are located close to Waldbrunn, Burgsinn, Illertissen, Lohr-West, Elmstein-Nord, Rohrbrunn, Rothenbuch, Schweinfurt, and Kelheim. On the plots, the mean annual temperature ranges from 7 to 8.5 °C, the annual precipitation is between 660 and 1150 mm y-1 and elevation is between 270 and 555 m a.s.l. Several measurements of long-term experimental plots were carried out in the time between 1927 and 2016. Since this study deals with spatial analyses, which require the coordinates of all trees, we excluded the measurements before 1986, when Cartesian coordinates for the trees were first recorded. Our study plots are rectangular, ranging between 0.2 and 1 hectare in size, and were surveyed 2-4 times, with 4 to 17-year intervals between measurements. The sample plots were 27-371 years old and have been thinned with different intensities (0-45% of total basal area on plots) and types (thinning from above and thinning from below). In each survey, all living trees with diameter at breast height (d) of 5 cm or greater, reaching a height (h) of 1.3 m or more, dead and thinned trees were tallied and identified at a species level. For every fifth tree, and for dominant and rare tree species, the tree height, crown radius and crown projection area were also measured. Tab. 1 summarises the structural properties of plots and the two main study species (European beech and sessile oak) for 20 unmanaged plots, where trees died only due to natural mortality and for 28 managed plots, where - in addition to natural mortality - further trees were removed due to thinning practices.
Tab. 1 - The initial stand characteristics (mean ± standard error) of the study plots in total and for the two main species. (N): number of trees (ha-1); (Dq): quadratic mean diameter of trees at breast height (cm); (BA): basal area (m2 ha-1) ; (H): mean height of the trees (m); (CRP, CRR): mean crown projection (m2) and crown radius (m) of trees within a plot, respectively. “Managed” and “Unmanaged” refer to plots with and without thinning practices, respectively.
Plot | Species | N | D q | BA | H | CRP | CRR |
---|---|---|---|---|---|---|---|
Unmanaged | European beech | 739 ± 98 | 17.88 ± 1.57 | 12.69 ± 0.86 | 18.18 ± 1.00 | 32.89 ± 3.28 | 3.01 ± 0.15 |
Sessile oak | 449 ± 120 | 36.34 ± 3.55 | 20.61 ± 1.42 | 26.58 ± 1.16 | 36.56± 6.69 | 3.03 ± 0.28 | |
Total | 1232 ± 177 | 23.65 ± 1.90 | 34.36 ± 1.14 | 21.65 ± 1.01 | 31.92 ± 3.63 | 2.91 ± 0.18 | |
Managed | European beech | 684 ± 95 | 18.24 ± 1.40 | 12.28 ± 0.79 | 18.44 ± 0.95 | 33.71 ± 2.96 | 3.07 ± 0.14 |
Sessile oak | 414 ± 119 | 37.48 ± 3.54 | 20.37 ± 1.21 | 26.67 ± 1.16 | 38.34 ± 6.61 | 3.12 ± 0.28 | |
Total | 1144 ± 173 | 24.61 ± 1.97 | 33.38 ± 1.11 | 21.85 ± 1.00 | 33.24 ± 3.48 | 2.99 ± 0.18 |
Stand structure quantification
A set of structural indices - describing the plots according to the intensity and spatial arrangements of the trees, the size distribution, and species intermingling - were selected for further analyses (Tab. S1 in Supplementary material). Either the structural measures were simple non-spatial measures of stand properties, which are often available in almost all forest inventories, or spatial quantifications of a central tree (reference tree) related to its neighbourhood. The spatial indices account for one or four nearest neighbours of each reference tree. Depending on the algorithm of the structural indices, the calculated values were at tree, species or plot level.
Indices 1-4 in Tab. S1 are non-spatial measures of stand properties, which are simple to assess, and can be used for more complex assessments. The quadratic mean diameter at breast height of trees (Dq) was representative of the trees’ dimensions, whereas the stand basal area (BA), the number of trees per hectare (N) and the Reineke stand density index (SDI - [39]), describe stand density. SDI sets the allometric relationship between N and Dq and estimates the expected number of trees at a quadratic mean diameter of 25 cm.
Indices 5-7 (Tab. S1 in Supplementary material) explore the horizontal distribution pattern of trees within the study plots. The contagion index (Con), also known as the uniform angle index, counts for the four neighbours ([19]) whereas the aggregation index ([7]) and the Pielou index ([27]) refer to the distances between the reference trees and the random points from the first nearest tree, respectively.
