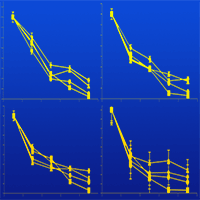
Effects of stand age on litter quality, decomposition rate and nutrient release of Kazdagi fir (Abies nordmanniana subsp. equi-trojani)
iForest - Biogeosciences and Forestry, Volume 13, Issue 5, Pages 396-403 (2020)
doi: https://doi.org/10.3832/ifor3306-013
Published: Sep 03, 2020 - Copyright © 2020 SISEF
Research Articles
Abstract
The influence of stand age on litter quality, decomposition rate and nutrient release was examined in pure stands of Kazdagi fir (Abies nordmanniana subsp. equi-trojani [Steven] Spach) differing in age (Fir38, Fir60, Fir90 and Fir100 years). The needle litters were collected and analysed for initial total carbon, cellulose, hemicellulose, lignin and nutrient concentrations (N, P, K, Ca, S, Mg, Mn and Fe). Initial litter quality parameters varied significantly among the four stand age classes. The Fir60 and Fir100 stands had higher total C than the Fir38 and Fir90 stands, while the Fir38 and Fir100 stands had higher N than the Fir60 and Fir90 stands. Mean cellulose and hemicellulose concentrations were highest in the Fir90 stand, while mean lignin concentration was highest in the Fir38 stand. Fir90 stand showed the highest ratios of C/N and Lignin/N. In general, the older fir stands showed higher Ca, Mg and K concentrations and lower P and S concentrations than the younger stands. The litter, however, showed higher a Mn concentration under the Fir60. Mean Fe concentration was highest under the Fir38 stand and lowest under the Fir60 stand. Litter decomposition was studied in the field using the litterbag technique. The litterbags were placed on the soil under each stand age class and sampled every 6 months for 2 years. The interaction of stand age and time on the mass loss was significant (p<0.01). The repeated measures ANOVA showed that the main effect of time on the mass loss was also significant (p<0.001). Needle litters under Fir100 and Fir60 stands decomposed faster than the needle litters under Fir90 and Fir38 stands. The calculated times required for 50% mass loss were higher under Fir38 (1.35 y) and Fir90 (1.27 y) stands than under Fir100 (1.05 y) and Fir60 (1.06 y) stands. The litters in Fir38 and Fir90 stands need approximately 4 years for 95% mass loss compared to the litters in Fir60 and Fir100 stands, which need 3 years. In general, Ca, Mg and S concentrations increased over time, whereas K and Mn decreased. These results illustrate that stand age is a key factor to be considered when studying litter decomposition dynamics.
Keywords
Litter Quality, Stand Age, Litter Decomposition, Nutrient Release, Fir
Introduction
Plant litter decomposition has long been recognized as an essential process for nutrient cycling and organic matter turnover within ecosystems ([23], [32]). Decomposition rate is mainly affected by climate (temperature and precipitation) and by litter quality (carbon/nitrogen [C/N] ratio, lignin, N and lignin/N ratio - [23], [44], [46]). In general, climate factors govern decay rates at broad scales, whereas litter quality plays an important role in controlling decay rates at small scales ([17], [47]). Most of the studies on the topic investigated the influence on decay rates of either interspecific differences in litter quality within systems or differences among systems using the same litter ([20], [8], [26], [29]). Moreover, considerable intraspecific variation in litter quality has been reported as related to factors such as tree canopy structure ([47]), soil characteristics ([43], [22], [48]), topography ([49]) and hydrology ([14]). The differences in litter quality within tree species may also reflect both genotypic and phenotypic variations resulting from biotic and/or abiotic interactions. However, a considerable variation in litter traits under different tree genotypes has also been observed. For example, polyphenol concentrations in leaf and fine root litter vary inherently among four distinct populations of Metrosideros polymorpha, the dominant tree species in Hawaiian montane forests ([22]).
While environmental heterogeneity and genetic variability have received some attention as sources of intraspecific litter quality and decay rate variability ([3]), the role of stand age has been poorly addressed ([26], [60]). Changes in photosynthesis, mineral nutrition, respiration, C allocation and hydrological function during stand development were reviewed in detail by Ryan et al. ([42]). Some authors proposed that the quality and quantity of soil organic matter and nutrient turnover can be influenced by forest stand age and the related changes in community structure and composition ([26]). Moreover, litter decomposition can be affected by microclimatic changes due to variations in solar radiation, wind speed or rainfall interception related to the canopy structure ([16], [64]). Relative humidity, in particular, can increase in the course of secondary forest succession and promote decomposition ([35], [62], [61]). However, there is evidence that plants can show considerable intraspecific variations in litter quality related to forest stand age ([54], [53]). For example, Trap et al. ([60]) showed that for pure beech (Fagus sylvatica) stands with different ages (15, 65, 95 and 130 years), forest age is an important driver of litter quality variation. Notable results included decreasing Mg, N and K concentrations during stand development and varying hemicellulose, cellulose and lignin content.
