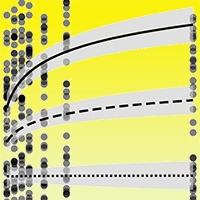
Effect of different dolomitic limestone dosages on soil respiration in a mid-altitudinal Norway spruce stand
iForest - Biogeosciences and Forestry, Volume 12, Issue 4, Pages 357-365 (2019)
doi: https://doi.org/10.3832/ifor2894-012
Published: Jul 05, 2019 - Copyright © 2019 SISEF
Research Articles
Abstract
The study focuses on the effect of chemical amelioration of dolomitic limestone (doses of 0, 2, 3, 4, 6, 9 and 26 t ha-1) on soil respiration in a Norway spruce monoculture in mid-altitudinal elevation during one-year period after application. Firstly, the soil respiration was measured in situ as monthly CO2 efflux from the soil surface horizon in the period May to October 2016. Secondly, basal respiration, microbial biomass carbon and metabolic quotient of the organic H and organo-mineral A horizons were assessed under laboratory conditions within one year after the treatment. Soil CO2 efflux increased by 3 to 31% and by 29 to 98% for the ameliorant of 2 and 26 t ha-1, respectively, compared to the unlimed control treatment. The CO2 efflux was significantly driven by external conditions such as soil moisture and temperature, especially in the last seasonal months. Basal respiration of the H horizon increased up to a dose of 9 t ha-1 but decreased at 26 t ha-1. In the A horizon, microbial activity increased in all the limed variants compared to the non-limed variant. A similar trend was observed in microbial carbon and the metabolic quotient of the soil. Our results prove that the ameliorant doses commonly used in the forestry sector (3-4 t ha-1) substantially increase the soil microbial activity during (soil CO2 efflux) and after (laboratory data) the first year after application. This results in the accelerated mineralization of soil organic material and subsequent loss from the forest ecosystem.
Keywords
Amelioration, Basal Respiration, Liming, Picea abies, Soil CO2 Efflux
Introduction
Lime treatment of forest stands has had a fairly long history in Central Europe ([45]). Historically, lime was used to reduce soil acidification as a negative effect of acidic deposition; it is currently used as a method of supplying the missing nutrients that are blocked in the forest floor ([48]). Liming in the Czech Republic reached the highest intensity during the air pollution crisis in the 1970s and 1980s ([21]). In particular, it was applied on mountain areas of the Czech borders in the dose of 3 t ha-1 ([47]). Within the early 21st century, liming was also targeted to mid-altitudinal forests to improve nutrient supply. In addition to the well-known immediate effects of liming on soil pH and nutrient supply, liming widely influences many aspects of the soil environment, which have not been well identified so far ([39], [9]), such as mineralization rate, microbial biomass, metabolic quotient, etc. However, while liming may only be a temporary solution, it is still commonly applied.
Liming results in a number of alterations, mostly in topsoil ([17]). Liming influences the soil sorption complex by increasing the base saturation ([31]), especially via bivalent base cations (Ca2+ and, in case of dolomitic limestone use, Mg2+ - [19]), and increasing of pH. Nevertheless, liming involves risks brought about by antagonism with potassium (K+), which is susceptible to leaching and blocked for nutrition availability ([53]) and organic matter mineralization. The latter one results from an increase in microbial activity and influences the soil water regime and water retention capacity ([37]), mineral nutrient mobilization ([44]) and nitrogen dynamics ([13]). Liming can also end in the redistribution of fine roots towards the topsoil, which especially in Norway spruce forests ([26]) increases the risk of drought stress ([34]) and uprooting.
The influence of liming on soil chemistry is manifested by edaphon functional group composition and biological activity ([39]). An increase in bacterial populations leads to a decrease in the fungi-to-bacteria ratio ([4]) and an increase in the mineralization intensity of organic matter, which can be measured as a release of nutrients and increase in soil respiration/CO2 efflux ([20]). The response of soil biota to liming can be assessed by monitoring of soil respiration ([38]), microbial biomass carbon and metabolic quotient reflecting energy maintenance ([3]). The soil microbial activity is closely related to temperature and moisture ([7]), thus it can be presumed that the effect of liming will differ under the influence of climate when measured in the field.
Some studies ([2], [29], [9]) found liming as an ambiguous, temporary but rather effective amelioration management. Only a few studies in the literature deal with the influence of liming on soil biota; therefore, a critical question regarding the significance of liming on soil respiration and related parameters is still unsolved. Indeed, soil biota response is estimated rather than precisely measured and the response will probably differ according to doses of the ameliorant, especially using extreme amounts.
The aim of the study is to quantify the effect of liming on the biological activity of topsoil in relation to the different doses of dolomitic limestone (0, 2, 3, 4, 6, 9, 26 t ha-1). The hypotheses were the following: (1) soil respiration will increase after liming; and (2) this effect will increase with a limestone dosage.
