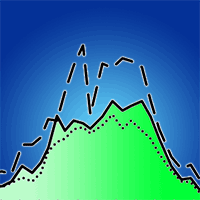
The manipulation of aboveground litter input affects soil CO2 efflux in a subtropical liquidambar forest in China
iForest - Biogeosciences and Forestry, Volume 12, Issue 2, Pages 181-186 (2019)
doi: https://doi.org/10.3832/ifor2812-012
Published: Apr 10, 2019 - Copyright © 2019 SISEF
Research Articles
Abstract
Litters on the forest floor represent an important organic carbon (C) sources from aboveground plants to the soil, which therefore have a significant influence on belowground processes such as soil respiration. In this study, dynamic property of soil respiration was investigated under aboveground litter manipulation treatments in a liquidambar forest in subtropical China. The purpose of this study was to examine the impacts of changing aboveground litter inputs on soil CO2 emission in forests. The litter manipulation included litter addition (LA), litter removal (LR) and litter control (LC) treatments. Each litter treatment had six replications. Soil respiration rates were measured using an infrared gas analyzer system (LI-COR 8100) with soil chambers. The results showed that mean soil respiration rates increased significantly in LA plots (mean ± SE: 2.21 ± 0.44 μmol m-2 s-1; P<0.05) and decreased slightly in LR plots (1.17 ± 0.16 μmol m-2 s-1) when compared to control plots (1.42 ± 0.20 μmol m-2 s-1). On average, LA treatment significantly increased annual soil respiration by about 56% (837.5 ± 165 gC m-2 year-1), while LR treatment decreased soil respiration by approximately 17% (443.1 ± 61.7 gC m-2 year-1) compared with the control (535.5 ± 75.7 gC m-2 year-1). The “priming effect” was a primary contributor to the increase of soil respiration in LA treatments and the reduction of soil CO2 efflux was mainly ascribed to the elimination of organic C sources in LR treatments. Soil temperature was the main factor affecting seasonal variation in soil respiration. Up to the 90% to 95% seasonal variation in soil respiration is explained by soil temperature within each of the litter treatments. Our study indicated that changes in litter inputs due to climate change and human practices would significantly affected soil CO2 emission and would subsequently affect C balance in subtropical forests.
Keywords
Soil CO2 Emission, Annual Litter Input, Deciduous Forests, Soil Temperature, Soil Water Contents, Subtropical China
Introduction
Soil respiration is commonly defined as the total CO2 efflux (FCO2) from the soil-litter surface and is one of the largest CO2 fluxes from land to the atmosphere ([11], [39]). It has been estimated that soil FCO2 is about 75 PgC year-1 ([26]), which is about ten times the flux of CO2 to the atmosphere contributed by the combustion of fossil fuels ([30]). Therefore, this flux represents a major component of the global carbon (C) cycle that can directly affect atmospheric CO2 concentration and thereby the global climate systems ([36], [23]).
Carbon dioxide emission from forest soils vary considerably, depending upon forest types ([26], [48], [43]), stand composition and structure ([16], [7]), growth and development stages ([18]), as well as litter amount on the floor ([50], [47]). Aboveground litterfall represents a major pathway of C from plant to the soil, and thus the litter amount accumulated on forest floor plays a critical role in maintaining forest production and regulating belowground processes such as soil respiration. Litter amount directly or indirectly affect soil respiration by regulating microclimatic conditions on the floor ([32]), modifying microbial community structure and function ([15], [42], [3]), changing the number of decomposer organisms ([10]), affecting decomposition rates ([17], [29]) and altering the amounts of available nutrients in the soil ([32]). As a consequence, changes in litter amount on soil surface might result in a significant alteration of soil respiration in forests ([4], [46]).
