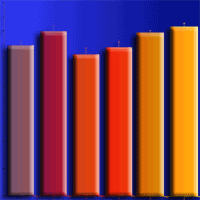
The responses of soil microbial community and enzyme activities of Phoebe zhennan cultivated under different soil moisture conditions to phosphorus addition
iForest - Biogeosciences and Forestry, Volume 11, Issue 6, Pages 751-756 (2018)
doi: https://doi.org/10.3832/ifor2725-011
Published: Nov 15, 2018 - Copyright © 2018 SISEF
Research Articles
Abstract
The importance of conservation and ecological restoration of the rare and economically important tree Phoebe zhennan is increasingly recognized. To this purpose, phosphorus (P) addition has been proposed to improve soil biological attributes and face the anticipated drought under climate change, though few studies have investigated its effect on the interaction between the soil microorganisms and plant host, as well as on ecosystem productivity. We investigated the effect of P addition on soil chemical properties, microbial communities, and enzyme activities in a soil planted with P. zhennan under two levels of water treatments (optimum water and drought treatments). P additions had no significant effect on microbial communities, dissolved organic nitrogen (DON), pH and soil moisture (SM), though the available P (aP) increased. Compared with no P treatment, alkaline phosphate and β-fructofuranosidase activities increased with P additions in the drought treatment. Drought decreased the total phospholipid-derived fatty acids (PLFAs), arbuscular mycorrhiza fungi (AMF), and fungi PLFAs compared to the well-watered. These findings indicated that P additions does not ameliorate the impact of drought on soil microbial communities and enzyme activities, except alkaline phosphate and β-fructofuranosidase, and P may not be responsible for regulating biochemical processes essential for maintaining the fertility of soil planted with P. zhennan under drought conditions. It is hypothesized that the lack of effects of P addition on the majority of the microbial properties could be due to the soil mechanism employed by P. zhennan to tolerate harsh conditions.
Keywords
Alkaline Phosphatase, Biomass, Drought, Enzymes, Microbial, Phoebe zhennan
Introduction
Phoebe zhennan S. Lee (Family: Lauraceae; common name: Golden Phoebe), also known as Nanmu, is a well-known and rare tree species valued for its high timber quality and medicinal values ([10]). P. zhennan is listed as a threatened species by the International Union for Conservation of Nature (IUCN), and it is protected nationwide in China ([7]). In the past few years, there has been a decline in the acreage of P. zhennan ([2]). At the moment, cultivation of P. zhennan has gained attention for use in tree resource conservation and ecological restoration projects. A program focusing on the protection and development of rare and precious tree species resources is currently being undertaken in Sichuan province ([10]). However, the increased aridity due to climate change may have implications for P. zhennan, either direct or indirect. To face this problem, it is therefore necessary to develop improved soil management practices such as phosphorus (P) application. Indeed, our previous study suggested that P addition could improve drought tolerance of P. zhennan seedlings through physio-biochemical adjustments ([27]), though the impact of P on soil chemical properties, microbial communities, and enzyme activities has not been investigated yet. Moreover, P in soil is subject to extensive physicochemical and biological reactions, with only a small component of total soil P being in a form directly available for plant or microbial uptake ([8]). The seedling stage in forest restoration is critical for successful establishment. The application of P might increase the tolerance of soil microbes to soil water deficit ([17], [11]) and subsequently improve seedling performance after transplantation in the field.
Soil microorganisms are the critical link between the composition of above ground plant species and ecosystem functioning ([33]). Recent studies reported a close two-way interaction between microbial communities and plant host as a vital determinant of plant health and productivity ([21], [20], [9]). While plants can affect soil microbial communities via their biomass, nutrient demand and water use efficiency, plant development can be strongly influenced by soil microbial communities ([23]). This reciprocal exchange and interactions between plants and soil microbial communities underpins forest soil health, ecosystem function, and the ecosystem resilience after disturbance, which is fundamental in response to global change ([23]). Although several studies have reported that the coupling between plant and soil microorganisms is vital for plant performance and maintenance of soil health ([30]), both plants and soil microorganisms can be affected by change in soil water content and nutrient availability, such as P ([22], [3], [24]). Moreover, the shifts in soil chemical properties and biological parameters can provide an initial indication of soil quality because of their sensitivity, rapid response to short-term disturbances, and capacity to integrate many environmental factors ([19], [28]).