In this study, we tested the hypothesis that litter quality of Kazdagi fir (Abies nordmanniana subsp. equi-trojani [Steven] Spach) changes during its 100-year life span while controlling for climate and soil conditions. Moreover, we hypothesised that variations in litter quality parameters affect litter decomposition and nutrient release.
Material and methods
Study site description and sampling
The study was conducted in pure fir-dominated stands around the town of Inebolu, which is located in the north part of Kastamonu Province in northwest Turkey (41° 51′ 23″ N, 33° 45′ 36″ E - Fig. 1). Study site elevation is 1030 m a.s.l. The aspect is northeast (NE) with a mean slope of 25%. The study site has a characteristic Black Sea climate with warm and rainy winters and hot but rainy summers. According to weather data for 1960-2015 (Inebolu Meteorology Station, 64 m a.s.l.), mean annual precipitation is 1551 mm, ranging from 90.3 mm in July to 185.9 mm in December. The mean annual temperature is 9.3 °C, reaching highs in August (26.6 °C) and lows in February (2.7 °C). The parent rock of the study site is neritic limestone and schists covered with sandy loam and clay. According to the FAO forest soil classification system, the soil of the fir stand is brown forest soil, and the humus layers were mull.
The fir is an important tree species in Turkey. Here this genus is represented by five taxa: (i) Abies nordmanniana subsp. nordmanniana Spach; (ii) Abies nordmanniana subsp. bornmuelleriana Maltfelt; (iii) Abies cilicica subsp. isaurica Coode & Cullen; (iv) Abies cilicica subsp. cilicica Carr.; and (v) Abies nordmanniana subsp. equi-trojani Coode & Cullen. These taxa are distributed over 584.781 hectares, which accounts for 2.62% of the overall forest coverage. As an endemic taxon, Kazdagi fir (Abies nordmanniana subsp. equi-trojani) is distributed in the Central and Western Black Sea Region and South Marmara Region in Turkey ([36]). Selective logging of the fir forests, which are natural forests in the region, is the main management type in the region.
Fir stands were classified into the following age classes according to average tree age: 38 (Fir38), 60 (Fir60), 90 (Fir90) and 100 (Fir100) years old. For each stand age class, three subplots (20 × 20 m = 400 m2) were chosen. Freshly fallen fir-needle litters were collected from a total of twelve subplots (3 replicates × 4 age classes = 12 subplots). Litters showed no visible signs of discolouration or fungal colonization. The needle litters were air-dried in the laboratory and then oven-dried at 40 °C for 48 h. The oven-dried samples were then stored in plastic bags at 6 °C until the analyses.
Stand characteristics (height, diameter and canopy closure) were measured in each subplot. Measurements of diameter at breast height (DBH) and tree height were conducted on three sample trees in each stand age class. DBH was measured using a diameter tape. Trees were cored at breast height to determine tree age using the dendrochronological approach. Tree heights were measured with a Blume-Leiss clinometer.
Soil samples were taken under the same trees from which the fir needle litter was collected. Soil samples were collected in an area of 0.5 × 0.5 m2 at a distance of 2 m from the base of the trunk and at a depth of 0-20 cm. The moist field samples were sieved (< 2 mm) to remove stones, roots and macro-fauna and bulked to give a single representative soil sample for each age class. Stand and soil characteristics are shown in Tab. 1.
Tab. 1 - Stand and soil characteristics for per stand age classes. Means with different alphabets in rows indicate significant (p<0.001) difference between the stand age classes.
Variable | Stand age classes (years) | |||
---|---|---|---|---|
Fir38 | Fir60 | Fir90 | Fir100 | |
Mean diameter (cm) | 30.8 ± 1.4 a | 41.5 ± 3.9 b | 47.0 ± 3.0 c | 48.0 ± 2.4 c |
Mean height (m) | 24 ± 2.0 a | 35 ± 1.0 b | 41 ± 2.0 c | 42 ± 2.0 c |
Canopy closure (%) | 41-70 | 41-70 | 71-100 | 71-100 |
PH (H2O) | 5.16 ± 0.14 a | 5.15 ± 0.30 a | 5.99 ± 0.37 b | 5.00 ± 0.23 a |
Sand (%) | 32 ± 3.0 b | 8 ± 1.0 a | 84 ± 3.0 c | 76 ± 3.0 c |
Clay (%) | 42 ± 3.05 b | 54 ± 2.0 c | 8 ± 1.0 a | 9 ± 1.0 a |
Silt (%) | 26 ± 0.58 c | 38 ± 3.0 d | 8 ± 4.0 a | 15 ± 2.0 b |
Soil bulk density (g cm-3) | 1.12 ± 0.08 a | 1.16 ± 0.28 a | 1.04 ± 0.35 a | 1.20 ± 0.35 a |
Needle litter and soil analysis
The stored needle litters were oven-dried at 85 °C, ground in a laboratory mill to a mesh fraction less than 1 mm and analysed for organic C, total N, P, K, Ca, Mg, S, Mn, Fe, ADF (acid detergent fibre), lignin and cellulose concentrations.