The respiration was measured (i) in situ as CO2 efflux from the soil surface monthly from May to October 2016 in the year following the application of the ameliorant (November 2015); and (ii) in the laboratory as soil basal respiration using gas chromatography at one year after applying the ameliorant. As supplementary data, the microbial biomass carbon and metabolic quotient were determined. The study is unique from the point of view of the following aspects: (1) the mid-altitudinal elevations (previous studies focused on mountain forests); (2) the wide range of doses of the ameliorant; and (3) the high number of selected investigated parameters (in situ soil CO2 efflux, soil moisture and temperature, basal respiration, microbial biomass carbon, metabolic quotient, soil pH).
Materials and methods
Site description
The experimental plots were established at the Field Research Station Rájec-Nemčice, Drahanská vrchovina region (Czech Republic - 49° 26′ 32″ N, 16° 41′ 52″ E; 623 m a.s.l.; flat terrain; moderately warm and humid climate; mean annual temperature 8.15 °C; mean annual precipitation 631 mm). According to the FAO classification system ([16]), the soil was classified as Haplic Cambisol with acid granodiorite as bedrock. Potential vegetation in terms of forest site complexes ([52]) was classified as Fagetum mesotrophicum (nutrient-medium Beech); actual vegetation is composed of 100 % Norway spruce monoculture (Picea abies [L.] H. Karst) aged 110 years with 70-75% coverage in the first generation and with 30% undergrowth coverage of Small balsam (Impatiens parviflora).
In November 2015, the study plot was sampled prior to the treatment. The soil was characterized by extremely acidic pH and unsaturated soil sorption complex (Tab. 1). The H humus horizon thickness was 3.0-4.5 cm, the A organo-mineral horizon thickness was 1.5-2.5 cm, stock of organic matter in the surface organic horizons was 94.05 t ha-1 on average (standard deviation = 19.65).
Tab. 1 - Selected soil properties characterizing the initial state in horizons H and A - November 2015. (sd): standard deviation; (n): number of repetitions; (pH/H2O): active soil pH; (pH/KCl): exchangeable soil pH; (Corg): organic (oxidizable) carbon content; (Cmic): microbial biomass carbon; (BasResp): basal respiration; (qCO2): metabolic quotient; (CEC): cation exchange capacity; (BS): base saturation; (C/N): carbon-to-nitrogen ratio; (dw): dry weight.
Variable | Units | H horizon (n = 13) | A horizon (n = 11) | ||
---|---|---|---|---|---|
mean | sd | mean | sd | ||
pH/H2O | - | 3.41 | 0.12 | 3.42 | 0.04 |
pH/KCl | - | 2.81 | 0.15 | 2.61 | 0.06 |
Corg | % | 32.24 | 2.75 | 9.77 | 0.9 |
Cmic | µg C g-1 dw | 1261.94 | 131.84 | 282.22 | 68.64 |
BasResp | µg C-CO2 h-1 g-1 dw | 3.52 | 0.55 | 1.31 | 0.19 |
qCO2 | µg C-CO2 mg-1 Cmic h-1 | 2.8 | 0.5 | 4.9 | 1.1 |
CEC | cmolc kg-1 | 36.42 | 2.52 | 34.61 | 0.89 |
BS | % | 17.67 | 3.11 | 8.26 | 1.13 |
C/N | % | 23.46 | 2.06 | 31.73 | 2.99 |
Experimental design and soil sampling
The homogeneous 35 × 20 m experimental plot was divided into 7 sampling subplots of 5 × 20 m, which were mutually isolated using PVC foil to the depth of 50 cm. Each subplot was spread with a different dose of finely ground dolomitic limestone (the doses of 0, 2, 3, 4, 6, 9, 26 t ha-1). The doses of 2, 4 and 6 t ha-1 were used to monitor the soil response near the standard dose of 3 t ha-1 ([48], [47]); the doses of 9 and 26 t ha-1 were used to study soil response under the extremely high amounts of the ameliorant. At each subplot, in the longitudinal axes, seven PVC rings were pre-installed for the duration of the experiment (15 cm high, embedded 3 cm deep in the soil; 49 rings in total) at the distance of 3 m to measure CO2 efflux (see below).
One year after liming (November 2016), a total of 21 soil samples were taken both from the horizons H (organic horizon of humification) and A (organo-mineral horizon), 3 from each lime treatment variant for the H and A horizons. The samples were sieved through a 2-mm sieve and conserved fresh at 4 °C for the time period of two weeks prior to laboratory analysis (assessment of basal respiration and microbial biomass carbon); the soil samples were air-dried and sieved through a 2-mm sieve for the subsequent assessment of pH.
To avoid measuring any CO2 incoming from the CaCO3 chemical dissolution, we verified the presence/absence of free carbonates in the soil using volumetric quantification with 4 mol HCl according to ISO-10693 ([22]).
Measurement of soil CO2 efflux in situ and expression of R10
In the time period May to October 2016, soil CO2 efflux was measured from 08:00 to 11:00 a.m. at the end of every month. The CO2 efflux was measured using a portable system LI-COR LI-8100A® (Li-Cor, Lincoln, NE, USA) with a 20-cm-diameter chamber, fitted on the pre-installed PVC rings. After closing a chamber, a period (dead band) of 15 s was set to allow steady mixing of the air in the chamber. During the following 60 s, the CO2 concentration was measured repeatedly at 1-s intervals, and a linear approach was used to calculate the soil CO2 efflux.