Elevated atmospheric CO2 concentration could stimulate plant growth and thereby increase litterfall production in forest ecosystems ([13], [33]). Such increases of aboveground litter are likely to lead to changes in soil microbial community structure and activity, which could constitute an important positive feedback to soil respiration process ([41], [32]). A number of researchers have investigated the responses of soil FCO2 process to the changes in litter amount on forest floor. Sayer et al. ([33]) reported that increased litter in tropical forests boosts the transfer of soil CO2 to the atmosphere. Zimmermann et al. ([50]) indicated that litter amount on soil surface made a large contribution to diurnal and annual patterns of soil respiration process in a tropical montane cloud forest. Our previous work has shown that when litter inputs were double, soil respiration increased about by 12% in a Masson pine and Camphor tree mixed forest. However, such effect of increasing soil FCO2 was not found both in a pure Camphor tree forest and in a pure Masson pine forest. In contrast, litter exclusion significantly reduced soil FCO2 rates by approximately 20-40% in these subtropical evergreen forests ([47]). Such variable results suggest that more studies are required to further understanding of the impacts of changing litter inputs on soil respiration in forests. It is a particular case for deciduous forests, because less study has been conducted in terms of litter-soil respiration relation in this forest type, despite deciduous forests are important components of subtropical forest communities ([24], [25], [5]).
Here, we conducted an aboveground litter input manipulation experiment to examine the impact of changes in litter inputs on soil respiration rates in liquidambar forests in the subtropical region of China. We hypothesized that litter manipulation treatments would affect soil FCO2 rates due to changes in carbon inputs in soil. The liquidambar forests are a common timber and green-garden deciduous forest type in southern China. The litter manipulation experiments were designed to address the following questions: (i) Do the changes in litter amount affect soil respiration rates in this deciduous forest? (ii) Do the litter manipulation treatments alter the seasonal patterns of soil FCO2 process? (iii) Do the relationships among soil respiration, soil temperature and moisture vary due to the change of litter inputs?
Materials and methods
Study site
The study was carried out at Hunan Botanical Garden in Changsha city, Hunan Province, China (113° 02′ - 113° 03′ E, 28° 06′ - 28° 07′ N). The study area is a typical moist subtropical zone with a mean annual temperature of 17.2 °C and mean temperatures of 4.7 and 29.4 °C in January and July, respectively. Annual precipitation is 1200-1700 mm and the average annual rainfall is 1422 mm, most of which occurring from April to August. Mean annual relative humidity is >80%. The frost-free period is 270-310 days per year. The garden covers about 140 ha, with elevation of 50-110 m a.s.l. and an average site slope of 5-15o. Soil is classified as a typical red earth developed from slate parent rock, which is equal to Alliti-Udic Ferrosols according to the World Reference Base for Soil Resources ([8]). Soil texture ranges from clay loam to sandy loam. pH on the topsoil (0-10 cm) was acidic with an average pH of 5.0. The concentration of organic carbon and nitrogen in the top 20 cm of the soil were 23.1% and 1.57%, respectively.
The liquidambar (Liquidambar formosana Hance) forests were planted in 1986 with an initial stand density of 2 × 2 m. When the current study started in 2013, the average height of the trees was 14.8 m, the average diameter at breast height 16.8 cm, the stand density 1100 trees per hectare and the stand crown density 0.9. Understorey consisted of Sassafeas tsumu Hemsl., Cinnamomum camphora, Symplocos caudata Wall. ex A. DC., Clerodendron cyrtophyllum Turcz, Nephrolepis auriculata Trimen, Lophantherum gracile Brengn., Miscanthus floridulus Warband and Phytolacca acinosa Roxb. T.
Experimental design
The experiment was a completely randomized design (CRD) initiated in May 2013. Three plots (each with 20 × 20 m in size) were chosen within the liquidambar forests in the study site. Six 3 × 4 m subplots were set up in each of the plots, where litter manipulation treatments were performed. Among these six subplots in a plot, two subplots were subject to one of the three litter manipulation treatments. Thus, there were six replications (six subplots) for each litter treatment in this study.
Three litter manipulation treatments were used in this study: litter removal (LR), litter addition (LA) and litter control (LC) treatments. The LR treatment was performed to remove all litter materials from the floor in the subplot at the beginning of the study. Then a 1-mm-mesh collection was installed about 0.8 m height above the forest floor on the subplot to prevent litter falling on the floor. All litter was collected and removed from the mesh collection twice a month. The LA treatment was performed by transferring and evenly distributing litter materials obtained from a LR subplot described above on LA subplots. The LA treatment was carried out twice a month. In the LC treatment, the natural status of litter on the floor was kept and the normal litter-fall process was allowed, excluding any removal or addition. The monthly litter-fall production varies greatly in the studied forests, ranging from about 180 to 1100 kg ha-1 year-1. Two PVC collars were established at each litter treatment subplot as the measurement points of soil respiration, and the mean value taken from these two PVC collars was considered to represent soil respiration from this subplot.
