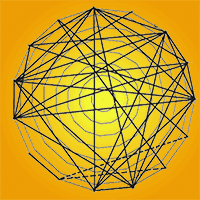
Responses of Taxus chinensis and Phoebe chekiangensis seedlings to controlled-release fertilizer in various formulations and application rates
iForest - Biogeosciences and Forestry, Volume 12, Issue 3, Pages 254-261 (2019)
doi: https://doi.org/10.3832/ifor2714-012
Published: May 03, 2019 - Copyright © 2019 SISEF
Research Articles
Abstract
Decline of species population, low natural regeneration, and heavy competition on field sites require the planting of large seedling stocks to restore Taxus chinensis and Phoebe chekiangensis in tropical China. In this study, we examined the effects of different formulations and application rates of controlled-release fertilizers (CRF) of nitrogen (N) and phosphorus (P) on nursery seedling growth and nutritional attributes. The objective was to determine optimum formulation (N:P2O5 ratio) and application rate to increase nutrient reserves of the seedlings before transplanting to the field. Four formulations (17-9-13 to 19-6-14 N-P2O5-K2O ratios) and four application rates (1.5 kg m-3 to 4.5 kg m-3) were used in a double-factors factorial design with 3 replications. The results showed that CRF formulation can affect nutritional attributes, while application rate modified seedling growth and nutritional attributes. The optimum seedling response occurred with the 17-6-16 formulation at the rate of 3.5kg m-3. These findings will guide nursery practice in the production of high-quality seedlings for optimum survival and growth in the field.
Keywords
Taxus chinensis, Phoebe chekiangensis, Controlled-Release Fertilizer, Formulation and Application Rate, Nutrient Utilization Efficiency
Introduction
Taxus chinensis [Pilg.] Rehd. (common name: Chinese yew) and Phoebe chekiangensis Shang (common name: Zhejiang Phoebe) are two slow-growing tree species native to tropical China ([7], [33], [43]). The main usage of these species is to harvest timber for manufacture of high end furniture, especially with Zhejiang Phoebe ([35], [43]). Since the 1990s, natural Chinese yew seeds have been targeted to extract toxoids for the treatment of cancer ([4]). The pharmaceutical use of Chinese yew has heavily impacted the natural populations with a sharp decrease of natural reserves ([33]), which stimulated the commercial development of this species. Under natural conditions, regeneration of these two species is very difficult ([43]), possibly due to constraints from intense seed dormancy, limited seed dispersal, susceptibility to seed rot, possible animal grazing, and the seed requirements for high moisture and low light during germination, seedling establishment, and early growth ([7], [43]). Global climate change may also disfavor the natural regeneration of these two species by higher temperatures and increasing drought ([40], [15], [21]). As a result, both species have been classified as “endangered” in the Red List Category by the International Union for Conservation of Nature (IUCN - [38], [33]). Seedling planting is therefore required for sustaining natural populations and restoring forests for timber harvest ([7], [43]), especially with the use of competitive seedlings ([35], [39]).
Container stock seedlings are superior to bare-root seedlings in terms of survival and growth ([41], [35]). The use of polymer-coated controlled-release fertilizer (CRF) at time of planting can promote seedling survival ([19], [27], [12]) and early growth ([17], [14], [19], [20], [27], [29], [3], [32], [12]). It is especially useful to employ CRF to seedlings in the face of harsh site conditions, such as intensive vegetation competition, poor soil nutrient availability, and heavy canopy shade ([8], [3], [31], [11]). These types of sites are especially hard to reforest with endangered tree species because these species typically grow slower than competitors and are more sensitive to field conditions ([35]).
CRF can also be used to enhance nursery seedling quality through nutrition-loading before transplanting ([18], [42], [22], [2]). In southern nurseries of China, one of the widely-used CRFs by nursery managers is Apex™ (J.R. Simplot Company, USA) which contains nitrogen (N), phosphorus (P, P2O5), and potassium (K, K2O) in a ratio of 18-8-8 and is typically applied at 2.5 kg m-3. This CRF application rate is often supplemented with the application of urea and ammonium phosphate to promote seedling growth. The current formulation and application rate with Apex for container seedlings is largely based on empirical observations, thus significant knowledge gaps exist in terms of the scientific employment of CRF with respect to inherent nutrient uptake and utilization by target seedlings.