Organic C and N concentrations were measured using a CNH-S elementary analyser (EA 3000® v.3.0 single, Eurovector, Milan, Italy) according to the dry combustion method. Nutrient concentrations (P, K, Ca, Mg, S, Mn, Fe) in the needle litters were analysed using an energy dispersive X-ray Fluorescence Spectrometer (EDXRF Xepos II®, Spectro-Analytical Instruments GmbH, Kleve, Germany). Certified soil reference material (NIST SRM 2709) was used to assess the accuracy of the EDXRF analyses.
Acid detergent fibre (ADF), a-cellulose and lignin were determined using the ADF-sulphuric lignin method by Rowland & Roberts ([41]). ADF was calculated as the mass loss after heating a 0.5 g tared sample for 1 h with acidified cetryltrimethyl ammonium bromide and filtering the suspension through a tared glass sinter, followed by drying and reweighing. Cellulose was calculated as the mass loss after acidification of the ADF with 72% H2SO4, and lignin was calculated from the residual mass of filtrate after ignition at 550 °C for 2 h.
Soil pH (H2O) was measured in a 1:2.5 mixture of deionized water and soil using a glass calomel electrode (pH 5 Series meter, LaMotte, Chestertown, MSD, USA) after equilibration for 1 h in solution ([27]). Soil moisture content on fresh soil samples was calculated by weight loss after drying approximately 10 g of soil for 24 h at 105 °C ([4]). Bulk density was determined by weight loss after drying the undisturbed soil cores. Soil texture (sand, silt and clay) was determined using the hydrometer method of Bouyoucos ([10]). All analyses were performed in triplicate.
Needle litter decomposition
The litterbag method ([9]) was used to measure the decomposition rates of the different needle litters in the field. Litterbags (20 × 20 cm) were made of 0.1-mm nylon with a mesh size of 1.5 mm to allow for inclusion of mesofauna but exclusion of macrofaunal detritivores.
Each bag was filled with 5 g of needle litter that was air-dried at room temperature until a constant weight was reached. Sub-samples of the needle litter were taken to determine the moisture content by drying at 85 °C. For each age class, the litterbags were prepared and placed in the original site where the needle litter was collected.
The number of needle litterbags used in the field experiment was 80 (4 stand age classes × 4 removal dates × 5 replicates). The needle litterbags were numbered and fixed to the ground of the corresponding site with metal pegs. Five litterbags were harvested from each age class after 6, 12, 18 and 24 months to evaluate needle litter decay over time. Percentage loss of initial mass was calculated after drying samples at 85 °C.
The needle litter decomposition rate (k) was calculated from the percentage of dry mass remaining using an exponential decay model ([38] - eqn. 1)
where Wt/Wo is the fraction of initial mass remaining at time t, t is the elapsed time (years) and k is the decomposition constant (y-1). As suggested by Olson ([38]), the times required for 50% and 95% mass loss were calculated as T50 = 1/k and T95 = 3/k, respectively.
For each sampling, the remaining litter mass, C, N, P, K, Ca, Mg, S, Mn and Fe concentrations were expressed as percentages of the initial values (Pij), according to the eqn. used by Baldantoni et al. ([5] - eqn. 2):
where C0j is the initial concentration of the j-th parameter analysed, W0 the initial litter weight, Cij is the concentration of the j-th parameter at the i-th sampling and Wi is the litter weight at the i-th sampling.
Statistical analysis
Means and standard deviations of litter quality parameters and litter decomposition rates were calculated for each stand age class (4 age classes and 3 replicates per age class). A two-way ANOVA (analysis of variance) was applied to analyse the effects of the stand age classes and time on the initial litter quality parameters using the software SPSS® ver. 17.0 for Windows (IBM, Armonk, NY, USA). Following the results of the ANOVA, Tukey’s HDS (Honestly Significant Difference) test (α = 0.05) was used to compare multiple litter quality parameters at each litter sampling time among age classes. Repeated measures ANOVA (RMANOVA) was adopted to test the effects of stand age class, time and their interactions on the differences in the litter decomposition rates.
Results
Fir stands, soil characteristics and litter chemistry
Stand characteristics and some mineral soil properties of the fir stand age classes are shown in Tab. 1. In general, the older stands (Fir90 and Fir100) had higher mean tree diameter, height, canopy closure and sand content and lower mean clay and silt contents than the younger stands (Fir38 and Fir60 - Tab. 1). The Fir90 stand showed the highest soil pH (5.99 ± 0.37) compared to the F38, Fir60 and Fir100 stands, which had similar soil pH (5.00 ± 0.23, 5.15 ± 0.30 and 5.16 ± 0.14, respectively). Soil bulk density, however, was similar in all fir stand age classes.
Initial litter parameters and the ratios of C/N and lignin/N in the needle litters are given in Tab. 2. The results showed that there were significant intraspecific variations (p<0.001) in the chemical compositions of Kazdagi fir litters among the different stand age classes, which in turn affected their decomposition rates. Initial litter carbon concentrations in Fir60 and Fir100 stands were higher (53.5% ± 1.9% and 53.2% ± 1.0%, respectively) than in Fir38 and Fir90 stands (50.4% ± 1.7% and 49.7% ± 1.6%, respectively). However, initial litter N and lignin concentrations in Fir60 and Fir90 stands (the middle-aged fir stands) were lower (1.11% ± 0.11% and 1.00% ± 0.1% for N, 14% ± 1.0% and 18% ± 4.0% for lignin, respectively) than in Fir38 and Fir100 stands (1.49% ± 0.1% and 1.39% ± 0.1% for N, 24% ± 3.0% and 21% ± 2.0% for lignin, respectively).