During each measurement, the soil temperature (°C) at 1.5 cm (TPD32 penetrate thermometer, Omega, Stamford, CT, USA) and soil moisture (%vol) in the 0-6 cm profile (ThetaProbe ML2x®, Delta-T Devices, Cambridge, UK) were measured at a distance of 5 cm outside the PVC ring for a minimum of three points for each measurement position.
Within the forest stand close to the experimental plots, the continuous measurements of soil CO2 efflux were carried out applying an automated closed (non-steady-state through-flow) system (developed at the Global Change Research Institute in Brno, CZ) with six chambers. The design and installation were described by Darenova et al. ([14]). Within each chamber, the soil temperature (thermometers PT-100®, Treston a.s., CZ) was measured simultaneously with soil CO2 efflux at the depth of 1.5 cm. Data from the continuous measurements were used to determine the temperature sensitivity of soil CO2 efflux during the periods of individual manual measurement periods.
Soil CO2 efflux (Rs) from the continuous measurements by the automated system from one week containing the measurement campaign was plotted against soil temperature (Temp) and this was expressed by an exponential regression curve with the regression equation (eqn. 1):
where α
and β
are the regression coefficients. Mean Q10 (the proportional change in CO2 efflux in relation to a 10 °C increase in temperature) from six chambers was calculated according to Lloyd & Taylor ([30] - eqn. 2):
being α
the regression coefficient from eqn. 1.
The Q10 values were used to normalize soil CO2 efflux from each position of the liming experiment for the temperature 10 °C (R10) according to the following equation (eqn. 3):
Laboratory determination of soil properties
Laboratory analyses were performed in autumn 2016. The basal soil respiration (soil native CO2 release) was determined according to the soil analyses guidelines published by the Central Institute for Supervising and Testing in Agriculture ([57]) and the International Organization for Standardization ([23]). The measurement was performed using the YL 6500GC® gas chromatograph (Soft Clarity Next Generation, YL Instruments Ltd., Anyang, Korea). The incubation was carried out for 24 hours at a constant temperature of 22 °C. The CO2 concentration was determined using an all-purpose detector PDD (Pulsed Discharge Detector).
Microbial biomass carbon (Cmic) was assessed using the fumigation-extraction method according to Vance et al. ([49]) and Joergensen ([25]). The samples were analysed as fumigated (24-hour chloroform fumigation) and as non-fumigated. The organic carbon was extracted from all the samples with 0.5M K2SO4, and the C concentration was analysed by wet combustion ([55]). Briefly, a mineralization mixture consisting of 25M K2Cr2O7, 95% H2SO4 and distilled water was added to the leachate. Mineralization occurred at 135 °C for 40 minutes. The soil extract absorbance was measured spectrophotometrically at a wavelength of 340 nm.
The metabolic quotient (qCO2) was calculated according to ISO-16072 ([23]), with qCO2 [µg C-CO2 mg Cmic-1 h-1] = basal respiration [µg C-CO2 h-1 g-1 dw] / Cmic [µg C g-1 dw].
Soil pH was determined according to ISO/DIS-10390 ([24]). It was assessed as both active (pH/H2O) and exchangeable (pH/KCl) in water or 1M KCl, respectively, for the air-dried soil samples for a soil:eluate ratio of 1:5 (horizon H) and 1:2.5 (horizon A). For the measurement, a glass electrode WTW SenTix 81™ (Thermo Fisher Scientific, Whaltham, MS, USA) combined with a fluid electrolyte and temperature sensor was used.
Statistical analysis
Analysis of variance (ANOVA) and Fisher’s LSD test were performed using the software package Statistica® ver. 12 (StatSoft, Tulsa, OK, USA). One-way ANOVA at a significance level α = 0.05 (95% confidence interval) was used for the comparison of soil properties of the individual liming variants. Post-hoc multiple comparisons was used in the case of significant differences among groups, using the Fisher’s LSD test.
We tested statistical relations of CO2 efflux depending on either the categorical variables (lime doses, months) or the continuous variables (temperature, moisture).
Correlation and linear regression were carried out in the R language and environment version 3.3.1 ([42]) using RStudio ver. 1.0.126. The correlation was verified via the Pearson’s correlation coefficient using the function “cor()” at a significance level of 0.05 with a critical value of 0.413 for n = 21.