We assessed the difference in stem diameters at breast height by another five indices, of which two are spatial (indices 8-9) and one is non-spatial (index 10). The diameter differentiation index (Dif - [14]) and diameter dominance index (Dom - [47]) count for the four nearest neighbours and quantify the diameter differentiation and the dominance status of a reference tree, respectively. Finally, the diameter heterogeneity of trees within a stand is evaluated by diameter variation (Dvar - [32]), where the higher Dvar indicates the higher diameter variation.
The spatial structure of species mixture was described by species mingling ([14]) and species segregation ([28]) (indices 11-12 - Tab. S1 in Supplementary material). The mingling index (Ming) quantifies the proportion of four nearest neighbours of a species other than that of the reference tree, whereas the segregation index (Seg) accounts for all pairs of reference trees and their first nearest neighbours within a stand and describes the intermingling of two species or species group.
Competition quantification
Additional to the structural indices, we quantified the spatial competition amongst the neighbouring trees applying the competition index introduced by Hegyi ([17] - eqn. 1). The intention was to examine the importance of species identity, stand density and tree dimensions in stand dynamics and structure, by using a single index. To do so, the competition load from oak (CIOak) and beech (CIBeech) neighbouring trees on each reference tree was separately quantified and summed up, to obtain a value for the competition forced by each species within a stand (eqn. 1):
To test whether a neighbouring tree j of any diameter (dj) is an active competitor for reference tree i with any diameter (di), we controlled the distance between them (rij). Based on the crown overlap method ([1]), we set a critical distance as the sum of the reference tree’s potential crown radius (Cri) and the neighbouring tree’s potential crown radius (Crj) and assumed trees i and j were actively interacting when rij< Cri+ Crj. Since the crown radius was not available for all trees within a plot, the non-linear allometric relationship between the potential crown projection (crp) and the diameter at breast height of trees with a crown measurement was employed ([30] - eqn. 2):
The potential crown projection model was derived for tree species of the Bavarian region, which made the use of the model plausible. In order to estimate the parameters a0 and a, for both species in the beech-oak mixture we used the nonlinear least squares regression and the trees with measured crowns from empirical data. For further analyses, we then used estimated crown projection area for all trees.
The edge effect correction
The immediate neighbours for trees standing near the monitoring plot borders may stand outside the plots, and there may be no information available about them. Among the different methods developed for avoiding the biased statistical estimations due to the edge effect, a combination of border method or buffer zone ([40]) and reflection method ([38]) was applied. For indices counting the first nearest neighbour, a buffer zone was set to exclude the reference trees, where their distance to the plot borders were shorter than their distance to their first nearest neighbours ([32]). However, those trees could serve as the nearest neighbours for other reference trees. This method ensures the removal of all border errors for the mentioned indices quantifications. The reflection method results in periodic neighbourhood situations that do not naturally exist, when applied to rectangular inventory plots ([9]). However, in order to avoid losing many reference trees, as might happen when using the border method, we used the reflection method when quantifying the indices accounting for more than one nearest neighbour.
The status of natural mortality and thinning throughout stand development
In a preliminary analysis, we checked the height development of sessile oak and European beech reference (central) trees both in managed and unmanaged stands. Since the heights of all reference trees were not recorded, individual tree heights were estimated by the uniform height curve system that was first created for the European beech and then parametrised for other species ([12]) in Bavaria, Germany (eqn. 3):
where h is the individual tree height, estimated as a function of a tree’s diameter at breast height, d. We derived the parameters b0, b1 and b2, utilizing the trees with measured height on the plots.
The effect of natural mortality and thinning on stand structure
In order to examine the changes in stand structure due to natural mortality and thinning practices, we computed the structural and competition indices for three phases. This was performed for all existing trees, in managed and unmanaged plots, prior to any natural mortality and thinning practices (phase0); after drop-outs due to natural mortality in managed and unmanaged plots (phasemor); and for the remaining trees after natural mortality and thinning practices, in managed plots (phasethin). We calculated the differences in the indices presented in Tab. S1 (Supplementary material), for the three aforementioned phases, as Δmor (phasemor - phase0) and Δthin (phasethin - phasemor). In the next step, we quantified the mortality rate and thinning intensity as the ratio of the removed basal area of plots (BAremove, m2 ha-1) to the total basal area of plots (BA, m2 ha-1), due to natural mortality and thinning, respectively (eqn. 4). We also defined the mortality and thinning mode by the relative diameter of the remaining trees (Dq(remain), cm) following the natural mortality and thinning, respectively, to the quadratic mean diameter of the plots (Dq, cm) prior to mortality or thinning (eqn. 5). Eventually, we assessed the effect of mortality and thinning as follows (eqn. 4, eqn. 5)):
We quantified the values of intensity and mode both for mortality (between phase0 and phasemor) and thinning practices (between phasemor and phasethin). In this context, the intensity can take values between 0 and 100% depending on the removed basal area (m2 ha-1). Mode values equal to 1 show that mortality and thinning were equally distributed among trees of any diameter. Mode values smaller than 1 refer to the mortality of larger than average diameter trees and thinning from above. Mode values larger than 1 refer to the mortality of smaller than average diameter trees and thinning from below.