Tab. 2 - Initial litter quality parameters from the 4 different fir stand age classes. Means with different alphabets in rows indicate significant (p < 0.001) difference between the stand age classes.
Parameters | Units | Stand age classes (years) | |||
---|---|---|---|---|---|
Fir38 | Fir60 | Fir90 | Fir100 | ||
C | (%) | 50.4 ± 1.7 a | 53.5 ± 1.9 b | 49.7 ± 1.6 a | 53.2 ± 1.0 b |
N | (%) | 1.49 ± 0.1 b | 1.11 ± 0.11 a | 1.00 ± 0.1 a | 1.39 ± 0.1 b |
Hemicellulose | mg g-1 | 497 ± 13.3 a | 465 ± 12.0 a | 619 ± 15.4 b | 464 ± 6.0 a |
Cellulose | mg g-1 | 644 ± 6.0 a | 729 ± 1.0 b | 788 ± 7.0 b | 670 ± 2.0 a |
Lignin | (%) | 24 ± 3.0 b | 14 ± 1.0 a | 18 ± 4.0 b | 21 ± 2.0 b |
C:N | ratio | 34:01:00 | 48:01:00 | 50:01:00 | 38:01:00 |
L:N | ratio | 16:01:00 | 13:01:00 | 18:01:00 | 15:01:00 |
Ca | mg kg-1 | 23.630 ± 30 a | 20.500 ± 20 a | 33.080 ± 30 b | 32.470 ± 30 b |
Mg | mg kg-1 | 2185 ± 21 b | 1235 ± 15 a | 3034 ± 25 c | 2113 ± 21 b |
K | mg kg-1 | 6065 ± 15 a | 6221 ± 15 a | 7184 ± 16 c | 7838 ± 17 c |
P | mg kg-1 | 1871 ± 3 c | 1822 ± 3 bc | 1780 ± 3 b | 1570 ± 3 a |
S | mg kg-1 | 2073 ± 3 b | 2023 ± 3 b | 2120 ± 3 b | 1998 ± 3 b |
Mn | mg kg-1 | 2339 ± 3 c | 3044 ± 3 d | 458 ± 1.3 a | 2024 ± 3 b |
Fe | mg kg-1 | 1068 ± 3 c | 421 ± 2.5 a | 489 ± 2.1 b | 494 ± 2.4 b |
The Fir90 stand had the highest litter cellulose and hemicellulose concentrations (788 ± 7.0 and 619 ± 15.4 mg g-1, respectively) compared to the other 3 age classes, which showed relatively similar hemicellulose concentrations (~465 mg g-1); however, for cellulose concentration, age class order was Fir60 (729 ± 1.0 mg g-1), Fir100 (670 ± 2.0 mg g-1) and Fir38 (644 ± 6.0 mg g-1 - Tab. 2). Initial litter C/N ratios were higher in Fir60 and Fir90 (48:1 and 50:1, respectively) than in Fir38 and Fir100 (34:1 and 38:1, respectively). The Fir90 stands also had a higher initial litter lignin/N ratio (18:1) than the Fir60 and Fir100 stands (16:1 and 15:1, respectively). However, the Fir60 stands showed the lowest initial lignin/N ratio (13:1). In general, the middle-age fir stands (Fir60 and Fir90) had lower N and lignin concentrations but higher cellulose concentrations and C/N ratios compared to the younger (Fir38) and older (Fir100) fir stands (Tab. 2).
Overall, initial litter Ca, Mg and K concentrations were higher in the older fir stands (Fir90 and Fir100) than in the younger fir stands (Fir38 and Fir60 - Tab. 2). Initial litter, P and Mn concentrations, however, were lower in the older fir stands than in the younger fir stands. Initial Fe concentration was highest in the youngest fir stand (F38) as compared to the other fir stands, which had similar Fe concentrations (Tab. 2). Initial litter S concentration did not vary among the fir stand age classes.
Needle litter mass losses
Fig. 2shows the remaining mass of the fir needle litter in the four different stand age classes over 24 months. According to the ANOVA results, the main effects of time and the interaction of time and stand age on mass loss were all significant (p<0.001). These results demonstrate that litter mass losses of fir varied significantly between the sampling times and between the fir stand age classes.