There is supporting evidence that seedlings with enhanced nutrient reserves are expected to exhibit increased survival and growth after field transplanting ([28], [10], [36], [12], [23]), through improved nutrient uptake and enhanced photosynthesis ([18], [25], [22]). Among all the elements required by tree seedlings, N and P are the most limiting and therefore generally contained in CRF application ([27], [29], [22], [32], [11], [45], [12]). Both CRF formulation (namely, N:P2O5 ratio) and application rate influence seedling performances ([26], [39], [23]), but the suggested empirical formulation for optimum growth from previous reports is highly variable ([26], [44], [5]). In fact, little information is available regarding the optimum combination of CRF formulation and application rate.
In this study, two-year-old containerized seedlings of Chinese yew and Zhejiang Phoebe were treated for one growing season with different formulations and application rates of N and P using CRF. The objective was to determine the combination of CRF formulation and application rate for optimum seedling growth and nutritional attributes, including nutrient recovery and utilization. It was hypothesized that in relation to the standard application of Apex (i) formulations with higher N:P2O5 ratios would improve seedling growth and nutritional attributes; and (ii) greater application rates would improve seedling growth and nutritional attributes ([35]).
Materials and methods
Study site
The study was conducted in the experimental forest nursery (27° 38′ N, 119° 01′ E) at Qingyuan, Zhejiang, China. The study site belongs to the sub-tropical monsoon region, with elevation of 510 m a.s.l., mean annual temperature of 17.6 °C, mean annual precipitation of 1721.3 mm, and frost-free growing season of 245 days. The experiment was set up in an open steel greenhouse equipped with an automated irrigation system. The greenhouse was 2.2 m from ground to roof and had an average light transmittance around 50%.
Study material
In late April of 2013, Chinese yew and Zhejiang Phoebe seedlings (40 days old after germination) were transplanted into non-woven fabric containers of 5 cm diameter × 10 cm height that were filled with a growing substrate (peat and bran in 7:3 in volume) mixed with 2.5 kg Apex™ (J.R. Simplot Company, USA) per cubic meter. The substrate for CRF treatments was a mixture of peat, chaff, and mineral soil (40%:30%:30% in volume). The mixture had total N of 14.2 g kg-1, total P of 0.7 g kg-1, total potassium (K) of 2.7 g kg-1, bulk density of 0.3 g cm-3, and pH of 6.0. Using an automated irrigation system, seeded substrates were watered twice a day (10-15 minutes in the morning and evening) except for rainy days. In early December, the seedlings reached an average height of 43 cm and root-collar diameter (RCD) of 4.34 mm for Chinese yew, and an average height of 31 cm and RCD of 4.58 mm for Zhejiang Phoebe, based on random samples of 20 seedlings per species. The measurements of the 20 random samples indicated the total seedling N and P of 5.14 mg and 1.87 mg for Chinese yew and of 9.94 mg and 4.50 mg for Zhejiang Phoebe, respectively.
CRF treatment
On April 21, 2014, the two-year-old seedlings were transplanted into larger non-woven fabric pots (18 cm diameter × 20 cm height). CRF granules (Jinzhengda Co. Ltd., Shandong, China), which should provide a continuous supply of nutrients for 6-7 months at 20°C, were incorporated into the potting substrates. The CRF treatments followed a two-way factorial design with four levels of formulation and four levels of application rate. The four formulations (F) differed in concentrations of N:P2O5:K2O (Tab. 1) with four N:P2O5ratios ranging from 17:9 (F1, reference level) to 19:6 (F4, emulating summer application of additional urea and ammonium phosphate at nursery). The four application rates (A) ranged from 1.5 kg m-3 (A1) to 4.5 kg m-3 (A4), with the 2.5 kg m-3 (A2) being considered a standard rate in southeast China nurseries. The lowest application rate (A1) was designed as a baseline to obtain comparable seedling performance at reduced CRF, while the highest rate (A4) was to evaluate possible gain from enhanced fertilization. These treatments were replicated three times with thirty seedlings assigned to each combination of formulations and application rates. In total, 1440 seedlings (4 formulations × 4 rates × 3 replications × 30 seedlings) were tested for each species. Fans and curtains were used to increase air movement and reduce sunlight when temperatures were above 30 °C during the experiment.
Tab. 1 - Formulation (F) and application rate (A) of controlled-release fertilizers for Chinese yew and Phoebe chekiangensis seedlings.