Soil FCO2 measurements
Soil FCO2 was measured on a biweekly basis from January 2013 to December 2014, using a portable infra-red gas analyzer (LI-COR 8100 with chamber (LI-COR Inc., Lincoln, Nebraska, USA). The measurements were delayed by 2-3 days when the designed time was a rainy day. At each measurement point, a PVC collar (11.7 cm in diameter, 4.4 cm in height) was installed into the soil, leaving 2.5 cm protruding above the soil surface. In order to minimize soil disturbance from the deployment of the flux chamber, PVC collars were placed into the soil at least one week prior to the first field measurement and remained in place through the course of the study. In each measurement date, soil FCO2 measurements were taken twice between 9:00 AM and 6:00 PM at each measurement points. The soil FCO2 value at each measurement point was the mean of the three sequential flux estimates at each sampling interval. Soil FCO2 was calculated from the change in CO2 concentration in the chamber during each flux measurements and the chamber height. Soil FCO2 is expressed as µmol m-2 s-1. The monthly data collection was timed to span a few days with similar weather patterns. A detailed description of soil FCO2 measurements is given in Yan et al. ([47], [48]).
Soil temperature and moisture measurements
Soil temperature (Tsoil) was measured simultaneously with soil FCO2, using a soil thermocouple probe (LI-COR 6000-09 TC) inserted in the soil to a depth of 5 cm below the soil surface. Soil water contents (Wsoil: volumetric soil water content, %) in the topsoil layer (0-5 cm) was measured using ECH2O Check (Decagon Devices Inc., Pullman, WA, USA) connected with EC-5 at the vicinity of PVC collars during soil FCO2 measurements.
Statistical analysis
Analysis of variance (ANOVA) was used to statistically assess the net effects of LR and LA on soil FCO2 rates, and plots temporal (month-to-month) variation on soil FCO2 rates, Tsoil, and Wsoil. Specifically, a mixed-design two-way repeated ANOVA was employed to test the effects of between litter treatments, measurement times, and treatment × time interaction on soil respiration. Prior to statistical analysis, the original soil FCO2 data were log-transformed to satisfy the normality and homoscedasticity assumptions of ANOVA. No log-transformation was performed on Tsoil and Wsoil data. Statistical analyses were conducted using the SAS statistical package ([31]).
Multiple regression analysis was employed to examine relationships between soil FCO2 and surrounding environmental factors (Tsoil and Wsoil). In order to accurately describe soil FCO2-Tsoil and soil FCO2-Wsoil relationships, data from litter treatment plots and control plots were separately used in the development of the models related to such relationships.
Results
Litter manipulation treatments significantly changed soil FCO2 rates (P<0.001) during this 2-year experiment. This effect was not significantly different between the measurement time of 2013 and 2014 (P>0.05). Specifically, litter addition (LA) treatments significantly increased soil FCO2 rates (P = 0.0017) while litter removal (LR) treatments significantly reduced soil FCO2 rates (P = 0.0199) compared to litter control (LC) treatments during the study period. On average, soil FCO2 rates increased by approximately 56% in LA plots (mean ± SE: 2.21 ± 0.44 μmol m-2 s-1) and reduced by about 17% in LR plots (1.17 ± 0.16 μmol m-2 s-1) when compared to the LC plots (1.42 ± 0.20 μmol m-2 s-1). Based on biweekly measurements, the cumulative annual mean soil FCO2 was 535.5 ± 75.7, 837.5 ± 165.0, and 443.1 ± 61.7 gC m-2 year-1 in LC, LA and LR plots, respectively.
The dynamic patterns of soil FCO2 rates were similar in the three litter manipulation treatment plots during the study period (Fig. 1). The soil FCO2 rates increased in the spring and reached their peaks during the summer times with a short falling-rising process in July. Soil respiration then decreased in the autumn and reached its minimum in the winter. The soil FCO2 rates varied substantially, ranging from 0.21-2.66, 0.36-4.58, and 0.27-2.38 μmol m-2 s-1 in LC, LA and LR plots, respectively (Fig. 1).