After 6 months, the needle litters in Fir100 and Fir60 stands showed higher mean mass losses (45% and 44%, respectively) than in Fir90 and Fir38 (41% and 38%, respectively). There was a 7% difference in needle-mass losses between the oldest and youngest stands. At 12 months, the needle litter under Fir100 still had the highest mass losses (63%), while Fir38 had the lowest (54%). The needle litters had similar mass loss under Fir60 (57%) and Fir90 (57%). The differences in needle mass losses between the oldest and the youngest stands were higher at 12 months (by 9%) than at 6 months. At 18 months, the needle litters under Fir60 decomposed much faster and had the highest values (76%), followed by Fir100 (71%), Fir90 and Fir38, which had the same mass losses (62%). The differences in the needle mass losses between the oldest and the youngest stands at 18 months were much higher (14%). At 24 months, the needle litters under Fir60 had the highest mass loss (80%), followed by Fir100 (76%), Fir90 (69%) and Fir38 (68%). The differences in the needle mass losses between the oldest and the youngest stands at 24 months were still higher (12%).
Decay rates (k) and the time required for 50% [T50 (y)] and 95% mass loss [T95 (y)] for the needle litters from the different stand age classes are given in Tab. 3. The decay rates of the needle litters were higher in Fir60 and Fir100 stands (-0.948 and -0.944, respectively) than in Fir38 and Fir90 stands (-0.743 and -0.788, respectively). The calculated times required for 50% mass loss were higher in Fir38 (1.35 y) and Fir90 (1.27 y) than in Fir100 (1.06 y) and Fir60 (1.05 y). A similar order was seen for the time required for 95% mass loss, indicating that the needle litters under Fir38 and Fir90 need approximately 4 years to lose 95% of mass compared to the needle litters under Fir60 and Fir100, which need less time (~3 y).
Tab. 3 - Annual decomposition constant (k) and the time (year) required for 50% [T50 (y)] and 95% mass loss [T95 (y)] under 4 different fir stand age classes.
Parameters | Stand age classes (years) | |||
---|---|---|---|---|
Fir38 | Fir60 | Fir90 | Fir100 | |
k (yr-1) | -0.743 | -0.948 | -0.788 | -0.944 |
r2 | 0.975 | 0.519 | 0.946 | 0.979 |
F | p<0.001 | p<0.001 | p<0.001 | p<0.001 |
T50 (y) | 1.35 | 1.05 | 1.27 | 1.06 |
T95 (y) | 4.04 | 3.16 | 3.81 | 3.18 |
Initial litter C, P, K, Mg, Ca, S, Mn and Fe concentrations all decreased over time (Fig. 3). Initial N concentration also decreased over time but had two peaks at the 6-month and 18-month intervals (Fig. 3b). The needle litters in the middle age stands (Fir60 and Fir90) had higher peaks than Fir38 and Fir100. At the 12-month and 24-month intervals, N concentration in the needle litters decreased. Initial C, P and S concentrations decreased linearly and did not show any significant differences among the fir stand age classes (Fig. 3a, Fig. 3f and Fig. 3g, respectively). Initial K concentration showed a sharp decrease at the 6-month interval but remained relatively stable after that (Fig. 3e). Initial Ca, Mn and Fe concentrations also decreased over time but varied among the fir stand age classes (Fig. 3c, Fig. 3h and Fig. 3i, respectively).
Fig. 3 - Variation in total C (a), N (b), Ca (c), Mg (d), K (e), P (f), S (g), Mn (h) and Fe (i) concentrations in fir litters during decomposition period (24 months) from the 4 different stand age classes.
Discussion
Variation in fir litter quality with stand age classes
In temperate ecosystems, initial litter quality parameters (mainly lignin and nitrogen concentration) largely influence litter decomposition rates, which in turn influences the turnover of associated carbon and nutrients as a dominant source of organic matter input to the soil ([37], [2]). Initial litter quality also affects nitrogen availability for plants and soil N dynamics ([24]). Many studies have shown that higher litter C/N and lignin/N ratios are associated with slow rates of net soil N mineralization ([52], [51]). The specific quality of the litter that plants produce is, therefore, a key trait for the functioning of ecosystems ([60]).
To our knowledge, no study has directly investigated the forest stand age-related variability in litter quality of Kazdagi fir (Abies nordmanniana subsp. equi-trojani [Steven] Spach) and its consequences for litter decomposition processes. Our results indicated that initial N and lignin concentration in fir needle litter was lower in the middle-age fir stands compared to the younger- and older-age fir stands. Similar findings were also reported by Inagaki et al. ([26]) who found that N and lignin concentrations in leaf litter were lower in middle-aged forests than in young forests. The authors state that in the middle-aged forests, tree density decreases due to thinning practices or competition among individuals; and the N capital in the biomass increases, especially for evergreen species, indicating that N becomes a less limiting resource. They concluded that changes in N demand for plants are likely a major factor affecting lignin production in leaf litter. In contrast to those findings, Trap et al. ([60]) who studied pure beech (Fagus sylvatica) forest stands differing in age (15, 65, 95 and 130 years) found that lignin and lignin/N ratios were highest in stands of intermediate age.
In the present study, significant differences were evident in nutrient concentrations among the age classes of the fir stands; however, the differences did not clearly show a linear increase or decrease pattern with the stand age classes. Other researchers have also stated that the effect of the nutrient concentrations on litter due to stand age was not continuous ([64]). Trap et al. ([60]) found significantly high Mg, N and K litter concentrations in 15-year-old stands of pure beech that subsequently decreased with increasing stand age; Mn, on the other hand, was the only nutrient analysed that was highest in the oldest stands. They attributed the observed differences among stands of different ages to (i) the relative abundance of sun and shade leaves and (ii) increasing intraspecific competition for soil nutrients with increasing tree age ([42]).