Changes in soil water content and nutrient availability can affect soil microbial communities directly by altering their activity, but also indirectly through their influence on aboveground tree species ([11], [23], [15]). Several studies have reported that drought can reduce the water potential of the physical soil matrix, and worsen nutrient deficiency by reducing its acquisition, mobility, and accessibility in the soil for plants and soil biota ([3], [24]). Therefore, it is anticipated that improved practices such as P application could buffer soil conditions, halting and even reversing the adverse effects of P deficiency in soils and increasing the stress-tolerating ability of soil microbes ([17], [11], [15]). The influence of P on aboveground plant communities is well understood; however, much less is known about the impact of P addition on belowground microbial communities. For instance, a limited response of soil microbial biomass and some enzyme activities to P application have been reported ([32], [7]). Such feedback may be context-dependent and could vary depending on the influence of host plant species, soil water content, and other variables ([30], [24]). To our knowledge, even though the necessity for conservation and ecological restorations of P. zhennan is well recognized, there is limited information about the effect of P addition as an improved management practice and its interaction with soil water content on P. zhennan chemical properties, microbial communities, and enzyme activities. Hence, understanding the effects of P addition on belowground microbial communities associated with P. zhennan seedlings under various levels of water treatment may be beneficial to improve cultivation strategies and may also provide better insights into effective management practices to maintain soil health under varying climate conditions.
In this study, we conducted a P addition experiment with two levels of water treatment (optimum water addition and drought) in a soil planted with P. zhennan, and measured the response of soil chemical properties, microbial communities, and enzyme activities. Our aim was to determine if P addition affects the soil chemical parameters, microbial community, and enzyme activities of soil planted with P. zhennan under changes in soil water content, as well as to ascertain if improved practices such as P application could buffer the soil environment against drought conditions.
Materials and methods
Experimental design
This study was conducted at the Center for Ecological Studies, Chinese Academy of Science, Sichuan Province, China (104° 04′ E, 30° 37′ N). The study area has a mild subtropical monsoon climate with a mean annual temperature of 16.7 °C and precipitation of 945.6 mm. Soils used in this study were collected at 15 cm depth and thoroughly mixed. The chemical properties of the soil samples were 0.89 g kg-1 total phosphorus (TP), 27.6 mg kg-1 available phosphorus (aP), 1.9 g kg-1 total nitrogen (TN), 26.7 g kg-1 total carbon (TC), and pH of 7.3.
A complete randomized experimental design was established consisting of two levels of water treatment (well-watered and drought) and two levels of P application (with and without P). The P. zhennan seedlings used in this study were grown for approximately two years, after which healthy and uniform seedlings were transplanted into 30 (10 L) pots filled with approximately 4000 g of a homogenized soil sample. Then, the plants were grown for another two months to allow adequate acclimation to the new environment before treatments were applied.
After 2 months growth, 20 healthy plants were selected and subjected to five replicates of four treatments: water with P-addition (WP), water only (W), drought with P-addition (DP), and drought only (D), for 3 months. Phosphorus fertilizer was sup-plied as sodium dihydrogen phosphate (NaH2PO4, 25.5% P) at the rate of 129.3 mg P per pot. The fertilizer was mixed with 200 ml of water per pot and supplied every 30 days for three months.
The soil-relative water content (SRWC) was determined according to Xu et al. ([31]) and categorized into two levels: optimum water addition (80%-85%) and severe drought (30%-35%). The SRWC of each pot was maintained by replacing the amount of water transpired and evaporated. A distance of 40 cm was maintained between pots to prevent shading by neighboring plants.
Determination of soil parameters
The soil obtained after removing plants from the pots was thoroughly homogenized and transferred to the laboratory in a sealed plastic bag. Soil moisture content was determined gravimetrically from mass loss after oven drying 10 g of moist soil to a constant weight at 105 °C for 24 h. The pH was measured using a pH meter (PHS-25®, INESA Instruments, China) at a ratio of 25 ml deionized water to 10 g soil. Ammonia (NH4+-N) and nitrate nitrogen (NO3--N) were extracted with 50 ml of 2.0 M KCl and determined on a flow injection auto-analyzer (AutoAnalyzer3®, Bran-Luebbe, Germany). The aP was extracted with 0.5 M NaHCO3 (pH 8.2) according to Olsen & Sommers ([16]) and measured colorimetrically by the molybdate-ascorbic acid method ([14]). Dissolved organic carbon (DOC) and nitrogen (DON) were extracted from fresh moist soils with an addition of 2 M KCl at 20 °C and measured using a TOC/ TN analyzer (Multi N/C 2100(S)®, Analytik Jena AG, Germany).