We used a Bayesian version of linear mixed effect models to estimate the effect of limestone addition, time during vegetation season (expressed as the month of measurement) and two more covariates (soil temperature and soil moisture) as well as the significance of these effects. Linear mixed effect models were chosen as measurements for each month on the same plot were repeated (this information was stored in data using plot ID). Plot ID was specified as a random effect in all the models. Variables such as soil moisture, soil temperature, dolomitic limestone dosage and months were considered as fixed effects. All the fixed-effect explanatory variables were considered as continuous, and were scaled before analysis to reduce the multicollinearity of variables when adding the interaction term; scaled estimated coefficients are also directly comparable across models. Limestone dosage values were log transformed (natural logarithm) prior to analysis and the trend of the relationship between limestone dosage and R10 was straighter after transformation. Limestone dosage values were back-transformed to the original values for the graphical presentation of the results. Control (zero addition) was kept as zero also for the transformed limestone dosage. We fitted several models, some with just one fixed-effect explanatory variables, others with their combination. We also included the interaction of months and limestone dosages as preliminary analysis revealed that the effect of limestone dosage on R10 could differ along the vegetation season. All the models were fitted using the package “brms” ver. 2.6.0 ([11]) running in the R environment. Uninformative priors were chosen in all the models (defaults in brms). The fitted models were compared using LOO IC, which is an information criterion based on the “leave-one-out” cross-validation method ([51]). Similarly to the well known Akaike Information Criterion (AIC), the model with smaller LOO IC has better fit to the data. Significance of the fixed-effect variables was evaluated using 95% highest definition intervals (HDI) whether zero value lies within credible values, i.e., whether it is within 95% HDI (see more in [27]). We also reported LOO adjusted version of Bayesian R2 as a measure of goodness-of-fit. For the final model, estimates were reported for the version of the model with unscaled fixed-effect variables.
All the graphs were created using the package “ggplot2” ver. 2.2.1 ([54]).
Results
Soil reaction after liming
Liming influenced pH/H2O in both horizons (Fig. 1a, Fig. 1b), but pH/KCl was significantly affected only in the horizon H. The pH/H2O ratio increased in all the treatments compared to the control; statistical significance was detected in the treatments only in the H horizon (p = 0.0032) at the doses of 4 (p = 0.0236), 6 (p = 0.0028), 9 (p = 0.0004) and 26 t ha-1 (p = 0.0013). The pH/KCl ratio increased only in the H horizon, however without statistical significance. The highest pH increase in comparison with the non-limed area occurred within the H horizon the variant 9 t ha-1 (pH/KCl: 0.29; pH/H2O: 0.46). Within the A horizon, the pH increase was very low in response to the ameliorant doses, but the pH/KCl values remained almost unchanged after liming (Fig. 1b).
Fig. 1 - Means of active (pH/H2O - white bars) and potentially exchangeable (pH/KCl - black bars) soil reaction of the horizons H (a) and A (b) for each liming variant one year after the application of the ameliorant. The error bars denote the standard deviation.
In situ seasonal measurements
Both soil temperature and moisture profoundly changed during the season. Mean soil temperature ranged 17.9 to 21.1 °C, except for October when it dropped to 7.0 °C. Soil moisture was the highest in May (18.5 %) and the lowest in September when it was only 3.7% (with a minimum of 1.8%, which corresponds to almost dry soil). Otherwise, soil moisture ranged 11.9 to 15.4%.
The seasonal pattern of monthly R10 in all the treatments was characterised by the highest values in May and the lowest in September (Fig. 2). The most significant factor affecting R10 during the season was the increasing drought at the end of summer. This fact was mostly evident in September when the lowest R10 was recorded. In October, an increase in soil moisture resulted in a higher R10 compared to September.
Fig. 2 - Medians of normalized soil CO2 efflux for 10 °C (R10) by month and application doses of dolomitic limestone. Upper and lower limits of the box (interquartile range - IQR) denote the 1st and 3rd quantile (Q1 and Q3, respectively); minimum and maximum are given by Q1 - 1.5 IQR and Q3 + 1.5 IQR, respectively; points are outliers.
During the observed period, the average R10 showed statistically significant differences between liming doses during the period May-August (p < 0.05). The largest differences in R10 for the liming dosage were found in May (p < 0.0001). In each month, R10 showed an upward trend with increasing liming intensity. The steepest gradient was found out in May, while the lowest was monitored in September and October (Tab. 2) when the soil CO2 efflux was more subjected to external factors caused by weather conditions than to liming. The exceptions were found in August at the dose of 4 t ha-1 and in September at the doses of 2 and 4 t ha-1, where the lowest R10 of all the variants were observed. Overall, the lowest values were found out in September and only slightly higher in October. The overall dynamics of R10 had a downward trend during the season and the highest liming effect was found in May with a 98% increase at the extreme doses (26 t ha-1). The reference doses, which are the most frequently used in forestry (2-4 t ha-1), resulted in a 7-17% increase in R10 in the all-season average with the lowest response at the dose of 4 t ha-1 (Tab. 2).
Tab. 2 - Mean R10 (in µmol CO2 m-2 s-1) by month and doses of dolomitic limestone expressed as the percentage difference (perc) in the month vs. the control treatment (LimeVol = 0).