Formulation treatment |
N-P2O5-K2O ratio |
Application treatment |
Application rate (kg m-3) |
N rate (g plant-1) |
P rate (g plant-1) |
---|---|---|---|---|---|
F1 | 17-9-13 | A1 | 1.5 | 1.30 | 0.69 |
A2 | 2.5 | 2.16 | 1.14 | ||
A3 | 3.5 | 3.03 | 1.60 | ||
A4 | 4.5 | 3.89 | 2.06 | ||
F2 | 16-7-16 | A1 | 1.5 | 1.30 | 0.69 |
A2 | 2.5 | 2.16 | 1.14 | ||
A3 | 3.5 | 3.03 | 1.60 | ||
A4 | 4.5 | 3.89 | 2.06 | ||
F3 | 17-6-16 | A1 | 1.5 | 1.30 | 0.69 |
A2 | 2.5 | 2.16 | 1.14 | ||
A3 | 3.5 | 3.03 | 1.60 | ||
A4 | 4.5 | 3.89 | 2.06 | ||
F4 | 19-6-14 | A1 | 1.5 | 1.30 | 0.69 |
A2 | 2.5 | 2.16 | 1.14 | ||
A3 | 3.5 | 3.03 | 1.60 | ||
A4 | 4.5 | 3.89 | 2.06 |
Seedling harvest, measurement and statistical analysis
Seedlings were destructively sampled in early December when current growth was complete. Ten randomly-selected seedlings from each treatment combination were measured for height (cm) and RCD (mm) and destructively sampled. For biomass determination, each of the sampled seedlings were washed, separated into leaves, stems and roots, and oven-dried for three days at 68 °C. The dried samples were then ground and sieved through 1.5 mm-mesh, and digested with H2SO4-H2O2 ([39]). N and P concentrations were determined using the Kjeldahl method ([39]) and ICP-OES (Vista-Mpx, Varian®, USA), respectively ([36]).
The Dickson’s seedling quality index (DQI) ([9]) was chosen to provide an integrated seedling quality assessment ([23] - eqn. 1):
where BAP is the total seedling biomass (g), SB is the shoot biomass (g), and RB is the root biomass (g).
Nutrient utilization index (UI) was used to evaluate photosynthetic production per unit leaf nutrient mass ([13] - eqn. 2):
where UI is N/P utilization index (NUI/PUI), and %Cleaf is percent leaf nutrient concentration.
Nutrient (N or P) recovery (Crecovery) was calculated using the following equation (eqn. 3):
where Cuptake is the seedling nutrient total (mg) at end of the treatments, Cinitial is the seedling nutrient total prior to CRF treatment (mg), and Cfertilizer is the total nutrient supply from CRF.
The utilization efficiency of nutrient uptake by mass input to root (EuU) was calculated for N (ENuU) and P (EPuU - eqn. 4):
Data analyses followed a two-way factorial design with four levels of CRF formulation and four application rates using the GLM procedure available in SAS (SAS Institute Inc., NC, USA). Measured response variables included height, RCD, total biomass, biomass allocations (component biomass to seedling biomass ratio), R/S ratio (root to stem biomass ratio), DQI (Dickson seedling quality index), N and P concentrations, and N and P uptake (seedling total) and allocations (ratios by biomass components). Means were compared with the post-hoc Tukey test when treatment effects were significant. When interactive effects of formulation by application rate were significant, data were re-analyzed with one-way ANOVA of sixteen treatments (four formulations by four application rates). Pearson’s correlation was calculated among response variables of seedling growth, biomass, nutrient uptake and utilizations.
Results
Growth and biomass
The CRF formulation only affected the stem biomass allocation of Chinese yew seedlings (Tab. 2), with values significantly lower in the F4 (mean ± SE: 38.88 ± 3.18 %) than in the F1 (42.20 ± 2.10 %), F2 (41.14 ± 2.63 %) or F3 (42.07 ± 3.46 %) treatment. Comparatively, the impact of application rate was greater, affecting most of the growth and biomass variables of the two species (Tab. 2). The highest growth and biomass were observed in the A3 treatment, which included height, RCD, seedling biomass and DQI of Chinese yew seedlings, and height and seedling biomass of Zhejiang Phoebe seedlings (Tab. 3). The CRF rate also affected stem and root biomass allocations and the root to shoot biomass ratio (R/S) of Zhejiang Phoebe seedlings, with the mean value significantly greater in the A1 treatment for root biomass allocation and R/S, and in the A4 treatment for stem biomass allocation (Tab. 3).
