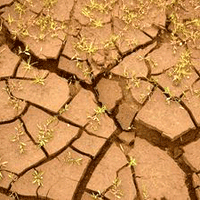
Separating soil respiration components with stable isotopes: natural abundance and labelling approaches
iForest - Biogeosciences and Forestry, Volume 3, Issue 4, Pages 92-94 (2010)
doi: https://doi.org/10.3832/ifor0541-003
Published: Jul 15, 2010 - Copyright © 2010 SISEF
Review Papers
Collection/Special Issue: NFZ Summer School 2009 - Birmensdorf (Switzerland)
Long-term ecosystem research: understanding the present to shape the future
Guest Editors: Marcus Schaub (WSL, Switzerland)
Abstract
Due to the potential of forest ecosystems contributing to CO2 increase as well as to climate change mitigation, forest-atmosphere CO2 exchange has been intensively studied over last decades. However, the contribution of individual components of belowground carbon pools is still poorly known. In particular, there is no unequivocal means to separate root respiration (autotrophic) from heterotrophic respiration by soil microflora and fauna. Most studies investigating soil respiration disturbed the soil and tried to exclude autrophic respiration. Here we review alternative non invasive methods to separate the two components. Those methods share the application of the stable carbon isotope 13C, using either local changes in natural abundance of 13CO2 or artificial labelling of trees with CO2 enriched or depleted in 13C. We conclude that the applicability of natural stable isotope methods is still limited in forest ecosystems because only in a few cases there are large enough differences in 13C among soil carbon pools (usually due to the earlier presence of a C4 canopy, which is seldom in forests). On the other hand, artificial labelling with CO2 either enriched or depleted in 13C is now in a widely used for partitioning soil respiration components. However, recent findings gave clear evidence that measurements of soil CO2 efflux can be substantially influenced by the return efflux of the abiotic label. Still, especially the combination of Free Air Carbon Enrichment (FACE) with dual δ13C and δ18O stable isotope approach has the potential to provide new answers on the response sensitivity of turnover dynamics of the largest belowground soil carbon storage to elevated temperature and CO2.
Keywords
Carbon stable isotopes, Labelling, Natural abundance, Autotrophic and heterotrophic soil respiration
Introduction
Soil respiration is the largest component of ecosystem respiration ([13]) and therefore a key element in the carbon source/sink role especially for forest ecosystems. The ongoing increase in both atmospheric temperature and CO2 content has an enhancing effect on the flux of CO2 from soil respiration ([8]). In spite of the increased soil respiration flux with elevated air temperature and CO2 level, the individual components of soil respiration [autotrophic root respiration, heterotrophic microbial respiration, and litter and soil organic matter (SOM) degradation] remain difficult to quantify. This is a challenging quest because all existing methods ([9]) bear their respective limitations. Promising new insights have been obtained from the use of stable carbon isotopes ([4], [11], [15]), where either: a) natural variability in the abundance of carbon isotopes in different compartments is examined; or b) a labelled 13CO2 signal is applied to compartments of the soil carbon cycle.
The aims of this mini review are firstly to present the main idea behind selected stable isotope approaches, namely: a) the method of natural 13C abundance; b) the method of isotopic labelling with 13C-depleted CO2; and (c) the method of isotopic labelling with 13C-enriched CO2. The second aim is to evaluate the methods’ applicability for the separation of the autotrophic and heterotrophic components of soil respiration in field conditions.
Method of natural abundance
The heavier stable isotope 13C is much less abundant than the lighter 12C.13C ≈ 1.10% of the total carbon ([5]). The isotopic carbon signature of any material is reported in the delta notation (δ13C) with per mil (‰) units, which refers to the ratio between the isotopic 13C/12C ratio (R) of the sample to the international reference V-PDB standard (eqn. 1):
where a higher δ13C stands for more heavy stable isotopes than the standard (enrichment) and a lower δ13C means less heavy stable isotopes than the standard (depletion). It is this ratio that is of particular interest for plant ecology as it is altered / fractionated to a varying degree by the different photosynthetic metabolisms of C3 and C4 plants. Because of differences in CO2 uptake and therefore different rates of diffusive fractionation, tissue of C3 plants will be isotopically depleted by about -29‰ ([9]), while C4 plant tissue shows a depletion of only -13‰ as compared to the standard. Numerous processes ([14]) including physiological status and meteorological conditions can influence these averaged values, but still these differences afford an excellent starting-point for separating soil respiration components by natural abundances of isotopes in the case where a C3 canopy follows a C4 crop (or vice versa) ([17]). Up to now, this is still an important limitation in the applicability of the natural abundance approach in forests, because C3 and C4 substitutions involving trees are rare. In the original forest without C3 and C4 substitutions, only small differences in the isotopic signal of soil respiration components were found in laboratory experiments. These differences might be diminished by the great spatial variability of soil efflux isotopic signal found in situ ([6]).