LimeVol (t ha-1) |
May | June | July | August | September | October | ||||||
---|---|---|---|---|---|---|---|---|---|---|---|---|
mean | perc | mean | perc | mean | perc | mean | perc | mean | perc | mean | perc | |
0 | 3.13 | 100.0 | 2.91 | 100.0 | 3.69 | 100.0 | 2.49 | 100.0 | 1.13 | 100.0 | 1.51 | 100.0 |
2 | 4.09 | 130.8 | 3.41 | 117.1 | 3.85 | 104.5 | 2.56 | 102.9 | 1.09 | 97.1 | 1.64 | 108.7 |
3 | 4.30 | 137.6 | 3.26 | 112.0 | 4.04 | 109.6 | 2.85 | 114.3 | 1.24 | 110.2 | 1.82 | 120.3 |
4 | 4.00 | 127.9 | 3.44 | 118.1 | 3.92 | 106.2 | 2.15 | 86.2 | 1.10 | 97.9 | 1.60 | 105.6 |
6 | 4.52 | 144.7 | 3.40 | 116.7 | 3.79 | 102.9 | 2.57 | 103.3 | 1.18 | 104.9 | 1.73 | 114.6 |
9 | 4.63 | 148.0 | 3.79 | 130.0 | 4.35 | 117.9 | 2.69 | 107.8 | 1.35 | 119.4 | 1.88 | 124.6 |
26 | 6.18 | 197.6 | 3.89 | 133.4 | 4.75 | 128.8 | 3.36 | 134.8 | 1.22 | 108.7 | 1.77 | 117.2 |
Soil CO2 efflux under influence of seasonal factors and treatments
Respiration was significantly affected by both categorical and continuous variables (Tab. 3). The influence of limestone dosage on the CO2 efflux was affected by external factors. The influence of liming alone was quite weak (M1), while the best fitting model was M11 which combines the limestone dosage, month of measurement and moisture. As both factors condition soil biological activity, the different effect of liming under the influence of seasonal dynamics lies either on moisture, which markedly decreased in September or temperature, which markedly decreased in October (reflected in model M11 by the interaction LimeVol × month).
Tab. 3 - Effect of dosage of dolomitic limestone and other variables (month, moisture and temperature) on R10. All the fitted models are Bayesian mixed effect models with random effect of plot ID. Explanatory variables were scaled before fitting, so the estimated parameters are on scaled scale. (LOO-ajd R2): LOO adjusted Bayesian R2; (LOO IC): information criterion - models with lower value of LOO IC had better fit; SD (ID): standard deviation among random effects in the scale of CO2 efflux. Models are ordered according their LOO IC from the best-fitting model to the worst one. Variables abbreviations: (LimeVol_ln): dosage of dolomitic limestone transformed by natural logarithm; (month): month of measurements; (Moist): soil volumetric moisture; (Temp): soil temperature.
Model No. | Predictor with estimated parameters | LOO-adj R2 | LOO IC | SD(ID) |
---|---|---|---|---|
M11 | 2.92 + 0.18·LimeVol_ln - 0.85·month + 0.47·Moist - 0.20·LimeVol_ln:month | 0.819 | 474.2 | 0.30 |
M9 | 2.92 + 0.18·LimeVol_ln - 0.86·month + 0.47·Moist | 0.787 | 517.2 | 0.28 |
M7 | 2.92 - 0.85·month + 0.5·Moist | 0.786 | 519.9 | 0.33 |
M10 | 2.91 + 0.30·LimeVol_ln - 1.19·month - 0.22·Temp - 0.21·LimeVol_ln:month | 0.726 | 588.5 | 0.16 |
M8 | 2.91 + 0.30·LimeVol_ln - 1.19·month - 0.22·Temp | 0.670 | 614.9 | 0.14 |
M5 | 2.91 - 1.06·month + 0.28·LimeVol_ln | 0.683 | 628.9 | 0.13 |
M6 | 2.92 - 1.19·month - 0.20·Temp | 0.680 | 632.3 | 0.34 |
M2 | 2.91 - 1.06·month | 0.663 | 645.7 | 0.33 |
M12 | 2.88 + 0.07·LimeVol_ln + 0.60·Temp + 0.91·Moist + 0.15·LimeVol_ln:Moist | 0.649 | 658.5 | 0.32 |
M4 | 2.92 + 0.86·Moist | 0.407 | 803.8 | 0.16 |
M3 | 2.90 + 0.55·Temp | 0.158 | 902.6 | 0.11 |
M1 | 2.90 + 0.29·LimeVol_ln | 0.028 | 941.1 | 0.08 |
The best fitted model M11 includes all the studied variables and the interaction LimeVol × month. Tab. 4 shows the relative influence of the scaled variables included in model M11, among which month had the strongest (negative) influence. In the measured season the most intensive CO2 efflux was in May and the weakest in October under the influence of different factors. The modelled influence of soil moisture on CO2 efflux, together with doses of lime and season (for months May, July and September) is shown in Fig. 3. An increase in CO2 efflux with increasing soil moisture (increasing intercept of the curves) is evident, as well as the steeper dependence of CO2 efflux on liming at the beginning of season (May), where the biological activity was unlimited by moisture and temperature. Therefore, equal response of biological activity on amelioration during the whole season cannot be expected.
Tab. 4 - Scaled and unscaled parameter estimates for final model M11 together with their highest density intervals (HDI). HDI are Bayesian alternative to confidence intervals with either scaled or unscaled variables. (LimeVol_ln): dosage of dolomitic limestone transformed by natural logarithm; (month): month of measurements; (Moist): soil volumetric moisture.