Tab. 2 - P values from ANOVA on the effects of different formulations (F) and application rates (A) of controlled-release fertilizers on the growth and nutrient attributes of Taxus chinensis and Phoebe chekiangensis seedlings. (RCD): root collar diameter; (Leaf biomass allocation): leaf biomass to seedling biomass ratio; (R/S): root to shoot biomass ratio; (DQI): Dickson’s seedling quality index; (Leaf N allocation): leaf total N to seedling total N ratio.
Variables | Taxus chinensis | Phoebe chekiangensis | ||||
---|---|---|---|---|---|---|
F | A | F × A | F | A | F × A | |
Height | 0.098 | <0.001 | 0.844 | 0.101 | <0.001 | 0.829 |
RCD | 0.077 | <0.001 | 0.165 | 0.589 | 0.088 | 0.292 |
Seedling biomass | 0.200 | <0.001 | 0.607 | 0.297 | <0.001 | 0.219 |
Leaf biomass allocation % | 0.884 | 0.966 | 0.243 | 0.128 | 0.064 | 0.550 |
Stem biomass allocation % | 0.030 | 0.274 | 0.578 | 0.736 | 0.001 | 0.068 |
Root biomass allocation % | 0.199 | 0.649 | 0.703 | 0.211 | <0.001 | 0.094 |
R/S | 0.141 | 0.274 | 0.897 | 0.169 | <0.001 | 0.140 |
DQI | 0.484 | <0.001 | 0.914 | 0.808 | 0.141 | 0.481 |
Leaf N concentration | 0.002 | <0.001 | <0.001 | <0.001 | <0.001 | 0.011 |
Stem N concentration | <0.001 | <0.001 | <0.001 | <0.001 | <0.001 | 0.002 |
Root N concentration | <0.001 | <0.001 | <0.001 | <0.001 | <0.001 | <0.001 |
Leaf P concentration | <0.001 | <0.001 | <0.001 | <0.001 | 0.026 | 0.024 |
Stem P concentration | <0.001 | <0.001 | <0.001 | <0.001 | <0.001 | <0.001 |
Root P concentration | <0.001 | 0.001 | <0.001 | <0.001 | <0.001 | 0.001 |
Seedling total N uptake | 0.359 | <0.001 | 0.047 | <0.001 | <0.001 | 0.423 |
Leaf N allocation % | <0.001 | 0.158 | 0.192 | 0.008 | 0.033 | 0.002 |
Stem N allocation % | <0.001 | 0.002 | <0.001 | 0.302 | <0.001 | 0.026 |
Root N allocation % | 0.007 | 0.083 | 0.625 | 0.034 | 0.040 | 0.005 |
Seedling total P uptake | 0.049 | <0.001 | 0.223 | 0.001 | 0.001 | 0.194 |
Leaf P allocation % | 0.118 | 0.127 | 0.009 | <0.001 | <0.001 | 0.018 |
Stem P allocation % | <0.001 | <0.001 | <0.001 | <0.001 | 0.177 | 0.038 |
Root P allocation % | <0.001 | 0.741 | 0.069 | 0.868 | <0.001 | 0.034 |
Tab. 3 - Mean comparisons of Taxus chinensis and Phoebe chekiangensis seedling growth and biomass by different application rates of controlled-release fertilizer treatments. (RCD): root collar diameter; (Leaf biomass allocation %): leaf biomass to seedling biomass ratio; (DQI): Dickson’s seedling quality index. Different letters indicate significant difference (p<0.05) after Tukey test.