Formánek & Ambus ([6]) were able to distinguish δ13C of CO2 efflux from root-free mineral horizons, root-free humus layer and roots. The δ13C signatures alone were not sufficient to separate contributions of root respiration and SOM decomposition in the forest soil CO2 efflux measured in the field. The necessary estimate of root-free respiration profile δ13C signature was roughly calculated by bulk densities related contribution of each layer to the total CO2 efflux. Therefore, the estimation of 43% of root from total soil respiration could be realistic, but needs to be considered with caution, although it falls into the 29-59% range as reported by other carbon isotopic studies of temperate mixed deciduous forests ([3], [7]).
Method of isotopic labelling
There are two main labelling processes available: (i) labelling with 13C-depleted CO2 originating from fossil fuel combustion; (ii) labelling with 13C-enriched CO2 produced by nuclear processes.
The isotopic signature of air CO2 is -8.00‰ at present ([2]), but continuously moves towards more negative values because of fossil C combustion. CO2 derived from fossil carbon sources, i.e., mineral oil and coal, shows a depleted carbon isotopic signature of about -35.00‰ ([10]), which turns it in an ideal and inexpensive substrate for isotopic labelling of the soil carbon cycle under both field and enclosed conditions. There are many ongoing and already conducted isotopic labelling studies (e.g., [1]) with very promising results, but in the present paper we focus on one particular work by Lin et al. ([10]), because this study achieved clear partitioning of three forest soil respiration components (i.e., CO2 efflux from root, litter, and SOM). In this study, 4-year old Douglas-fir trees were grown under controlled conditions in terracosms with four different combinations of ambient and elevated CO2 concentrations and temperature. SOM and soil litter originated not from the present trees but from an old growth Douglas-fir forest. In both the elevated and ambient groups, a constant CO2 concentration was maintained by fumigating the terracosms with tank CO2. The tank CO2 fumigation resulted in newly formed plant material such as needles and roots with a δ13C of -35.77 ± 0.09‰ (± standard deviation) compared to an atmospheric CO2 δ13C of -8.55 ± 0.13‰ in the area where SOM as well as the organic litter of the soil in use had formed. With this approach alone, it would have not been possible to separate the three main components of soil respiration and to understand their individual responses to elevated temperature and CO2, because CO2 both from SOM and litter degradation carrying the same carbon isotopic signal. Lin et al. ([10]) found an elegant solution to this problem: they took advantage of the information in the δ18O oxygen isotopic signature in the soil respired CO2 molecule, which is in equilibrium with the respective signature in soil water. When soil water evaporation occurs, there is a diffusive fractionation of the water molecules carrying the two different stable oxygen isotopes 16O and 18O. This isotopic enrichment of 18O takes place only in the upper 10 cm of soil which allows a separation of CO2 originating from litter degradation and CO2 from SOM degradation according to their respective oxygen isotopic signature. The disadvantages of this particular approach apply as well for other labelling studies, i.e., FACE. In most cases, the task of maintaining constant conditions results in an elevated CO2 concentration. This means a major disturbance to the ecosystem carbon flux, because in the supply to microbial communities, the intricate relation between the supply of fresh energy abundant carbon compounds (leaf litter, rhizo-exudates and rhizo-deposition) and old, more nutrient prone soil organic carbon is altered ([12]). The need to label some components limits the applicability of the method under field conditions, even if a main focus is put on maintaining ambient conditions concerning the CO2 concentration.
A very interesting example of isotopic labelling with enriched 13CO2 is the study of Subke et al. ([16]) conducted on stand scale in a naturally regenerated Pinus silvestris forest in northern Sweden. Subke et al. ([16]) pulse-labeled (3h) the trees in two chambers (each 200 m³) with highly enriched 13CO2 and monitored the resulting 13CO2 soil efflux with a combination of deep, root free and surface collars over a period of 6 days. In this setup, the isotopic 13C signature of deep collars excluded plant derived CO2. The authors were able to separate the initial physical 13CO2 flux caused by the direct tracer diffusion from the atmosphere and the later occurring biological 13CO2 flux caused by root respiration of photosynthates (biotic label return). Approximately 2-3 days after pulse labelling, only in deep collars 13CO2 returned from the initial peak to near natural abundance, while in surface collars, 13CO2 likely from the respiration of labelled assimilates allocated below-ground, increased again and reached the maximum ca. 3.5-4 days after the pulse. In spite of the separation of autotrophic pulse-derived 13CO2 tracer from the difference between surface collars and deep collars, the experiment was primarily designed to separate abiotic and biotic tracer returns. However, the method has the potential for separating the autotrophic and heterotrophic part of soil CO2 efflux, if the experimental design allows plant photosynthesis to use only the labelled 13C-enriched CO2 source.