The statistical analyses
In order to check the effect of natural mortality and thinning practices on structural indices, we used the generalised additive mixed effects model (GAMM). The GAMM can appropriately address the unequal number of reference trees on the different inventory plots, and other unknown differences between the plots and potential correlations of residuals within the plots ([11] - eqn. 6):
where y is a function of x independent variables, with unknown and possibly non-linear effects, b represents a vector of random effects, ε is the remaining errors and a is the model’s intercept to be estimated, and f1, …, fp are nonlinear smoothing functions.
We first fitted the model for the mortality analyses and then for evaluating the thinning effects. We set Δmor and Δthin as response variables, intensity and mode (of mortality and thinning) as predictor variables and trees within plots and measurements as a random vector to cover any unknown variability of different study plots. We assessed the significant effect of tree mortality and thinning practices on the structural indices (p-values < 0.05) and the direction of changes (positive / negative).
Using the GAMM, we also tested the relationship between the mortality rate and thinning intensity, the relationship between intensity and mode of both mortality and thinning for European beech and sessile oak trees, and the changes in intensity and mode of both mortality and thinning during the stand development (expressed by stand age).
Furthermore, we used a GAMM to assess the tendency of changes in the height development of trees, as related to natural mortality and thinning practices. The smooth curves deduced from the model show how the natural mortality and thinning influenced the height of beech and oak trees in unmanaged and managed stands. We conducted the analysis once for all trees in the mixed stands and once for beech and oak separately. In our regression analyses, in order to find influential outliers, we calculated the studentized residuals. If an observation had a studentized residual larger than 3 (in absolute value) we counted it as an outlier and excluded it from our predictor variables sets. We repeated the regression analyses, and if the exclusion of the possible outliers did not significantly change the results, we put those omitted observations back into the regression calculations.
Data preparation, indices calculations and analyses were carried out in R statistical software ver. 3.5.0 ([37]). We used the “mgcv” package to fit the generalised additive mixed effects models, and the “ggplot2” package combined with “ggpubr” package to visualise the results.
Results
Natural mortality and thinning
Fig. 1a illustrates a negative correlation between the mortality rates and thinning intensities on the study plots at a 95% confidence interval; however, the correlation was only significant for oak trees (p-value < 0.05). Fig. 1b shows the positive correlation between the mortality mode and mortality rate for both species, explaining that the increase in mortality rate results in a significant increase in drop-out of trees of smaller than the average size (p-value < 0.001). Fig. 1c shows the statistically significant (p-value < 0.001) negative relationship between the thinning mode and intensity for beech, where the high intensity thinning removed medium-sized to large trees. The trend was statistically significant (p-value < 0.001) but in the opposite direction for oak trees, whereby mostly small trees were thinned out while maintaining a higher number of large trees.
Fig. 1 - The relationship between (a) mortality rates and thinning intensities on the study plots at a 95% confidence interval; (b) mortality mode and mortality rate for European beech and sessile oak; (c) thinning mode and intensity for sessile oak and European beech.
We also detected significant changes (p-value < 0.05) in mortality rate / thinning intensity and mortality mode / thinning mode related to stand age, at the plot and at species level. As illustrated in Fig. 2a, the mortality rate of oak was almost similar to the mortality rate of the entire plot, whereas a much lower mortality rate was detected for beech, which could be due to senescence at older ages. Fig. 2b shows that the high mortality in oak was mostly related to the drop-out of small oak trees of below-average diameter. The mortality rate of smaller oak trees decreased up to the age of 100 years (Fig. 2a). After that age, most of the mortality occurred among the larger trees, which could be due to senescence. Mortality at a young age (age < 60) in beech was related either to trees of around average size or larger, or to the death of small trees in stands older than 60 years (Fig. 2b).
Fig. 2 - (a) The mortality rate and thinning intensity of the studied plots, at the plot and at species level related to stand age, where changes by stand are significant (p-value < 0.05); (b) The mortality and thinning mode of the studied plots, at the plot and at species level related to stand age, where changes by stand are significant (p-value < 0.05).