Growth variables |
Application treatment |
n | Taxus chinensis | Phoebe chekiangensis | ||||
---|---|---|---|---|---|---|---|---|
Mean | SE | diff | Mean | SE | diff | |||
Seedling height (cm) | A1 | 12 | 87.81 | 4.11 | bc | 77.81 | 3.01 | c |
A2 | 12 | 89.84 | 4.04 | bc | 82.06 | 7.23 | bc | |
A3 | 12 | 104.08 | 4.88 | a | 92.12 | 4.24 | a | |
A4 | 12 | 92.6 | 4.43 | b | 87.33 | 3.72 | ab | |
RCD (mm) | A1 | 12 | 8.38 | 0.38 | b | 11.1 | 0.54 | a |
A2 | 12 | 8.81 | 0.47 | b | 11.71 | 0.68 | a | |
A3 | 12 | 9.59 | 0.61 | a | 11.78 | 0.65 | a | |
A4 | 12 | 8.84 | 0.42 | b | 11.67 | 0.65 | a | |
Seedling biomass (g) | A1 | 12 | 29.7 | 2.84 | c | 51.5 | 8.81 | c |
A2 | 12 | 35.3 | 4.28 | b | 60.9 | 7.24 | b | |
A3 | 12 | 43.9 | 6.16 | a | 67.7 | 7.53 | a | |
A4 | 12 | 33.5 | 4.13 | bc | 62.7 | 6.28 | ab | |
Leaf biomass allocation (%) | A1 | 12 | 28.1 | 2.35 | a | 30.6 | 2.25 | b |
A2 | 12 | 28.5 | 2.66 | a | 32.4 | 1.85 | a | |
A3 | 12 | 28.2 | 2.44 | a | 33.0 | 2.01 | a | |
A4 | 12 | 28.4 | 1.53 | a | 32.0 | 2.11 | ab | |
Stem biomass allocation (%) | A1 | 12 | 41.8 | 2.32 | a | 36.8 | 2.48 | c |
A2 | 12 | 41.6 | 1.58 | a | 38.8 | 2.13 | b | |
A3 | 12 | 39.9 | 4.05 | a | 38.6 | 2.42 | bc | |
A4 | 12 | 41.0 | 3.51 | a | 40.9 | 2.31 | a | |
Root biomass allocation (%) | A1 | 12 | 30.1 | 4.03 | a | 32.5 | 3.11 | a |
A2 | 12 | 30.0 | 3.06 | a | 28.8 | 2.38 | b | |
A3 | 12 | 31.9 | 4.99 | a | 28.3 | 1.58 | b | |
A4 | 12 | 30.5 | 4.47 | a | 27.1 | 2.46 | b | |
R/S | A1 | 12 | 0.44 | 0.08 | a | 0.48 | 0.07 | a |
A2 | 12 | 0.43 | 0.06 | a | 0.41 | 0.05 | b | |
A3 | 12 | 0.48 | 0.11 | a | 0.40 | 0.03 | b | |
A4 | 12 | 0.45 | 0.09 | a | 0.37 | 0.05 | b | |
DQI | A1 | 12 | 0.36 | 0.04 | b | 0.72 | 0.13 | a |
A2 | 12 | 0.41 | 0.05 | b | 0.86 | 0.19 | a | |
A3 | 12 | 0.52 | 0.08 | a | 0.85 | 0.10 | a | |
A4 | 12 | 0.4 | 0.05 | b | 0.8 | 0.10 | a |
Nutritional attributes
In both species, N and P concentrations and uptakes generally peaked at F3 (Tab. 2, Fig. 1). The effect of application rate varied with species, nutrient type, and biomass component (Fig. 1), as well as with N:P2O5 ratio (significant formulation by application rate interactions - Tab. 2). In comparison, the relationship of nutrient uptake with application rate was more consistent with the highest N and P uptake observed at the A3 treatment in both species.
Fig. 1 - N and P concentrations in leaves, stems and roots of Taxus chinensis and Phoebe chekiangensis seedlings in response to the interactive effects of controlled-release fertilizer formulation (F1-F4) and application rate (A1-A4).
More N and P were allocated to leaf and root than to stem (Fig. 2) in both species. Most allocation variables significantly changed with CRF formulation and application rate (Tab. 2), but the general trends differed by species, biomass components and nutrient types (Fig. 2). For example, root N and P allocations increased, while stem P allocation decreased with the increase of N:P2O5 ratio from F1 to F4 in Chinese yew seedlings (Fig. 2a, Fig. 2c). The same formulation change, however, reduced leaf N allocation and increased stem P allocation in Zhejiang Phoebe seedlings (Fig. 2b, Fig. 2d). Peak stem N allocation in Chinese yew and leaf N allocation in Zhejiang Phoebe occurred at F2. These trends, however, varied with application rate (significant treatment interactions - Tab. 2).
Fig. 2 - N and P allocation (%) to leaves, stems and roots of Taxus chinensis and Phoebe chekiangensis seedlings in response to the interactive effects of controlled-release fertilizer formulation (F1-F4) and application rate (A1-A4).