Conclusions
In this mini review, we compared the use of natural abundance and labelled carbon isotope methods in partitioning forest soil autotrophic and heterotrophic respiration components. The natural abundance carbon isotope method has the potential of separating forest soil respiration components, although its applicability is limited to situations where overplanting from C4 to C3 plants occurred. On forest sites with an exclusive C3 vegetation history, the individual soil components must be physically isolated (disturbing the carbon cycle). In addition, natural δ13C signatures show high spatial variation allowing only for a rough partitioning of soil respiration. The difficulty of relying on the existing C4 SOM can be overcome by the tank fumigation with 13C-depleted or 13C-enriched CO2. The newly formed organic material with depleted or enriched 13C signatures enables to distinguish the root autotrophic respiration from heterotrophic respiration of litter, roots and SOM formed before labelling with natural abundance 13CO2. However, also long-term fumigation experiments require an exclusion of the roots for a separation of the components of autotrophic soil respiration. A step further to non-invasive separation of autotrophic and heterotrophic components was achieved by the combination of long-term fumigation with dual δ13C and δ18O stable isotope tracers. Isotopic methods advanced from the disturbance of carbon cycle to a less invasive approach achieved by the combination of the former accomplishments. If a virtually undisturbed partitioning of the forest soil respiration components into fast and slow carbon turnover would be possible, it would enable better opportunities to study the effects of both elevated temperature and CO2 concentration onto the balance of long-term soil carbon storage.
Acknowledgements
We want to thank the whole team of the WSL summer school 2009, especially Dr. Marcus Schaub, not only for making this work possible but also for a warm welcome to Zurich and for an enlightening week. We are grateful to three reviewers for their valuable comments on an earlier version of this manuscript. We also want to express our gratitude to our supervisors and institutions for their support.
References
Authors’ Info
Paper Info
Citation
Braig E, Tupek B (2010). Separating soil respiration components with stable isotopes: natural abundance and labelling approaches. iForest 3: 92-94. - doi: 10.3832/ifor0541-003
Academic Editor
Marcus Schaub
Paper history
Received: May 25, 2010
Accepted: May 31, 2010
First online: Jul 15, 2010
Publication Date: Jul 15, 2010
Publication Time: 1.50 months
Copyright Information
© SISEF - The Italian Society of Silviculture and Forest Ecology 2010
Open Access
This article is distributed under the terms of the Creative Commons Attribution-Non Commercial 4.0 International (https://creativecommons.org/licenses/by-nc/4.0/), which permits unrestricted use, distribution, and reproduction in any medium, provided you give appropriate credit to the original author(s) and the source, provide a link to the Creative Commons license, and indicate if changes were made.
Web Metrics
Breakdown by View Type
Article Usage
Total Article Views: 50344
(from publication date up to now)
Breakdown by View Type
HTML Page Views: 42604
Abstract Page Views: 2456
PDF Downloads: 4386
Citation/Reference Downloads: 32
XML Downloads: 866
Web Metrics
Days since publication: 5126
Overall contacts: 50344
Avg. contacts per week: 68.75
Article Citations
Article citations are based on data periodically collected from the Clarivate Web of Science web site
(last update: Feb 2023)
Total number of cites (since 2010): 5
Average cites per year: 0.36
Publication Metrics
by Dimensions ©
Articles citing this article
List of the papers citing this article based on CrossRef Cited-by.
Related Contents
iForest Similar Articles
Research Articles
Soil respiration along an altitudinal gradient in a subalpine secondary forest in China
vol. 8, pp. 526-532 (online: 01 December 2014)
Research Articles
Spatial heterogeneity of soil respiration in a seasonal rainforest with complex terrain
vol. 6, pp. 65-72 (online: 07 February 2013)
Research Articles
Soil respiration and carbon balance in a Moso bamboo (Phyllostachys heterocycla (Carr.) Mitford cv. Pubescens) forest in subtropical China
vol. 8, pp. 606-614 (online: 02 February 2015)
Research Articles
Seasonal dynamics of soil respiration and nitrification in three subtropical plantations in southern China
vol. 9, pp. 813-821 (online: 29 May 2016)
Research Articles
Effect of different dolomitic limestone dosages on soil respiration in a mid-altitudinal Norway spruce stand
vol. 12, pp. 357-365 (online: 05 July 2019)
Research Articles
Short-time effect of harvesting methods on soil respiration dynamics in a beech forest in southern Mediterranean Italy
vol. 10, pp. 645-651 (online: 20 June 2017)
Research Articles
Thinning effects on soil and microbial respiration in a coppice-originated Carpinus betulus L. stand in Turkey
vol. 9, pp. 783-790 (online: 29 May 2016)
Research Articles
Comparison of soil CO2 emissions between short-rotation coppice poplar stands and arable lands
vol. 11, pp. 199-205 (online: 01 March 2018)
Research Articles
Soil stoichiometry modulates effects of shrub encroachment on soil carbon concentration and stock in a subalpine grassland
vol. 13, pp. 65-72 (online: 07 February 2020)
Research Articles
The manipulation of aboveground litter input affects soil CO2 efflux in a subtropical liquidambar forest in China
vol. 12, pp. 181-186 (online: 10 April 2019)
iForest Database Search
Search By Author
Search By Keyword
Google Scholar Search
Citing Articles
Search By Author
Search By Keywords
PubMed Search
Search By Author
Search By Keyword