Estimation of microbial communities
Using phospholipid-derived fatty acids (PLFAs - [1]), soil microbial communities were assessed. Microbial lipids were extracted from frozen soil samples corresponding to 8 g dry mass of soil, placed in 50 ml centrifuge tubes, and then extracted with a mixture of chloroform (CHCl3), methanol, and phosphate buffer (1:2:0.8). The fatty acids in the lower chloroform phase were saponified and transformed into fatty acid methyl esters using a strong acid methylation. PLFAs methyl esters were separated and identified using gas chromatography (model 6890N®, Agilent, USA) coupled with a flame ionization detector (model 19091B-102®, Agilent, USA) and an HP-5 capillary column. The concentration of each PLFAs was quantified according to the 19:0 internal standards (nonadecanoic acid methyl ester). The abundance of individual fatty acids in each sample was expressed as nmol per g dry soil. Individual fatty acids were used to indicate broad functional groups of microorganisms according to existing PLFAs biomarker data ([6], [12]). Actinomycetes and fungi were expressed by the PLFAs 10me 18:0, 10me 17:0, 10me 16:0, 10me 19:0 and 18:1ω9c, 18:2ω6.9c, 16:1ω5c, respectively. PLFAs 16:1ω5c was used as the maker for arbuscular mycorrhiza fungi (AMF). The sum PLFAs i15:0, i16:0, a17:0, i14:0, a15:0, i17:0, i13:0, a16:0, i18:0 were chosen to represent the Gram-positive (G+) bacteria, while Gram-negative (G-) bacteria were express by the sum of 16:1ω7c, 15:1ω6c, 16:1ω11c, 18:1ω7c, cy17:0, 17:1ω8c, 16:1 2OH, 11Me18:1ω7c, 18:1ω5c, cy19:0ω8c. The sum of all extracted PLFAs was used as the estimate of total microbial biomass (totPLFAs).
Enzyme activities assay
Activities of soil enzymes, including, β-glucosidase, alkaline phosphatase (AP), amidohydrolase (urease), and β-fructofuranosidase (invertase) were measured colorimetrically using the procedure of Tabatabai & Bremner ([25]), Tabatabai ([26]), and Torres et al. ([29]). Alkaline phosphatase activities were determined by the release of p-nitrophenol (PNP) after cleavage of the enzyme-specific synthetic substrate. One gram of soil was incubated for 1 h at 37 °C with a mixture of 4 ml modified universal buffer (pH 6.5), 0.2 ml toluene, and 1 ml appropriate substrate solution. One ml 0.5 M NaOH and 4 ml 0.5 M CaCl2 were used to stop the reaction and allow for color development. The soil solution was then filtered and analyzed colorimetrically at 660 nm. β-glucosidase activity was measured by adding 2 ml of MUB pH 6.0 and 0.5 ml of 0.025 mol l-1 p-nitrophenyl β- D-glucopyranoside to 1 g soil sample and determined colorimetrically at 400 nm after incubating the mixtures at 37 °C. A control test was performed for each sample and the control (without soil), with the substrate being added after the reactions were stopped. For the determination of β-fructofuranosidase activities, 2 g air-dried soil sample was extracted with 0.2 ml toluene, 5 ml phosphate buffer solution, and 5 ml 10% sucrose solution. The mixture was incubated at 37 °C for 2 h. The resultant mixture was treated with a 3 ml of 3.5-dinitrosalicylic acid solution and determined colorimetrically at 508 nm. The urease activities were determined by incubating 2 g soil samples with an aqueous urea solution at 37 °C for 2 h. NH4+ released from the soil was extracted with 1 M KCl solution and measured by a modified indophenol-blue colorimetric reaction.
Data analysis
For all the data obtained, homogeneity of variance was tested and log-transformation was applied when necessary (applicable to soil enzyme activities). Data obtained for chemical properties, enzyme activities, and microbial communities were analyzed by analysis of variance (two way-ANOVA) using SPSS® ver. 18.0 (SPSS Inc., Chicago, IL, USA). The treatment means were compared using Duncan’s multiple range tests at the 0.05 probability level. Figures were prepared using Origin pro ver. 8.5 (OriginLab Corp., Northampton, MS, USA).
Results
Effects of P addition and water deficit on soil chemical properties
P addition had no significant effects on soil NH4+-N, DON, pH, and soil moisture contents (Tab. S1 in Supplementary material). Soil aP significantly increased (p < 0.05) in soils receiving P addition irrespective of the level of water treatments compared to those without P addition (Tab. 1). The aP of the drought treatments was higher (+P and -P: 105.33 and 48.66 mg kg-1 dry soil, respectively) than that of the well-watered (+P and -P: 71.33 and 44.66 mg kg-1, respectively) treatments. P addition significantly increased (p < 0.05) NO3--N in well-watered treatments (8.23 mg kg-1) compared to no P (5.52 mg kg-1), but no significant differences were observed in NO3-- N between the P and no P additions in drought treatments. The DOC contents significantly decreased (p < 0.05) in soil receiving P addition irrespective of the level of water treatments (Tab. 1). Generally, NO3--N, DON and aP in drought treated soils was higher than those of the well-watered soil. The result of the ANOVA showed that the interactions of water and P treatments had no significant effects on the majority of the soil chemical properties, except NO3-- N and aP (Tab. S1 in Supplementary material).