Nutrient recovery and utilization
Nutrient recovery decreased with the increase of application rate (A1 to A4, P< 0.01 in both species), with N recovery from 23.33% to 10.11% and P recovery 5.0% to 1.77% in Chinese yew seedlings and N recovery from 38.63% to 16.12% and P recovery 8.17% to 2.95% in Zhejiang Phoebe seedlings, but peaked at formulation 3 (F3) in both species (P<0.05 only in Zhejiang Phoebe). Interactive effect of formulation by application rate was significant only in Chinese yew where the decreasing pattern of N recovery with application rate was slightly different in F1 and F4.
Nutrient utilization index (UI) was generally highest in the A3 treatment for Chinese yew (NUI: 6.95 ± 0.86, P<0.01; PUI: 68.45 ± 9.04, P=0.003) and the A4 treatments for Zhejiang Phoebe (NUI: 16.08 ± 2.12, P=0.001; PUI: 187.09 ± 22.28, P<0.01). The NUI patterns with application rate also varied with formulation in both species (significant interactions).
In Chinese yew, the utilization efficiency of nutrient uptake (EuU) was only affected by application rate for N (P=0.032) and formulation for P (P=0.028), with the highest value observed at A4 (41.13) and F3 (4.27), respectively. In Zhejiang Phoebe, the highest ENuU and EPuU were recorded at F3 (43.02 and 4.23, respectively; P<0.01) and A3 orA2 (46.28 and 4.36, respectively; P<0.01) with some variations (significant interactions).
Association among performance variables
In Chinese yew seedlings, height, RCD and biomass were positively correlated with N uptake, P uptake, NUI and PUI, but not with leaf N concentration (Fig. 3a). Comparatively, leaf P concentration was correlated, not only with height and biomass, but also with DQI. Leaf N concentration was positively associated with ENuU and EPuU, both of which were negatively correlated with P recovery.
Fig. 3 - Pearson’s correlations among seedling growth and nutritional attributes of height, root-collar diameter (RCD), biomass, root to shoot biomass ratio (RS), Dickson seedling quality index (DQI), leaf N concentration (leaf %N), leaf P concentration (leaf %P), total N uptake, total P uptake, N recovery, P recovery, N and P utilization index (NUI and PUI), and utilization efficiency of N and P uptake (ENuU and EPuU). Solid lines indicate positive correlations and dashed lines indicate negative correlations.
In Zhejiang Phoebe seedlings, correlation was positive between height and leaf N + P concentrations, between N uptake and height + RCD + biomass, between R/S and N and P recoveries (Fig. 3b), between P uptake and RCD + biomass, between N + P uptakes and DQI, between biomass and NUI + PUI, and between EnuU+ EPuU and N + P uptakes, but negative in the relationship of R/S with height, leaf P concentration, ENuU and EPuU.
Discussion
Our first hypothesis was only partially confirmed by the findings of this study. The change of CRF formulation did not affect seedling size, biomass, and biomass allocation, possibly due to the similar influences of N and P on the growth of Chinese yew and Zhejiang Phoebe seedlings. Similar responses were found by Walker & Huntt ([34]) in Pinus jeffreyi seedlings and Oliet et al. ([26]) on the root growth of Pinus halepensis seedlings. Our results, however, are different from those by Oliet et al. ([26]) who found that high N ratio promoted shoot growth and therefore reduced R/S ratio in Pinus halepensis seedlings in nursery. Jacobs et al. ([20]) and Earnshaw et al. ([11]) also indicated that high N ratio in CRF can enhance biomass accumulation of transplanted seedlings in field conditions. Therefore, despite the significant effect of N input on tree seedling growth, a higher proportion of N component in CRF cannot be responsible for the higher quality for tree seedlings of all species.
Although seedling quality index by growth and biomass did not substantially change with formulation, most seedling nutrient variables changed significantly in response to the different formulation levels. Leaf and root nutrient concentrations respond to the change of CRF formulation and contribute heavily to the variation in shoot and root growth ([34], [26]). Hawkins ([13]) discerned that seedlings with high nutrient utilization index tend to produce more biomass. Similarly, in this study, most of growth variables and DQI were positively related with N and P uptakes and PUI (Fig. 3). Our results support the connections between seedling growth and DQI ([23]).