Variable | Scaled variables (μ = 0; σ = 1) | Unscaled variables | ||||
---|---|---|---|---|---|---|
Estimate | Lower 95% HDI |
Upper 95% HDI |
Estimate | Lower 95% HDI |
Upper 95% HDI |
|
intercept | 2.92 | 2.81 | 3.02 | 3.980 | 3.409 | 4.559 |
LimeVol_ln (A) | 0.18 | 0.07 | 0.29 | 1.075 | 0.793 | 1.356 |
Month (B) | -0.85 | -0.92 | -0.78 | -0.322 | -0.388 | -0.256 |
Moist | 0.47 | 0.40 | 0.55 | 0.085 | 0.071 | 0.099 |
A × B interaction | -0.20 | -0.26 | -0.14 | -0.119 | -0.155 | -0.083 |
Fig. 3 - Graphic results of the Bayesian modelling. Model M11 values of CO2 efflux (R10, y-axis) in dependence on doses of lime (x-axis), in three categories of soil moisture (5, 12.5 and 20 vol. %) and three selected months (May, July and September). The grey belts denote 95 % confidence interval.
Soil microbial characteristics one year after liming
In the soil samples collected for soil respiration no carbonates were detected, hence all CO2 measured during the analysis comes from respiration. In one year, the effect of liming was evident within both the H and A horizons (Fig. 4a, Fig. 4b).
Fig. 4 - Microbial activity expressed by basal soil respiration (a, b), microbial biomass carbon (Cmic - c, d) and metabolic quotient (qCO2 - e, f) for the horizons H and A. Error bars represent the 95 % confidence intervals.
For the H horizon, apart from the 26 t ha-1 treatment, the values of soil respiration tended to increase depending on the increasing amounts of the ameliorant. Unlike the CO2 efflux measured from the soil surface, the 26 t ha-1 variant unexpectedly reached the lowest soil respiration level. The highest values were reached for the 9 t ha-1 limed area.
The lowest values in the A horizon were reached in the control subplot; compared to them, the different ameliorant doses caused an increase in soil respiration activity. However, the most heavily limed area showed significantly lower soil respiration than did the variants at 9 and 6 t ha-1.
The microbial biomass carbon (Cmic) amount was dependent on ameliorant doses in both horizons. With an increasing ameliorant dose, there is a gradual increase in the Cmic values in the humus horizon, followed by a significant decrease at the doses of 9 and 26 t ha-1, which are even lower than the control (Fig. 4c). In the A horizon, Cmic decreased with increasing the ameliorant dose (except for the variant 2 t ha-1 where the increase was non-significant) to the lowest values in the most heavily limed treatment. Therefore, in the control, immediately after the application of the 2 t ha-1 variant, it reaches the highest values (Fig. 4d).
In both horizons H and A, the soil respiration was significantly affected by Cmic (Pearson’s r = 0.721, p<0.05; and r = 0.517, p<0.05, respectively).
The metabolic quotient (qCO2) was found to be higher in the A-horizon than in the H-horizon (Fig. 4e, Fig. 4f). Moreover, all the treated subplots showed the increased qCO2 values in comparison with the control, except for the variant 26 t ha-1 in the H horizon (Fig. 4e), where a remarkable decrease in qCO2 was observed.
Discussion
The practice of limestone application may affect various aspects of soil properties and microbial activity. Andersson & Nilsson ([1]) demonstrated an increase in pH of the upper soil layers over a period of 12 or more years after the liming doses of 8.8 t ha-1. Conversely, lower pH values in mineral soil 4 years after liming at the dose of 3.25 t ha-1 are reported by Lundström et al. ([33]). The short-term effect of the soil pH modification is documented by Vavríček & Kučera ([50]) who reported a minimum impact on pH 15 years after the application dosage of 26 t ha-1. By contrast, McKie et al. ([36]) discussed the potential risks connected with the inappropriately high doses of limestone and consequent substantial pH changes in the humus layer. Soil buffering capacity was demonstrated in our study, when one year after liming there was only a slight pH increase in the two observed horizons (0.4 in H horizon, 0.1 in A horizon), especially when thick humus layers leading to moder and mor humus forms evolve - a situation typical of most of the Norway spruce stands ([35]).
Both methods applied in this study for determining soil respiration demonstrated the dependence of this parameter on the individual doses of ameliorant. In particular, basal soil respiration in organic horizons showed an increase when increasing the ameliorant dose ([38], [36]), except for the 26 t ha-1 treatment, which decreased to values even lower than the non-limed treatments. These results are inconsistent with the general expectations and it is possible that extreme ameliorant doses may have a different impact on soil biological properties compared to lower doses. However, the literature does not provide any examples of the inhibition of soil biological activity due to the application of extreme doses of the ameliorant. Moreover, our results from field measurements did not reveal any decrease in soil CO2 efflux under the highest limestone dosage. The possible cause is a significant alteration of the microbial community due to the substantial chemical impact (similar to the findings by [46]), leading to changes both in the total volume of microbial biomass and in the related microbial activity.