Fig. 1 - Dynamics of soil respiration in the liquidambar forests with different litter manipulation treatments from January 2013 to December 2014.
On average, the maximum mean monthly soil FCO2 rates appeared in June and minimum in February. There were likely two peaks in terms of annual soil FCO2 process in liquidambar forests: one appeared in the months of June and July and another in October. LA treatments significantly increased monthly mean soil FCO2 rate during the whole course of the study when compared to the control, while LR treatments reduced monthly mean soil FCO2 rates significantly for all months, except in the winter times when the difference was not significant between the treated plots and control plots (Fig. 2).
Fig. 2 - Monthly mean soil respiration of the liquidambar forests with various litter manipulation treatments during the period 2013-2014. Different letters above the bars in the same month indicate significant differences (P<0.05) among treatments after ANOVA.
Fig. 3 - Monthly mean soil temperature of the liquidambar forests with various litter manipulation treatments during the period 2013-2014.
Fig. 4 - The relationships between soil respiration rates and volumetric soil water content of the liquidambar forests with various litter manipulation treatments during the period of time of 2013 and 2014.
Fig. 5 - The relationships between soil respiration rates and soil temperature in the liquidambar forests with various litter manipulation treatments during the period of time of 2013 and 2014.
There were similar seasonal patterns in Tsoil in the three litter manipulation treatment plots, with the highest during summer and the lowest during the winter (Fig. 3). Litter manipulation treatments did not significantly change both Tsoil (P >0.05) and Wsoil (P >0.05). Soil FCO2 rates were strongly correlated with Tsoil (P = 0.0205 - Fig. 4), but not with Wsoil (P = 0.2630 - Fig. 5) in all studied plots. The relationship between soil FCO2 rates and Tsoil can be described using an exponential function as follows. In LA plots (eqn. 1):
where the corresponding Q10 was 2.52 (R2 = 0.903). In LR plots (eqn. 2):
where the corresponding Q10 was 2.46 (R2 = 0.966). In LC plots (eqn. 3):
where the corresponding Q10 was 2.57 (R2 = 0.945).
Discussion
Climate change includes correlated increases of atmospheric CO2 concentration and temperature on the Earth, which may increase forest productivity, including litterfall. But the consequences for soil FCO2 process remain poorly understood. The present experiment was designed to address how changes in aboveground litter inputs affect CO2 emission from the soil in deciduous liquidambar forests. Our results showed that soil FCO2 rates significantly increased by about 39% due to LA treatments and declined by 10% in LR plots when compared to the LC. These results were consistent with other previous findings ([35], [34]). For instance, Sulzman et al. ([38]) reported that doubling needle litter addition caused a 34% increase in the total C efflux in an old growth coniferous forest. Li et al. ([21]) found that soil CO2 efflux decreased considerably by 54% and 68% on average in a secondary forest and a plantation, respectively, compared to the control. Chemidlin Prevost-Boure et al. ([6]) carried out a litter manipulation treatment in a temperate deciduous forest and found that soil FCO2 significantly increased by 60-120% in LA treatment, but significantly decreased by 25-45% in a litter exclusion treatment when compared to the control. They attributed the effect of litter treatment on the variations in soil FCO2 to the alteration of soil activity mediated by the quantity of fresh litter. Litterfall and litter decomposition constitute a linkage between aboveground portion of the plants and the soil and are a key process in the regulation of C and nutrients cycling ([33], [45], [50]). The increase of soil respiration with LA might be attributed to several possible reasons. First, LA increased C and nutrients resources in the soil system and facilitated nutrients availability to soil organism uptake. Therefore, it would stimulate soil fauna and microbial activity, thereby increasing soil respiration processes ([9], [22], [20]). Second, the inputs of fresh organic C substrates due to LA increased the leaching of organic acids, often resulting in a “priming effect”, which led an increase in soil organic C mineralization and soil FCO2 rates ([19]). Third, LA modified microclimate environments on forest floor such as Tsoil and Wsoil by decreasing soil exposure, which indirectly caused a change in soil FCO2 ([32], [47]). In contrast, the reduction of soil FCO2 under LR treatments was due principally to the decline of nutrient inputs into the soil, which resulted in a suppression of food and nutrient supply to microbial communities ([40], [33]).