Fig. 2a shows that the thinning intensity was a lot higher for beech compared to oak, as the curve of thinning intensity for beech was similar to the thinning intensity of the entire stand. Fig. 2b shows that mostly smaller than average oak trees (thinning mode > 1) and larger than average beech trees (thinning mode < 1) were thinned. Thus, the high thinning intensity of beech tree is due to the removal of many large beech trees.
Effects of natural mortality and thinning on structure and competition
We presented a general overview of the quantified structural and competition indices in Tab. 2 and the effect of natural mortality and thinning on stand structure in Tab. 3. We assessed the effect of natural mortality and thinning by indices divided into four groups: indices counting for (i) tree size distribution, (ii) stand density and horizontal distribution patterns, (iii) species intermingling and for (iv) competition.
Tab. 2 - The quantified structural and competition indices for the studied plots in total and for the two main species. The means are given in plain text, and standard deviations in italics. The meaning of each acronym is reported in Tab. S1 (Supplementary material).
Data | Species | N | D q | BA | SDI | Con | Agg | I P | Dif | Dom | D var | Ming | Seg | CI Beech | CI Oak |
---|---|---|---|---|---|---|---|---|---|---|---|---|---|---|---|
phase 0 | Total | 1042 843 |
270.6 124.1 |
34.3 6.3 |
714.0 164.6 |
0.56 0.02 |
0.99 0.09 |
1.03 0.17 |
0.47 0.07 |
0.52 0.01 |
56.84 11.94 |
0.41 0.11 |
-0.16 0.17 |
1011.6 1017.8 |
873.7 1165.3 |
Beech | 670 491 |
201.6 92.7 |
13.6 5.7 |
309.2 107.2 |
0.56 0.03 |
0.96 0.12 |
1.04 0.23 |
0.45 0.05 |
0.39 0.08 |
51.41 10.3 |
0.34 0.17 |
-0.15 0.17 |
861.2 851.0 |
654.4 615.8 |
|
Oak | 464 606 |
357.4 154.0 |
21.9 6.3 |
414.6 163.1 |
0.55 0.03 |
1.04 0.12 |
1.00 0.22 |
0.50 0.11 |
0.82 0.11 |
20.84 6.34 |
0.68 0.20 |
-0.19 0.19 |
185.3 305.1 |
303.0 774.9 |
|
phase mor | Total | 952 731 |
274.3 122.8 |
33.6 6.0 |
692.5 146.0 |
0.56 0.02 |
0.99 0.09 |
1.03 0.17 |
0.46 0.07 |
0.52 0.01 |
55.36 11.47 |
0.42 0.11 |
-0.17 0.16 |
877.6 915.9 |
722.7 857.7 |
Beech | 625 450 |
204.5 92.0 |
13.4 5.6 |
302.3 103.0 |
0.56 0.03 |
0.96 0.12 |
1.05 0.26 |
0.45 0.05 |
0.38 0.08 |
50.21 10.05 |
0.34 0.16 |
-0.16 0.17 |
757.5 785.8 |
574.6 531.9 |
|
Oak | 401 470 |
359.1 160.0 |
21.3 6.1 |
398.1 142.9 |
0.55 0.03 |
1.04 0.13 |
1.00 0.17 |
0.50 0.12 |
0.82 0.10 |
19.62 4.59 |
0.69 0.19 |
-0.20 0.19 |
146.3 210.5 |
200.8 456.7 |
|
phase thin | Total | 892 710 |
274.3 128.9 |
30.5 6.6 |
630.7 156.4 |
0.56 0.02 |
1.00 0.08 |
1.02 0.16 |
0.46 0.07 |
0.52 0.02 |
55.69 12.06 |
0.41 0.11 |
-0.16 0.15 |
793.7 861.3 |
647.5 816.0 |
Beech | 599 430 |
199.0 89.6 |
12.3 5.6 |
278.4 101.3 |
0.56 0.04 |
0.96 0.12 |
1.02 0.23 |
0.45 0.06 |
0.38 0.08 |
49.84 10.5 |
0.34 0.18 |
-0.15 0.16 |
695.6 736.6 |
521.4 507.4 |
|
Oak | 393 481 |
361.1 158.6 |
19.8 5.6 |
373.0 144.0 |
0.55 0.03 |
1.05 0.12 |
0.99 0.18 |
0.49 0.12 |
0.81 0.11 |
19.67 5.02 |
0.66 0.21 |
-0.20 0.18 |
139.9 208.9 |
199.2 451.6 |
Tab. 3 - Summary analyses of the effect of natural mortality (intensity and mode) and thinning practices (intensity and mode) on the stand structure. (sp*): significant difference between Oak and Beech species (p-value < 0.05); the direction of relationship is shown with + and -, showing positive and negative correlation, respectively and the first +/- sign is for Beech species and the last is for Oak. The meaning of each structural and competition acronym is reported in Tab. S1 (Supplementary material).