Variation in initial litter quality parameters among fir subspecies
The initial litter C and N concentrations of Abies nordmanniana subsp. equi-trojani were partly comparable with the findings of other studies and occasionally lower or higher than their results depending on the fir subspecies used in their studies. Wei et al. ([63]) found that initial C concentration for Abies fabri was 51.3%, which was similar to the initial litter C concentration (51.7%) for Abies nordmanniana subsp. equi-trojani. Jagodzinski et al. ([28]) studied Abies alba stands ranging from 8-115 years old (12 different aged tree stands) in southern Poland and found that C concentration ranged from 50.4% (8 years old) to 52.7% (93 years old). A study by Wu et al. ([66]) showed that initial carbon and N concentrations of Abies faxoniana were 52% and 1.12%, respectively. Similarly, in a study of Abies faxoniana tree stands in western Sichuan, China, Wu et al. ([65]) found that initial C and N values were 51.6% and 0.92%, respectively. Sariyildiz et al. ([49]) reported that for Abies nordmanniana subsp. bornmuelleriana Maltfelt, initial litter was 47% C and 1.10% N. Accordingly, Abies nordmanniana subsp. equi-trojani had lower initial litter lignin concentrations (from 14% to 24%) than the litters of Abies alba (mean 30.5%) and Abies nordmanniana subsp. bornmuelleriana (mean 36%) but similar values with the litter of Abies faxoniana (mean 25%).
Initial litter hemicellulose and cellulose concentrations of Abies nordmanniana subsp. equi-trojani were much higher than the values reported for Abies alba ([25]), which were 199.9 mg g-1 for cellulose and 123.4 mg g-1 for hemicellulose. The same was true for Abies nordmanniana subsp. bornmuelleriana Maltfelt, which had initial litter cellulose concentrations between 260 mg g-1 and 290 mg g-1 ([49]). Palosuo et al. ([39]) found 416 mg g-1 for initial cellulose and 303 mg g-1 for initial lignin in Douglas-fir (Pseudotsuga menziesii [Mirb.] Franco) stands. Wu et al. ([65]) noted that initial lignin and cellulose concentrations for Abies faxoniana trees were 418.3 mg g-1 and 294.5 mg g-1, respectively. Mean C/N ratios in the litters of Abies nordmanniana subsp. equi-trojani (from 34:1 to 50:1) were similar to the litter of Abies nordmanniana subsp. bornmuelleriana Maltfelt, ranging from 38:1 to 51:1. However, mean lignin/N ratios were lower in the litters of Abies nordmanniana subsp. equi-trojani (from 13:1 to 18:1) than in the litter of Abies nordmanniana subsp. bornmuelleriana Maltfelt, ranging from 25:1 to 45:1. Palosuo et al. ([39]) found that C/N ratios and lignin/N for Douglas-fir were 56:1 and 45:1, respectively.
When comparing the findings of other studies with the initial litter P and K concentrations found in the present study, our values are higher than the values found for Abies alba ([25]), which were 1180 mg g-1 for P and 2200 mg g-1 for K. Our initial Ca and Mg concentrations were also much higher than the values found for Abies alba ([25]), which were 12.400 mg g-1 for Ca and 800 mg g-1 for Mg.
Variation in mass losses with stand age classes
Our results indicate that decomposition rate did not vary monotonically with the increase in stand age of fir. Needle litters under Fir100 and Fir60 stands decomposed faster than the needle litters under Fir90 and Fir38 stands. The faster rate of litter decomposition in Fir60 and Fir100 stands noted in the present study was associated with a lower value of lignin/N ratio (13:1 and 15:1, respectively) in the litter samples compared to Fir38 and Fir90 (16:1 and 18:1, respectively). It is generally argued that decomposition is relatively faster with litter of lower lignin content and in sites with a warm and humid climate; dry, wet and cold climates and poor litter quality would inhibit litter decomposition processes ([32], [7], [18]). Several studies have also reported significant correlations between lignin content or the lignin/N ratio and decay rates for a variety of litter types ([20], [6], [45]). For example, Melillo et al. ([37]) found strong, negative, linear relationships between initial lignin/N ratios and mass-loss rates of leaf litter from northern hardwoods and other areas. The conditions for microorganisms were possibly more favourable in Fir100 and Fir60 stands than Fir90 and Fir38 stands, resulting in the higher litter decomposition rates. Because lignin is a resistant substrate, more time is required for microbial decomposition; therefore, organic substances with large amounts of lignin decompose over longer periods ([44]). The lignin content was highest in Fir38 and lowest in Fir60, which may have affected litter decomposition. Long-term climate changes have the greatest effect on litter decomposition and carbon sinks. Such changes are not only related to temperature changes, but to seasonal precipitation changes as well, which can be more influential ([31]). Similarly, Fogel & Cromack ([20]) stated that the rapid decomposition of litter was due to excessive temperature differences (especially since it affects the water-soil regime), and that the mass-loss rate of coniferous species significantly affected the mass.