Tab. 1 - Effects of P additions under the two level of water treatments on soil chemical properties. Values are means ± SE. Means with different letters represent significant differences (p<0.05) among the four treatments after Duncan’s test. (SM): soil moisture (%); (pH): soil pH; (DOC): dissolved organic carbon; (DON): dissolved organic nitrogen; (NH4+-N): soil ammonium nitrogen (mg kg-1); (NO3--N): soil nitrate nitrogen (mg kg-1); (aP): available phosphorus.
Parameters | Well-watered | Drought | ||
---|---|---|---|---|
-P | +P | -P | +P | |
NO3--N (mg kg-1) | 5.52 ± 0.05 c | 8.23 ± 0.36 b | 9.73 ± 0.48 a | 9.19 ± 0.57 a |
NH4+N (mg kg-1) | 0.16 ± 0.04 b | 0.17 ± 0.01 ab | 0.14 ± 0.01 b | 0.24 ± 0.02 a |
DOC (mg kg-1) | 351.54 ± 32 a | 259.73 ± 5.95 b | 305.45 ± 34 a | 255.18 ± 11.32 b |
DON (mg kg-1) | 103.29 ± 1.38 a | 117.83 ± 6.84 a | 114.27 ± 7.73 a | 128.85 ± 13.18 a |
aP (mg kg-1) | 44.66 ± 3.30 c | 71.33 ± 0.66 b | 48.66 ± 3.52 c | 105.33 ± 3.33 a |
pH | 7.52 ± 0.09 a | 7.71 ± 0.11 a | 7.50 ± 0.08 a | 7.58 ± 0.03 a |
SM | 15.51 ± 0.86 a | 16.28 ± 0.70 a | 9.21 ± 0.41 b | 9.05 ± 0.56 b |
Phosphorus addition and water deficit impacts on soil microbial community and enzyme activities
Soil microbial communities, including total PLFAs, AMF, fungal PLFAs, G- bacteria, total bacteria, and actinomycete PLFAs in P addition treatments were not significantly different from the no P treatments in well-watered or drought treated soils (Fig. 1, Fig. 2b, Fig. 2c). Compared with the no P addition treatments, P addition significantly increased (p < 0.05) G+ bacteria in well-watered treatments (Fig. 2a).
Fig. 1 - Response of soil microbial community, (a) total PLFAs, (b) AMF, (c) fungi PLFAs, (d) G- bacterial PLFAs to phosphorus (P) application in well-watered and drought treatments. Different lowercase letters indicate significant differences (p<0.05) among the treatments. The error bar represents standard error (SE).
Fig. 2 - Response of soil microbial community, (a) G+ bacterial PLFAs, (b) total bacterial PLFAs, and (c) actinomycetes PLFAs to phosphorus (P) application in well-watered and drought treatments. Different lowercase letters indicate significant differences (p<0.05) among the treatments. The error bar represents standard error (SE).
A significant effect of water treatments was observed on total PLFAs, AMF, fungi, G+ bacteria, and total bacteria PLFAs (Tab. S2). Drought decreased the total PLFAs, AMF, fungi, and total bacteria compared to well-watered treatments (Fig. 1a, Fig. 1b, Fig. 1c, Fig. 2b). There were no significant differences in G- bacteria and actinomycete PLFAs in the well-watered and drought treatments (Fig. 1d, Fig. 2c). The results of the ANOVA indicated that there were no significant interactive effects of P addition and water treatments on all the microbial communities (Tab. S2 in Supplementary material).
The effects of P addition on soil enzyme activities varied depending on the level of water treatments. P addition significantly increased (p < 0.05) alkaline phosphate and β-fructofuranosidase activities in drought treatments but had no significant effects in well-watered treatments (Fig. 3a, Fig. 3b). β-glucosidase activity was lower with P addition, irrespective of the level of water treatments, although there were no significant differences compared to their no P counterparts (Fig. 3c).
Fig. 3 - Response of soil enzyme activities, (a) alkaline phosphate, (b) β-fructofuranosidase, (c) β-glucosidase and urease to phosphorus (P) application in well-watered and drought treatments. Different lowercase letters indicate significant differences (p<0.05) among the treatments. The error bar represents standard error (SE).