Treatment | Natural mortality | Thinning | Index type | ||
---|---|---|---|---|---|
No effect | Effect | No effect | Effect | ||
Intensity | BA | Dqsp*(+,+), Domsp*(-,+), Dvarsp*(-,-), Dif(-,-) | Dif, D var | Dqsp*(-,+), BAsp*(-,-), Dom(-,-) | Size distribution |
Mode | - | Dqsp*(+,+), BAsp*(-,-), Difsp*(-,-), Dvarsp*(-,-), Dom(-,-) | Dq, BA, Dvar | Difsp*(+,-), Dom(-,-) | |
Intensity | Con | Nsp*(+,-), Aggsp*(-,+), IP(+,-), SDIsp*(+,-) | Con | N(-,-), Aggsp*(-,+), IPsp*(+,-), SDI(-,-) | Density and distribution pattern |
Mode | Con | Nsp*(-,-), Aggsp*(+,+), Ip(-,-), SDIsp*(-,-) | N, Con, SDI | Aggsp*(-,+) , Ip(-,-) | |
Intensity | - | Mingsp*(-,+), Seg(+,+) | - | Mingsp*(+,-), Seg(+,+) | Species interming-ling |
Mode | - | Mingsp*(+,-), Seg(-,-) | Seg | Mingsp*(+,-) | |
Intensity | CI Oak | CIBeechsp*(+,-) | - | CIBeechsp*(-,-), CIOak(-,-) | Competition |
Mode | - | CIBeechsp*(-,+), CIOaksp*(-,+) | - | CIBeech(+,+), CIOak(+,+) |
Tree size distribution
The intensity and the mode of mortality and thinning had a significant effect on the size distribution of trees within the study plots (Tab. 3). The removal of rather large beech trees, in the case of low mortality rates (Fig. 1b) and high thinning intensities (Fig. 1c) reduced the quadratic mean diameter (Dq) of beech. In the case of higher mortality rates, the death of small beech trees increased the Dq of beech. In oak trees, the quadratic mean diameter increased with a rising both in mortality rate and thinning intensity. This can be explained through the mortality and thinning of smaller oak trees (Fig. 1c). The basal area (BA) of both species was reduced due to removal of oak and beech trees through natural mortality and thinning practices.
The dominance status of trees (Dom) due to natural mortality changed significantly for the two studied species (Tab. 3). Sessile oak showed a higher mortality rate than beech (Fig. 1b), which resulted in the death of many small oak trees, and consequently increased the dominance of remaining larger oak over their direct neighbours. In managed stands, a larger number of small oak (also beech) trees were thinned out in high-intensity thinning practices for oak (about 20-25 %, see Fig. 1c). This increased the probability of larger remaining trees occurring in close proximity to reference oak trees, and caused a reduction in the dominance of oak.
The diameter differentiation of trees (Dif) reflects that an increased rate and mode of mortality led to a rise in the homogeneity of the diameters of oak and beech trees. In the case of a high mortality rate and mode (Fig. 1b), a higher number of small trees dropped out, and consequently the difference in diameter between the measured reference trees and their neighbours decreased. In managed stands, the thinning of rather large beech trees (thinning mode < 1) and small oak trees (thinning mode > 1), increased the difference in the diameter of beech trees and decreased it for oak.
The non-spatial measure of diameter variation (Dvar) showed that an increasing mortality rate lowered the diameter variation for beech and oak trees through the drop-out of rather smaller trees. Consequently, the mortality of a high amount of smaller trees (oak followed by beech, see Fig. 1b) and the survival of larger trees, made the stands more homogeneous in terms of tree size.
Stand density and horizontal distribution pattern
Among the second group of indices, accounting for density and distribution patterns of trees within the stands, the number of beech trees per hectare (N) increased in the case of a high mortality rate. This can be explained by the long time span between measurements, which was long enough for the ingrowth of small beech trees. As expected, thinning decreased the number of trees per hectare for both species. In this context, the decreased value of stand density index (SDI) in the case of a high thinning intensity was evident, because SDI depends directly on the number of tree per hectare.
As shown by the indices Agg and Ip, the distribution pattern of trees within the stands changed as a result of natural mortality and thinning practices (Tab. 3). Both thinning and mortality led to a more regular distribution of the remaining oak and beech trees through the drop-out of suppressed trees or through releasing target trees of disturbing neighbours (higher value of Agg and smaller values of IP). The beech trees exhibited a slight tendency towards clumping, in the case of a high thinning intensity, high mortality rate and thinning of large trees. Many small oak trees died out in the stands with high mortality rates (> 8.3%), which resulted in a lower density and higher regularity (see higher Agg and lower Ip in Tab. 2). During the thinning phase, the mean distances to the immediate neighbours decreased through the elimination of a high number of large beech trees standing large distances from their nearest neighbours.