Interestingly, the alterations in soil respiration due to LA and LR treatments were not proportional in the current study. Indeed, the increase of soil FCO2 due to LA treatments (39% on average) were much higher than the decrease of soil respiration (10%) in LR treatments when compared to the control, meaning about 29% higher than expected by the LA treatment alone. In fact, such a disproportional response in soil respiration to changes in litter inputs was a common phenomenon in forests. Han et al. ([16]) investigated the effects of aboveground litter input manipulation on soil respiration in three subtropical successional forests and found that soil respiration decreased by 35% in LR plots and increased by 77% in LA sites on average. Sayer et al. ([33]) reported that soil respiration was on average 20% lower in the LR and 43% higher in the LA treatment compared to the controls in a lowland tropical forest. In our previous study, we found soil FCO2 rates were significantly decreased by about 39%, 24% and 22% in three evergreen forest types (Camphor tree forests, mixed forests, and Masson pine forest, respectively) due to LR treatments. However, LA treatments significantly increased soil CO2 by 12% in the mixed forests, but not in both Camphor tree and Masson pine forests when compared to the control ([47]). Although the mechanisms underlying the different magnitudes of the effects on soil respiration in LA and LR were not clear, a number of factors were likely involved in the results. It is well-known that microorganism communities and their activity play a central role in soil organic matter decomposition. Microbial communities might respond differently to the added fresh materials and to the removal of substrates. These responses may be the result of a different status of labile C availability for soil microorganisms ([44]), the utilization of different decomposed C resources ([14], [19]), the choice of specific degradable carbohydrates and water-soluble sugar components ([27]), the presence of a particular rhizosphere and the associated mycorrhizal fungi community ([9]) and the employment of linear and non-linear stratagems for microbes growth and activity ([21]).
Soil temperature (Tsoil) is widely recognized as a major abiotic factor related to soil respiration in a single geographic area, and the seasonal variations in soil respiration process is closely linked with seasonal patterns of soil temperature ([2]). In the current study, soil FCO2 rates were positively and exponentially correlated with Tsoil (Fig. 5) and these results are in agreement with many previous studies ([2], [37], [48]). Usually, the level of soil microorganisms’ activity increased with increasing Tsoil and the activity of soil enzymes was also enhanced under warming temperature, which caused a faster degradation of soil organic matter ([12], [1]). The temperature sensitivity (the Q10 value) of soil respiration was often described by an exponential equation and was defined as a factor by which soil FCO2 increase with increasing of temperature of 10 0C ([28]). Our Q10 values (2.46 - 2.57) in the three litter manipulation treatments were well within the range of published Q10 values widely across different forest types ([49]). It is worthy to point out that the litter manipulation experiment in the present study significantly altered soil FCO2 rates but did not affect soil temperature and Q10 values, indicating the priming effect occurred in the study sites. Similar observations had been previously reported in different forests ([38], [6]).
Conclusions
Climate change, forest management practices, and local human activities might result in changes of litter inputs and litter amounts on forest floor, which had substantial effects on belowground processes in forests. In this study, we examined how litter input changes affect soil respiration in a liquidambar forest in a subtropical region of China. LA treatments lead to a significant increase in soil FCO2, while LR treatments resulted in a significant reduction of soil respiration in the studied forests when compared to the control. The mechanisms of litter effects on soil respiration is very complex, but the increase or decrease in soil respiration are not likely determined by local soil microclimates (Tsoil and Wsoil) and temperature sensitivity (Q10) because litter manipulation did not significantly affected seasonal patterns of Tsoil and Q10 among the three litter input treatments. We proposed that the priming effect was a primary contributor to the increase of soil respiration in LA treatments and the reduction of soil FCO2 was mainly ascribed to the elimination of organic C sources in LR treatments. Our results suggest that changes in litter inputs due to climate change and human practices will significantly affect soil CO2 emission, thus affecting the C balance in subtropical forests.
Acknowledgements
This project was financially supported by Chinese Forestry Specific Research Grant for Public Benefits (no. 201404316), New Century Excellent Youth Program of the Ministry of Education of China (no. NCET-10-0151), “Bai Ren” Scholar Program of Hunan Province, and Central South University of Forestry and Technology’s grant (0842).