The respiration of the plant root system is also an important factor affecting the resulting soil CO2 efflux measured in the field ([41]). Comstedt et al. ([12]) revealed that respiration of the rhizosphere accounted for about 50% of the total soil CO2 efflux in a mature Norway spruce forest. According to Hanson et al. ([18]) the proportion of root respiration can be 10-90 % of total soil respiration, depending on vegetation type and season. However, the subdivision of the total soil respiration in root respiration (autotrophic) and soil microorganism respiration (heterotrophic - [28]) is still unclear ([8], [5]) and often yielded different results ([10]). Our study dealt with the total soil respiration (CO2 efflux) which characterises the response of the whole soil ecosystem in our study.
Although field measurement of CO2 efflux from soil under natural conditions allows for the respiratory activity dynamics to be monitored during the season, the effects of external environmental factors can also be incorporated. These factors substantially drive the temporal (from daily to seasonal) variability of soil CO2 efflux and include mainly soil temperature and moisture ([15]). In our study, the reduction of normalized CO2 efflux at 10 °C (R10) under soil moisture in September was observed, and the corresponding soil moisture was only 3.7 %. Under such conditions, soil CO2 efflux is almost independent on temperature, and soil moisture becomes the driving factor ([56]). The R10 decline in October can be associated with the decrease in microbial biomass and activity at the end of the season and with permanent low temperatures. Moreover, the increase in respiration during warmer months could lead to the significant interaction between R10 and temperature observed in this study ([43]).
The largest differences in field measurements were observed for the treatment 26 t ha-1 in May, when R10 increased by 97.6% compared to the control. However, this enormous difference decreased during the season, and by September, the increase was only 8.7% (Tab. 2). Lundström et al. ([33]) reports a 35% increase in soil respiration 14 years after liming the spruce stands at the dose of 8.75 t ha-1 compared to the control. In our case, the average R10 measured in May increased by 32.4% after 9 t ha-1 liming; the lowest increase in this variant was detected in August (7.8%).
In some cases, the expected liming effect on microbial soil activity is also reflected in the increase in the carbon microbial biomass Cmic ([3]) and metabolic quotient qCO2 ([32]). On the other hand, Priha & Smolander ([40]) reported no or only minimal changes in the amount of microbial biomass after liming. In our study, we found that the response on liming may differ between the individual horizons and also in dependence on limestone dosage. In the H horizon, Cmic tended to increase after liming, except for the dosage of 26 t ha-1. On the contrary, Cmic slightly decreased after liming the A horizon except for the dosages of 2 t ha-1. Therefore, it may be assumed that in the A horizon, liming acted as an inhibitor, to a certain extent, and the Cmic decreased as the dose of dolomitic limestone increased.
The effect of various liming doses on the metabolic quotient (qCO2) is expressed as an increase in qCO2 values according to increasing of ameliorant doses. This can be related to bacterial communities reacting to changes in soil chemistry ([6]). However, the exception is the 26 t ha-1 variant within the H horizon, which showed 33.2% decrease compared to the control. In this case, the extreme doses could lead to a temporal inhibition of microbial activity (qCO2).
Despite the current application of dolomitic limestone on forest soils, it may be discussed whether liming is a suitable practice to improve soil chemistry. Liming results in an increase of soil biological activity and hence in an increase in mineralization of organic layers. Although this is confirmed by the presented results, there are still unknown aspects regarding the consequences of liming. The following questions still need to be answered: (i) what is the proportion of autotrophic/heterotrophic respiration? (ii) are we able to measure how fast is the organic layer mineralization? (iii) what are the consequences of liming on long-term plant nutrition and soil water regime?
Conclusions
In general, liming increased soil respiration in our experimental forest stand, and this was confirmed by both in situ measurements and laboratory analyses. However, the effect of liming in situ was mitigated by environmental conditions such as drought or low temperatures.
The hypothesis that the effect of liming will increase with limestone dosage was fully confirmed only for in situ measurements of soil CO2 efflux and qCO2 in A horizon. For the basal respiration, a decrease for the dose of 26 t ha-1 was observed, with values in the H horizon even lower than in the control.
In the H horizon, liming increased the amount of microbial biomass, and a gradual inhibition of microbial development was observed at the highest ameliorant doses. In contrast, microbial biomass development in the A-horizon was inhibited after liming and this inhibition increased with the ameliorant dose.
Our results confirm that the dose of ameliorant commonly used in forest management (3 t ha-1) significantly increases the microbial activity of forest soil even one year after liming. This may accelerate the mineralization of soil organic material and lead to a decrease in soil quality. Future studies based on soil respiration measurement under controlled laboratory conditions will provide a better understanding of the potential response of soil microorganisms to changes in soil chemistry under optimal conditions.
List of abbreviations
(BasResp): basal respiration; (BS): base saturation; (CEC): cation exchange capacity; (Cmic): microbial biomass carbon; (Corg): organic (oxidizable) carbon content; (C/N): carbon-to-nitrogen ratio; (dw): dry weight; (HDI): highest density interval; (LimeVol): liming variant; (LOO-ajd R2): adjusted R2; (LOO IC): LOO information criterion; (Moist): soil moisture; (pH/H2O): active soil pH; (pH/KCl): exchangeable soil pH; (qCO2): metabolic quotient; (Q10): the proportional change in CO2 efflux in relation to a 10 °C increase in temperature; (R10): normalized soil CO2 efflux for 10 °C; (Rs): soil CO2 efflux; (sd): standard deviation; SD (ID): estimation of variability among random effects in scale of CO2 efflux; (Temp): soil temperature.