Tree species intermingling
In the third group of indices, Ming accounted for four nearest neighbours and revealed that oak trees appeared mostly in mixed groups. An increasing mortality rate increased the mixture level of the remaining oak trees. On the other hand, we realized lower degrees of species mingling among beech trees. An increase in the mortality rate, mostly due to the drop-out of oak trees, even decreased the species intermingling of beech central trees and their surrounding oak trees. The positive correlation between the mortality mode and mingling in beech trees showed that large surviving beech trees tend to be more intermingled with oak trees compared to small beech trees. Thinning practices counteracted by removing more beech trees and increasing the relative share of oak within the stand, resulted in a drop in the mingling level of oak trees and an increase in the mingling level of beech trees (Tab. 3).
Accounting only for the closest neighbour (Seg), we found an increased segregation for both species in the case of high mortality rates and high thinning intensities. This can be explained by a lower number of mixed oak-beech pairs, potentially due to the death of many oak trees in the case of high mortality rates (> 8.3%). Those lessened the chance of beech trees having an oak tree as a direct neighbour. Another reason was the thinning of many large beech trees, which increased the chance for oak central trees to have other oak trees as neighbours. However, the remaining presence of large beech and oak trees after natural mortality decreased species segregation, and the thinning mode demonstrated no significant effect on the Seg values of either species (Tab. 3).
Tree competition
An examination of the competitive status of beech and oak trees (CIBeech and CIOak) showed that the competition posed by beech vis-à-vis oak trees declined during the mortality phase, which might be explained by the mortality of suppressed oak trees suffering from a high competition load imposed by the neighbouring beech trees. Thinning practices reduced the competition induced by oak and beech through releasing the reference trees and eliminating the competing neighbours of both species.
Regarding the competition influences of beech on oak, we also compared the height development of oak and beech trees in unmanaged and managed plots, with different thinning intensities. As illustrated in Fig. 3, oak appeared to be dominant in height compared to beech; however, in unmanaged plots older than 150 years of age, beech dominated oak in terms of height. Oak trees were more dominant in height within managed stands where thinning practices favoured oak trees by thinning large beech trees, as shown in Fig. 2b.
Fig. 3 - The height development of sessile oak and European beech trees in managed and unmanaged plots.
Discussion
In our study, the structural processes in mixed beech-oak stands became visible in different structural traits. The analysis of tree-size distribution, spatial distribution, and competition at individual tree and stand level in unmanaged and managed plots demonstrates the clear dominance of European beech, and how the interspecific competition can be modified by thinning. We therefore reject our starting hypothesis, assuming a similar effect of tree removal during natural mortality or/and thinning.
Mortality and thinning significantly modified the tree-size distribution. Through natural mortality only a few large beech trees, small beech trees and many small oak trees dropped out (Fig. 1b), showing that the regeneration of oak was difficult in unmanaged stands. With increasing thinning intensity, a higher number of large beech trees were cut (Fig. 1b). The mean quadratic diameter of beech therefore decreased with thinning, and that of oak increased. The change in tree-size distribution in the analysed beech and oak stands of this study explains the concerns of producers of high-quality oak wood in regions of mixed beech-oak forests. In the case of set-aside plans or restricted silvicultural management, their concern is a high mortality of sessile oak ([18]), or at least a lower production of high-quality oak wood due to the lacking option of supporting oak growth ([48]). Still, sessile oak occurs naturally in pristine forests as long as gaps open up during the decay stage and provide the regeneration with enough light. Natural disturbance could provide those gaps needed for the regeneration of oak ([20]). In most managed continuous-cover beech stands, the phase of late senescence with natural decomposition and the opening of gaps, when old trees naturally die, is shortened through harvesting at earlier points in time, and a fostering of the regeneration of oak would therefore still be necessary e.g., by artificially providing gaps. The discussion of abandoning managed forest areas for conservation must address how to keep oak viable, which is considered a challenge ([46]). The structural heterogeneity of forests in once managed and abandoned forest stands was found to be lower than in both managed stands and pristine forests ([43]). Since oak trees in mixtures with shade-tolerant species are more likely to die, the share of oak is expected to decrease after sudden abandonment.