On the other hand, age-related forest stand changes in forest structure influence the quality and quantity of organic matter input and thus nutrient turnover ([26], [59]). Additionally, structural changes of the canopy layer during succession can also affect the microclimate on the forest floor due to alterations in solar radiation, wind speed or rainfall interception ([16], [55]). As shown by other studies, forest development over time can result in substantial changes in fir stand conditions, especially microclimate factors ([2]), soil fauna ([15]), soil microbial community composition ([58]) and nutrient cycling efficiency ([59]), which are seen as the most influential factors on litter mass loss.
Other studies using fir or different tree species have also found contrasting results with litter mass losses related to stand age. Klaus ([30]) noted that the litter decomposition rates for balsam fir (Abies balsamea) and white spruce (Picea glauca) needles increased slightly with increasing stand age. Welke & Hope ([64]) studied Douglas-fir (Pseudotsuga menziesii) and paper birch (Betula papyrifera) aged 10-25, 50-65 and >85 years old and found that Douglas fir litter decayed more in old stands than in younger stands, while the opposite trend was seen for birch litter. Yu et al. ([67]) assessed four Chinese fir plantations (7, 16, 23 and 29 years old) and found that litter decomposition rates decreased between the 7- and 16-year-old plantations, then significantly increased from the 16- to 23-year-old plantations and reached a peak in the 29-year-old plantations. Trogisch et al. ([61]) found that litter decomposition declined with forest stand age. Their results clearly showed that microclimatic site conditions were more favourable for litter decomposition in young forest stands. A study by Brun et al. ([12]) also showed that Scots pine needles in the 118-year-old stands decayed less than in the 70- and 53-year-old stands. Sharma & Ambasht ([53]) found that T1/2 value for Alnus nepalensis mass was lowest in the 30-year stand and highest in the 56-year stand, indicating that mass loss was much faster in the 30-year stand.
Variation in mass losses and nutrients during litter decomposition
As demonstrated by several authors ([46], [40]), the general trend in litter decomposition processes is initially rapid and then slows down. A similar trend was also seen in our study for fir litter decomposition (Fig. 2). The litters of Fir38, Fir60, Fir90 and Fir100 respectively lost 54%, 57%, 57% and 63% of their initial weight in the first year (0-12 months) compared with 14%, 23%, 12% and 13% losses in the second year (12-24 months). Initially, accelerated litter decomposition rates are mainly attributed to easier degradation of water-soluble organic compounds, while the later, slower litter decomposition rates are mostly due to difficulties in microbial degradation and mineralization of chemically recalcitrant complex compounds, such as polyphenols and lignin. Researchers have shown that litter N concentration has a strong, positive influence on initial litter decay (<3 months), as higher concentration of this element is associated with faster decomposition rates, while lignin and C/N ratios determine later rates of decomposition ([1]).
Mean mass loss in the fir stands was 58% after 12 months and 74% after 24 months. These values were much higher than the values shown by Sariyildiz & Küçük ([50]) for fir (31% and 52%, respectively). Wei et al. ([63]) noted that throughout the experiment, the dry weight loss rate of fir litter was 32%. The decay-rate coefficients (k) for fir litters (ranging from -0.743 to -0.948 in this study) were comparatively higher than the -0.370 k value reported by Sariyildiz & Küçük ([50]). Klaus ([30]) noted that the decay-rate coefficients (k) for balsam fir aged 1, 13, 16, 28 and 29 years were -0.26, -0.34, -0.31, -0.35 and -0.36, respectively, after 12 months.
Research suggests that nutrient concentrations vary to some extent during the decomposition period. An increase in litter N concentration (Fig. 3b) followed by a decline over time, as observed in this study, is similar to the patterns found in other studies ([13], [56]). Increases in N concentration could be attributed to the addition of N from exogenous sources to microbial biomass ([37], [57]). Among the nutrients, initial litter K and Mn (Fig. 3e and Fig. 3h, respectively) were lost from the decomposing litter mostly during the first 6 months. This indicates an initial leaching loss of K because of its solubility. The relative elemental transfer from the decomposing litter generally demonstrates that potassium is highly mobile and nitrogen is the least mobile (K > Ca > P > N). The results of Laskowski et al. ([34], [33]) revealed an initial rapid decrease in K concentration followed by stabilisation or a slow increase. Similarly, Brais et al. ([11]) demonstrated for Abies balsamea that K, Ca and Mg concentrations decreased with time. Gosz et al. ([21]) reported an increase in absolute phosphorus content in deciduous litter during the first year of decomposition. However, Edmonds ([19]) found that phosphorus was rapidly lost in the first three months, and absolute weights never exceeded initial weights in A. rubra and A. nepalensis litter. Sharma & Ambasht ([53]) demonstrated that the 30-year stand had a higher rate of phosphorus loss than the other stands, while the 56-year stand had the lowest rate. We did not study the differences in soil nutrient status and microclimate conditions of the different stand age classes of the fir species; however, the results indicate that those factors should be taken into account to fully understand the differences in the decomposition process among the different stand age classes.