Acknowledgments
The work was supported by a Specific University Research Fund of the FFWT Mendel University in Brno (project No. LDF_VP_2016026), and projects QK1920328 (NAZV), Youth and Sports of Czech Republic within the National Sustainability Program I (NPU I), grant number LO1415.
References
Gscholar
CrossRef | Gscholar
Gscholar
Gscholar
Gscholar
Gscholar
Gscholar
Gscholar
Gscholar
CrossRef | Gscholar
Gscholar
Gscholar
Gscholar
Gscholar
Gscholar
Gscholar
Gscholar
Gscholar
Authors’ Info
Authors’ Affiliation
Aleš Kučera 0000-0002-3139-7675
Valerie Vranová 0000-0003-0931-2345
Mendel University in Brno, Faculty of Forestry and Wood Technology, Department of Geology and Pedology, Zemedelska 3, 61300 Brno (Czech Republic)
Global Change Research Institute, Academy of Sciences of the Czech Republic, Belidla 4a, 60300 Brno (Czech Republic)
Mendel University in Brno, Faculty of Forestry and Wood Technology, Department of Forest Botany, Dendrology and Geobiocoenology, Zemedelska 3, 61300 Brno (Czech Republic)
Corresponding author
Paper Info
Citation
Rosíková J, Darenova E, Kučera A, Volarík D, Vranová V (2019). Effect of different dolomitic limestone dosages on soil respiration in a mid-altitudinal Norway spruce stand. iForest 12: 357-365. - doi: 10.3832/ifor2894-012
Academic Editor
Rossella Guerrieri
Paper history
Received: Jun 11, 2018
Accepted: Apr 13, 2019
First online: Jul 05, 2019
Publication Date: Aug 31, 2019
Publication Time: 2.77 months
Copyright Information
© SISEF - The Italian Society of Silviculture and Forest Ecology 2019
Open Access
This article is distributed under the terms of the Creative Commons Attribution-Non Commercial 4.0 International (https://creativecommons.org/licenses/by-nc/4.0/), which permits unrestricted use, distribution, and reproduction in any medium, provided you give appropriate credit to the original author(s) and the source, provide a link to the Creative Commons license, and indicate if changes were made.
Web Metrics
Breakdown by View Type
Article Usage
Total Article Views: 35656
(from publication date up to now)
Breakdown by View Type
HTML Page Views: 31024
Abstract Page Views: 2092
PDF Downloads: 2047
Citation/Reference Downloads: 3
XML Downloads: 490
Web Metrics
Days since publication: 1849
Overall contacts: 35656
Avg. contacts per week: 134.99
Article Citations
Article citations are based on data periodically collected from the Clarivate Web of Science web site
(last update: Feb 2023)
Total number of cites (since 2019): 4
Average cites per year: 0.80
Publication Metrics
by Dimensions ©
Articles citing this article
List of the papers citing this article based on CrossRef Cited-by.
Related Contents
iForest Similar Articles
Research Articles
Soil CO2 efflux in uneven-aged and even-aged Norway spruce stands in southern Finland
vol. 11, pp. 705-712 (online: 06 November 2018)
Research Articles
Spatial heterogeneity of soil respiration in a seasonal rainforest with complex terrain
vol. 6, pp. 65-72 (online: 07 February 2013)
Research Articles
Soil respiration along an altitudinal gradient in a subalpine secondary forest in China
vol. 8, pp. 526-532 (online: 01 December 2014)
Research Articles
The manipulation of aboveground litter input affects soil CO2 efflux in a subtropical liquidambar forest in China
vol. 12, pp. 181-186 (online: 10 April 2019)
Review Papers
Separating soil respiration components with stable isotopes: natural abundance and labelling approaches
vol. 3, pp. 92-94 (online: 15 July 2010)
Research Articles
Different harvest intensity and soil CO2 efflux in sessile oak coppice forests
vol. 9, pp. 546-552 (online: 25 March 2016)
Research Articles
Seasonal dynamics of soil respiration and nitrification in three subtropical plantations in southern China
vol. 9, pp. 813-821 (online: 29 May 2016)
Research Articles
Short-time effect of harvesting methods on soil respiration dynamics in a beech forest in southern Mediterranean Italy
vol. 10, pp. 645-651 (online: 20 June 2017)
Research Articles
Soil respiration and carbon balance in a Moso bamboo (Phyllostachys heterocycla (Carr.) Mitford cv. Pubescens) forest in subtropical China
vol. 8, pp. 606-614 (online: 02 February 2015)
Research Articles
Thinning effects on soil and microbial respiration in a coppice-originated Carpinus betulus L. stand in Turkey
vol. 9, pp. 783-790 (online: 29 May 2016)
iForest Database Search
Search By Author
Search By Keyword
Google Scholar Search
Citing Articles
Search By Author
Search By Keywords
PubMed Search
Search By Author
Search By Keyword