The effects of mortality and thinning became visible also in the spatial distribution of the two species. Both mortality and thinning decreased the tree density and led to a higher regularity in the horizontal distribution patterns of trees within the stand. Natural mortality slightly reduced the clumping pattern in beech and made the spatial distribution of beech more homogenous. This is a logical consequence due to the density-dependent mortality, or self-thinning, when the regeneration of light-demanding species is impeded by the shading of larger trees in their proximities. Trees growing close to strong neighbours are therefore more susceptible to stress and more likely to die ([45]). The remaining trees will have a more random or even a regular horizontal distribution ([29]). Comparable to the effect of natural mortality, thinning released oak trees by removing the closest neighbouring beech trees. This led to a larger growing space and a higher survival rate for oak. The horizontal distribution of oak trees thus became more regular, which is the usual effect of thinning with the aim of reducing competition ([24]).
Over the stand development, the intermingling of oak and beech trees increased during the mortality phase. As a shade tolerant r-strategist species ([16]), beech usually occupies the gaps made available through the drop-out of large and old trees or any other disturbances. That increased the probability for already-existing oaks to obtain more beech trees as their nearest neighbours. This natural process of beech replacing and suppressing oak by occupying each possible location became visible in our study and from other previous studies ([23]). With progressing stand development, it is mainly the suppressed oak trees which drop out ([45]). Strong surviving oak trees therefore remain at an appropriate distance from neighbouring beech trees and can enlarge their growing space. This decreases the possibility of beech having oak in close proximity. After thinning, the intermingling of oak declined because of the removed neighbouring beech trees, which resulted in an increased intermingling of beech. To regulate the interspecific competition, thinning practices often aim at maintaining a high number of oaks in the overstorey while restraining beech ([48]). This might explain our finding of decreased and increased intermingling in oak and beech surroundings, respectively. Interspecific competition can thus be managed by controlling the level of intermingling in mixed stands.
Natural competition is an important factor in mixed-species stands, particularly when species depend on the same resources ([44]). As the analysed long-term experimental plots used in this study have a favourable water and nutrient supply, most of the intra- and inter-specific competition is for light ([33]). The competition for light is especially influenced by the vertical and horizontal structural characteristics of a stand. Note that the sensitivity to competition, crowding and overtopping changes during the ontological development of trees, and that the changes are different for each species ([42]), which could not be well reflected in this study by using only one competition index. In any case, an analysis of the competition in mixed beech-oak stands shows the high competitiveness of European beech, as evidenced by the higher number of trees and its relatively wide crown compared to sessile oak (see Tab. 1). The strong competitiveness of beech and the outcompeting of oak ([18]) also became visible in our study, with a higher mortality rate for oak than for beech and lower final tree heights of oak in unmanaged stands compared to managed stands (Fig. 3). We found that oak experienced less competition from beech upon increasing natural mortality. The competition load on trees of both species, especially of oak, was reduced both by the drop-out of suppressed small trees, and by thinning. Thinning therefore intensified the natural process of self-thinning, through the cutting of suppressed, competing neighbour trees to promote the growth of target trees ([4]). Consequently, our analysis showed that in the case of a high natural mortality of beech, a lower intensity of thinning was necessary and vice versa. On the experimental plots, thinning operations in favour of oak were required in order to maintain the mixture of beech and oak over all phases of stand development ([23]). Even though the experimental plots used in this study were implemented for testing different intensities of thinning, their management might differ from that in private-owned forests. Nonetheless, the experimental plots may be useful for providing an idea of the management effect in mixed beech and oak stands in the long term ([36]).
Conclusions
Sessile oak in mixed beech-oak stands is usually overshadowed and suppressed, and both its basal area proportion and the number of stems in the stands decrease more strongly than those of beech do. The strong competitiveness of beech over oak is reflected by several stand-structural traits. For maintaining a certain share of oak in mixed beech-oak forests, either regular thinning interventions ([4]) or an initial spatial or temporal separation of the two species ([35]) is necessary. Our results - which are representative of a large area of the common distribution range of European beech and sessile oak in Europe - show the need for thinning interventions when aiming at a sustainable mixture of the two species. In terms of climate change, the competitiveness of European beech might be decreasing and sessile oak is expected to adapt better to climatic changes (such as drought) than beech ([41]). This might make mixed oak and beech stands even more attractive when it comes to facing challenges around future forest ecosystems. Understanding and managing the structural processes behind competition and facilitation between beech and oak can therefore lead to a more stabilised performance of mixed stands providing multiple ecosystem functions.