Conclusion
Our results indicate that forest stand age is an important driver of intraspecific variability in fir litter quality parameters and decomposition rates with associated consequences for ecosystem functioning. The decomposition rate did not vary monotonically with increases in fir stand age. However, the results have clearly shown that the faster decomposition rates in Fir60 and Fir100 stands are associated with a lower value of lignin/N ratio in the litter samples compared to Fir38 and Fir90 stands. Unfortunately, these forest age-related intraspecific variations in litter quality and mass losses are unprecedented and largely unexplained. There is clearly a need for further studies focusing on those topics, their variation and their potential driving forces on soil processes.
Acknowledgements
This research has been supported by the Scientific Research Projects Coordination Department of the Kastamonu University (KUBAP), Turkey (Project no. KUBAP-03-2015-01).
References
Gscholar
CrossRef | Gscholar
Gscholar
Gscholar
CrossRef | Gscholar
Gscholar
CrossRef | Gscholar
Gscholar
Authors’ Info
Authors’ Affiliation
Kastamonu University, Faculty of Forestry, Department of Forest Engineering, 37150, Kastamonu (Turkey)
Bursa Technical University, Faculty of Forestry, Department of Forest Engineering, 16310, Bursa (Turkey)
Corresponding author
Paper Info
Citation
Savaci G, Sariyildiz T (2020). Effects of stand age on litter quality, decomposition rate and nutrient release of Kazdagi fir (Abies nordmanniana subsp. equi-trojani). iForest 13: 396-403. - doi: 10.3832/ifor3306-013
Academic Editor
Daniela Baldantoni
Paper history
Received: Dec 02, 2019
Accepted: Jun 22, 2020
First online: Sep 03, 2020
Publication Date: Oct 31, 2020
Publication Time: 2.43 months
Copyright Information
© SISEF - The Italian Society of Silviculture and Forest Ecology 2020
Open Access
This article is distributed under the terms of the Creative Commons Attribution-Non Commercial 4.0 International (https://creativecommons.org/licenses/by-nc/4.0/), which permits unrestricted use, distribution, and reproduction in any medium, provided you give appropriate credit to the original author(s) and the source, provide a link to the Creative Commons license, and indicate if changes were made.
Web Metrics
Breakdown by View Type
Article Usage
Total Article Views: 33788
(from publication date up to now)
Breakdown by View Type
HTML Page Views: 29723
Abstract Page Views: 1721
PDF Downloads: 1845
Citation/Reference Downloads: 1
XML Downloads: 498
Web Metrics
Days since publication: 1540
Overall contacts: 33788
Avg. contacts per week: 153.58
Article Citations
Article citations are based on data periodically collected from the Clarivate Web of Science web site
(last update: Feb 2023)
Total number of cites (since 2020): 1
Average cites per year: 0.25
Publication Metrics
by Dimensions ©
Articles citing this article
List of the papers citing this article based on CrossRef Cited-by.
Related Contents
iForest Similar Articles
Research Articles
Effects of arbuscular mycorrhizal fungi on microbial activity and nutrient release are sensitive to acid deposition during litter decomposition in a subtropical Cinnamomum camphora forest
vol. 16, pp. 314-324 (online: 13 November 2023)
Research Articles
Litter quality changes during decomposition investigated by thermal analysis
vol. 8, pp. 827-837 (online: 19 March 2015)
Research Articles
Effect of plant species on P cycle-related microorganisms associated with litter decomposition and P soil availability: implications for agroforestry management
vol. 9, pp. 294-302 (online: 05 October 2015)
Research Articles
No home-field advantage in upper Andean tropical forests despite strong differences in site environmental characteristics
vol. 17, pp. 286-294 (online: 27 September 2024)
Research Articles
Soil fauna communities and microbial activities response to litter and soil properties under degraded and restored forests of Hyrcania
vol. 14, pp. 490-498 (online: 11 November 2021)
Research Articles
Changes in organic compounds during leaf litter leaching: laboratory experiment on eight plant species of the Sudano-guinea Savannas of Ngaoundere, Cameroon
vol. 1, pp. 27-33 (online: 28 February 2008)
Research Articles
The manipulation of aboveground litter input affects soil CO2 efflux in a subtropical liquidambar forest in China
vol. 12, pp. 181-186 (online: 10 April 2019)
Research Articles
Mid-rotation fertilization and liming of Pinus taeda: growth, litter, fine root mass, and elemental composition
vol. 14, pp. 195-202 (online: 24 April 2021)
Research Articles
Modeling of early stage litter decomposition in Mediterranean mixed forests: functional aspects affected by local climate
vol. 8, pp. 517-525 (online: 18 November 2014)
Research Articles
Contribution of legume and non-legume trees to litter dynamics and C-N-P inputs in a secondary seasonally dry tropical forest
vol. 15, pp. 8-15 (online: 13 January 2022)
iForest Database Search
Search By Author
Search By Keyword
Google Scholar Search
Citing Articles
Search By Author
Search By Keywords
PubMed Search
Search By Author
Search By Keyword