As expected, seedling height, RCD, biomass, DQI, and nutrient uptake all increased with application rate from A1 to A3, consistent with findings by many others ([18], [22], [42], [30], [12]). The decrease of growth parameters from A3 to A4 may result from toxic uptake ([26]). The decrease of nutrient recovery with application rate, as reported by Oliet et al. ([26]), indicates a greater leachate with increasing nutrient supply ([26]). With an increase of application rate, only Zhejiang Phoebe demonstrated a common response of plants to resource change where there is a reduction in the biomass allocation to roots ([35], [6], [16]), possibly due to greater seedling biomass, than Chinese yew, and therefore pot restriction on root development.
The stronger response of seedling growth to application rate than to the change of N:P2O5 ratio suggest that N and P elements are equally important to the growth of tree seedlings ([17], [22], [35], [23]), despite some possible influences of formulation on seedling nutrient uptake ([34], [26]). The equal significance of N and P to Chinese yew and Zhejiang Phoebe seedlings is also revealed from strong correlations of N and P concentrations and uptakes with growth and utilization efficiency of nutrient uptake (EuU). In both species, nutrient utilization efficiency was determined primarily by biomass production ([13]), as demonstrated from similar treatment response patterns with biomass and strong correlations of utilization index with growth, biomass, and DQI, but not with leaf N or P concentrations.
Greater nutrient allocations indicate that leaves and roots are the main nutrient storage of the plants and contribute significantly to new shoot and root growth ([34], [26]), as also shown by the positive growth correlation with leaf N and P concentrations ([26]) and uptakes (Fig. 3). Likely, the allocation to leaves would have increased in an earlier season of the year as winter hardening (December) would promote nutrient allocation to stem for winter storage and cold resistance ([45], [23]).
Substrate property is critical for production of high-quality container seedlings through modified nutrient uptake and utilization. Our study showed a higher nutrient utilization (NUI>4.0 and PUI>50) for both Taxus chinensis and Phoebe chekiangensis relative to the values reported previously, e.g., 1.3-1.8 ([13]), 1.5-2.1 ([23]), and 1.72-4.74 ([24]) for NUI, and PUI < 40 ([23], [24]). These differences likely result from the differences in potting mixtures that are generally mixed by peat and perlite in previous studies. In our study, the mixing chaff and soil with peat has apparently increased total N, compared to the potting mixtures used by others, e.g., Pinus contorta (1.2 g kg-1 - [1]) and Larix olgensis (5.7 g kg-1 - [37]). The high N and P would favor root development and hence help nutrient uptake, utilization, and recovery (10-38% and 3-9%, respectively) of the two subtropical seedlings, compared to the nutrient recovery values reported by others, e.g., 17-28% in Pinus contorta ([1]) and 30-56% in Larix olgensis ([37]). Therefore, the substrate used in this study is appropriate and unlikely has undesired impacts on seedlings.
Conclusions
The increase of N:P2O5ratio in CRF formulation did not affect seedlings growth, suggesting that N and P are equally important to Chinese yew and Zhejiang Phoebe seedlings. The optimum nutritional attributes generally occurred at formulation F3 (17-6-16 for N-P2O5-K2O), and optimum growth at application rate A3 (3.5 kg m-3). The higher N:P2O5 ratio (F4, 19-6-14 for N-P2O5-K2O) reduced nutrient recovery, utilization index, and utilization efficiency of uptake, possibly due to P limitation, while higher application rate (A4, 4.5 kg m-3) may have resulted in toxic effects on growth. Different interactive effects of formulation by application rate on nutritional attributes indicate a lack of consistent optimum treatment combination for different nutritional attributes. These findings need to be confirmed through connection of seedling growth and nutrient parameters with field performance.
Acknowledgements
Thank Rongzhou Man and MyaRice, both from the Ontario Forest Research Institute in Canada, for their polishing work and their suggestions for the paper. This study was supported by the “Forestry Science and Technology Cooperation Project between Zhejiang Province and Chinese Academy of Forestry” (2017SY19), and Special funds for the basic scientific research service of the central public welfare research institute (CAFYBB2018GC003), and “Zhejiang Science and Technology Major Program on Agricultural New Variety Breeding” (2016C02056-3).