References
Gscholar
Gscholar
CrossRef | Gscholar
Authors’ Info
Authors’ Affiliation
Yuanying Peng
Cao Zhang
College of Life Science and Technology, Central South University of Forestry and Technology, Changsha, Hunan 410004 (China)
Xiaoyong Chen
National Engineering Laboratory for Applied Forest Ecological Technology in Southern China, Changsha, Hunan, 410004 (China)
College of Arts and Sciences, Lewis University, Romeoville, Illinois 60446 (USA)
College of Arts and Sciences, Governors State University, University Park, Illinois 60484 (USA)
Corresponding author
Paper Info
Citation
Yan W, Peng Y, Zhang C, Chen X (2019). The manipulation of aboveground litter input affects soil CO2 efflux in a subtropical liquidambar forest in China. iForest 12: 181-186. - doi: 10.3832/ifor2812-012
Academic Editor
Carlotta Ferrara
Paper history
Received: Apr 13, 2018
Accepted: Jan 24, 2019
First online: Apr 10, 2019
Publication Date: Apr 30, 2019
Publication Time: 2.53 months
Copyright Information
© SISEF - The Italian Society of Silviculture and Forest Ecology 2019
Open Access
This article is distributed under the terms of the Creative Commons Attribution-Non Commercial 4.0 International (https://creativecommons.org/licenses/by-nc/4.0/), which permits unrestricted use, distribution, and reproduction in any medium, provided you give appropriate credit to the original author(s) and the source, provide a link to the Creative Commons license, and indicate if changes were made.
Web Metrics
Breakdown by View Type
Article Usage
Total Article Views: 44295
(from publication date up to now)
Breakdown by View Type
HTML Page Views: 37143
Abstract Page Views: 3465
PDF Downloads: 2758
Citation/Reference Downloads: 3
XML Downloads: 926
Web Metrics
Days since publication: 2282
Overall contacts: 44295
Avg. contacts per week: 135.87
Article Citations
Article citations are based on data periodically collected from the Clarivate Web of Science web site
(last update: Mar 2025)
Total number of cites (since 2019): 8
Average cites per year: 1.14
Publication Metrics
by Dimensions ©
Articles citing this article
List of the papers citing this article based on CrossRef Cited-by.
Related Contents
iForest Similar Articles
Research Articles
Soil respiration along an altitudinal gradient in a subalpine secondary forest in China
vol. 8, pp. 526-532 (online: 01 December 2014)
Research Articles
Seasonal dynamics of soil respiration and nitrification in three subtropical plantations in southern China
vol. 9, pp. 813-821 (online: 29 May 2016)
Research Articles
Soil respiration and carbon balance in a Moso bamboo (Phyllostachys heterocycla (Carr.) Mitford cv. Pubescens) forest in subtropical China
vol. 8, pp. 606-614 (online: 02 February 2015)
Research Articles
Factors of soil CO2 emission in boreal forests: evidence from Central Siberia
vol. 16, pp. 86-94 (online: 19 March 2023)
Research Articles
Soil fauna communities and microbial activities response to litter and soil properties under degraded and restored forests of Hyrcania
vol. 14, pp. 490-498 (online: 11 November 2021)
Research Articles
Soil stoichiometry modulates effects of shrub encroachment on soil carbon concentration and stock in a subalpine grassland
vol. 13, pp. 65-72 (online: 07 February 2020)
Research Articles
Response of soil bacterial communities to nitrogen and phosphorus additions in an age-sequence of subtropical forests
vol. 14, pp. 71-79 (online: 11 February 2021)
Research Articles
Effect of exogenous nitrogen and phosphorus inputs on the microbe-soil interaction in the secondary Castanopsis sclerophylla forest in east China
vol. 11, pp. 794-801 (online: 14 December 2018)
Research Articles
Effects of arbuscular mycorrhizal fungi on microbial activity and nutrient release are sensitive to acid deposition during litter decomposition in a subtropical Cinnamomum camphora forest
vol. 16, pp. 314-324 (online: 13 November 2023)
Research Articles
Wood-soil interactions in soil bioengineering slope stabilization works
vol. 2, pp. 187-191 (online: 15 October 2009)
iForest Database Search
Search By Author
Search By Keyword
Google Scholar Search
Citing Articles
Search By Author
Search By Keywords
PubMed Search
Search By Author
Search By Keyword