Acknowledgements
KM and LZ are the two main authors and contributed equally to this manuscript. The authors would like to thank the Bayerischen Staatsforsten (BaySF) for supporting the establishment of the plots, and the Bavarian State Ministry for Nutrition, Agriculture, and Forestry for its constant support of the project W 07 “Long-term experimental plots for forest growth and yield research” (#7831-22209-2013). Thanks are also due to anonymous reviewers for their constructive criticism. This research received funding from the European Union for the project “Mixed species forest management. Lowering risk, increasing resilience (REFORM)” (# 2816ERA02S) under the framework of Sumforest ERA-NET.
References
Gscholar
CrossRef | Gscholar
Gscholar
Gscholar
Gscholar
Gscholar
CrossRef | Gscholar
Gscholar
Gscholar
CrossRef | Gscholar
Gscholar
Gscholar
Gscholar
CrossRef | Gscholar
CrossRef | Gscholar
Gscholar
Gscholar
Supplementary Material
Authors’ Info
Authors’ Affiliation
Institute of Forest Research, University of Quebec in Abitibi-Temiscamingue, 445 boul. de l’Université, Rouyn-Noranda, QC J9X 5E4 (Canada)
Hans Pretzsch 0000-0002-4958-1868
Chair for Forest Growth and Yield Science, School of Life Sciences Weihenstephan, Technical University of Munich, Hans-Carl-von-Carlowitz-Platz 2, 85354 Freising (Germany)
Corresponding author
Paper Info
Citation
Maleki K, Zeller L, Pretzsch H (2020). Oak often needs to be promoted in mixed beech-oak stands - the structural processes behind competition and silvicultural management in mixed stands of European beech and sessile oak. iForest 13: 80-88. - doi: 10.3832/ifor3172-013
Academic Editor
Giorgio Alberti
Paper history
Received: Jun 19, 2019
Accepted: Jan 02, 2020
First online: Mar 01, 2020
Publication Date: Apr 30, 2020
Publication Time: 1.97 months
Copyright Information
© SISEF - The Italian Society of Silviculture and Forest Ecology 2020
Open Access
This article is distributed under the terms of the Creative Commons Attribution-Non Commercial 4.0 International (https://creativecommons.org/licenses/by-nc/4.0/), which permits unrestricted use, distribution, and reproduction in any medium, provided you give appropriate credit to the original author(s) and the source, provide a link to the Creative Commons license, and indicate if changes were made.
Web Metrics
Breakdown by View Type
Article Usage
Total Article Views: 33857
(from publication date up to now)
Breakdown by View Type
HTML Page Views: 29512
Abstract Page Views: 1837
PDF Downloads: 1992
Citation/Reference Downloads: 11
XML Downloads: 505
Web Metrics
Days since publication: 1609
Overall contacts: 33857
Avg. contacts per week: 147.30
Article Citations
Article citations are based on data periodically collected from the Clarivate Web of Science web site
(last update: Feb 2023)
Total number of cites (since 2020): 13
Average cites per year: 3.25
Publication Metrics
by Dimensions ©
Articles citing this article
List of the papers citing this article based on CrossRef Cited-by.
Related Contents
iForest Similar Articles
Research Articles
How biomass and other tree architectural characteristics relate to the structural complexity of a beech-pine forest
vol. 16, pp. 368-376 (online: 19 December 2023)
Research Articles
A Decision Support System for trade-off analysis and dynamic evaluation of forest ecosystem services
vol. 11, pp. 171-180 (online: 19 February 2018)
Research Articles
Long-term effects of thinning and mixing on stand spatial structure: a case study of Chinese fir plantations
vol. 14, pp. 113-121 (online: 08 March 2021)
Research Articles
Green oriented urban development for urban ecosystem services provision in a medium sized city in southern Italy
vol. 7, pp. 385-395 (online: 19 May 2014)
Research Articles
Do different indices of forest structural heterogeneity yield consistent results?
vol. 15, pp. 424-432 (online: 20 October 2022)
Research Articles
Effects of silvicultural thinning on stand structure and coarse woody debris in the deciduous Arasbaran forest, Iran
vol. 16, pp. 377-384 (online: 19 December 2023)
Research Articles
An index of structural complexity for Apennine beech forests
vol. 8, pp. 314-323 (online: 03 September 2014)
Research Articles
Interactions between thinning and bear damage complicate restoration in coast redwood forests
vol. 13, pp. 1-8 (online: 08 January 2020)
Research Articles
Development phases and structural characteristics of the Penteleu-Viforta virgin forest in the Curvature Carpathians
vol. 13, pp. 389-395 (online: 01 September 2020)
Research Articles
Deriving tree growth models from stand models based on the self-thinning rule of Chinese fir plantations
vol. 15, pp. 1-7 (online: 13 January 2022)
iForest Database Search
Google Scholar Search
Citing Articles
Search By Author
Search By Keywords