References
Gscholar
Gscholar
CrossRef | Gscholar
CrossRef | Gscholar
CrossRef | Gscholar
Online | Gscholar
Gscholar
CrossRef | Gscholar
Gscholar
Authors’ Info
Authors’ Affiliation
Zhichun Zhou
Research Institute of Subtropical Forestry, Chinese Academy of Forestry; Zhejiang Provincial Key Laboratory of Tree Breeding, 311400, Hangzhou (China)
Dongbei Zhang
Xiaolin Wu
Experimental Forest Farm of Qingyuan County, Zhejiang Province, Qingyuan 323800, Zhejiang (China)
Corresponding author
Paper Info
Citation
Chu X, Wang X, Zhang D, Wu X, Zhou Z (2019). Responses of Taxus chinensis and Phoebe chekiangensis seedlings to controlled-release fertilizer in various formulations and application rates. iForest 12: 254-261. - doi: 10.3832/ifor2714-012
Academic Editor
Gianfranco Minotta
Paper history
Received: Dec 20, 2017
Accepted: Feb 01, 2019
First online: May 03, 2019
Publication Date: Jun 30, 2019
Publication Time: 3.03 months
Copyright Information
© SISEF - The Italian Society of Silviculture and Forest Ecology 2019
Open Access
This article is distributed under the terms of the Creative Commons Attribution-Non Commercial 4.0 International (https://creativecommons.org/licenses/by-nc/4.0/), which permits unrestricted use, distribution, and reproduction in any medium, provided you give appropriate credit to the original author(s) and the source, provide a link to the Creative Commons license, and indicate if changes were made.
Web Metrics
Breakdown by View Type
Article Usage
Total Article Views: 37290
(from publication date up to now)
Breakdown by View Type
HTML Page Views: 32605
Abstract Page Views: 1750
PDF Downloads: 1986
Citation/Reference Downloads: 3
XML Downloads: 946
Web Metrics
Days since publication: 1912
Overall contacts: 37290
Avg. contacts per week: 136.52
Article Citations
Article citations are based on data periodically collected from the Clarivate Web of Science web site
(last update: Feb 2023)
Total number of cites (since 2019): 8
Average cites per year: 1.60
Publication Metrics
by Dimensions ©
Articles citing this article
List of the papers citing this article based on CrossRef Cited-by.
Related Contents
iForest Similar Articles
Research Articles
Potential for utilization of wood ash on coastal arenosols with limited buffer capacity in KwaZulu-Natal and its effect on eucalypt stand nutrition and growth
vol. 10, pp. 180-188 (online: 19 November 2016)
Research Articles
Effects of stand age on litter quality, decomposition rate and nutrient release of Kazdagi fir (Abies nordmanniana subsp. equi-trojani)
vol. 13, pp. 396-403 (online: 03 September 2020)
Research Articles
Controlled-release fertilizers combined with Pseudomonas fluorescens rhizobacteria inoculum improve growth in Pinus halepensis seedlings
vol. 8, pp. 12-18 (online: 12 May 2014)
Research Articles
Energy production of poplar clones and their energy use efficiency
vol. 7, pp. 150-155 (online: 23 January 2014)
Research Articles
The responses of soil microbial community and enzyme activities of Phoebe zhennan cultivated under different soil moisture conditions to phosphorus addition
vol. 11, pp. 751-756 (online: 15 November 2018)
Research Articles
Changes in moisture exclusion efficiency and crystallinity of thermally modified wood with aging
vol. 12, pp. 92-97 (online: 24 January 2019)
Research Articles
Contrasted growth response of hybrid larch (Larix × marschlinsii), jack pine (Pinus banksiana) and white spruce (Picea glauca) to wood ash application in northwestern Quebec, Canada
vol. 14, pp. 155-165 (online: 06 April 2021)
Research Articles
Carbohydrate metabolism during new root growth in transplanted Larix olgensis seedlings: post-transplant response to nursery-applied inorganic fertilizer and organic amendment
vol. 10, pp. 15-22 (online: 22 September 2016)
Research Articles
Nursery fertilization affected field performance and nutrient resorption of Populus tomentosa Carr. ploidy levels
vol. 15, pp. 16-23 (online: 24 January 2022)
Research Articles
Wood production and nutritional status of Pinus taeda L. in response to fertilization and liming: a meta-analysis of the Americas
vol. 16, pp. 195-201 (online: 25 July 2023)
iForest Database Search
Search By Author
Search By Keyword
Google Scholar Search
Citing Articles
Search By Author
Search By Keywords
PubMed Search
Search By Author
Search By Keyword