The result of the ANOVA showed that P addition and its interactions with water treatment had no significant effects on any enzyme activity (Tab. S3 in Supplementary material). However, independent factors of water treatments alone significantly affected alkaline phosphate, β-glucosidase, and urease activities (Tab. S3). Urease and β-glucosidase activities were higher in well-watered treatments than in the drought treatments (Fig. 3c, Fig. 3d).
Discussion
A comprehensive understanding of the interactive influence of climate and biotic factors on soil microbial communities and enzyme activities is vital for our understanding of changes in soil health ([33]), particularly as the aridity of the soil environment limits P availability for plant growth in many soils ([19]). In this study, we determined the effects of P addition on soil chemical properties, microbial communities, and enzyme activities in soil planted with P. zhennan at two levels of water treatments. Consistent with previous studies ([22], [23]), it was observed that the majority of the microbial communities and enzymes activities were partitioned along the water levels. The decrease in the total PLFAs, AMF, fungi, and total bacteria in drought treatments compared to well-watered treatments indicated that microbial communities were under physiological or nutritional stress, most probably because of competition between plant and soil microrganisms for limited accessible nutrients under drought conditions ([11], [3], [15]). Studies have shown that decreases in soil moisture also reduce N and/or P uptake by plants ([5], [21]). Likewise, the higher NO3--N and DON in drought treatments compared to the well-watered treatments suggested a reduction in nutrient uptake by P. zhennan during drought conditions. The negative feedback of less water and lower nutrient uptake could negatively affect the production capacity and fitness of plants when drought persists or becomes more severe ([22], [3], [23]). Nevertheless, the abundance of actinomycetes, a heterogeneous group of bacteria noted for their filamentous and branching growth pattern, which may confer resistance to desiccation, were similar for both well-watered and drought treatments. These results suggested that P. zhennan may adapt to drought conditions by enhanced hydraulic conductance of the root system ([27]) and increased production of biochemical compounds, which in turn increases actinomycete abundance ([13]).
Contrary to the hypothesis that improved management practices such as P application could buffer soil conditions and increase the drought-tolerating ability of soil microbes ([17], [11]), we found that P addition had no significant impact on the total PLFAs, AMF, fungi PLFAs, G- bacteria, total bacteria, and actinomycete PLFAs of P. zhennan. Although the root biomass of P. zhennan was enhanced with P addition, as reported by Tariq et al. ([27]), the DOC, which represents the labile soil carbon and acts as the energy source for soil microbes ([11]), decreased with P addition. This may be the reason for our results, which showed no significant effects of P addition on microbial communities. Moreover, the leaf dry matter, which can be used as a proxy for the proportion of vascular tissue, cellulose, insoluble sugar, and leaf lignin, and thus an additional carbon source for microbial communities, was lower with P addition ([18], [27]). Leaf nitrogen content is an indicator of plant growth and resource uptake, and it has been recognized that N-rich leaves could lead to bacterial dominated soil microbial communities ([18]). Corresponding to the lower leaf nitrogen content reported by Tariq et al. ([27]) in drought-treated soil regardless of P addition, we observed that bacterial communities were lower in drought-treated soil. This further suggests that the application of P as an improved management strategy could buffer the soil environment of P. zhennan against drought conditions. Studies have reported substantial increases in phosphatase activity in the rhizosphere of plants experiencing depletion of soil organic P ([19]). However, we observed that increased aP corresponded to an increase in alkaline phosphate in P treated dry soil. The mechanism underpinning this observation may be that even in the presence of high aP, P uptake by P. zhennan was constrained, hence hampering the effective utilization of P by P. zhennan under drought stress ([19], [21]). Taken together, the observed differences in the microbial communities appears to be related more to other abiotic variables or the growing nature of P. zhennan rather than to the availability of P due to P addition. Moreover, P. zhennan is a slow-growing forest tree species with a low mineral absorption rate and a slow growth strategy ([4]). Examining the impact of P addition on the drought tolerance of P. zhennan, Tariq et al. ([27]) demonstrated that, although drought stress severely affected the growth and metabolism, the species employs a range of strategies to tolerate harsh conditions. The strategies include limited stomatal conductance and transpiration rate, increased antioxidative activities, and accumulation of osmoprotectors. Although P. zhennan exhibits some inherent strategy which allowed it to lower the critical P requirement for growth and allowed its soil environment to operate at lower accessible levels of soil P ([19]), we suggest that the negative impact of less water and lower nutrient uptake could impact on the production capacity and fitness of P. zhennan when drought persists or becomes more severe. Our ongoing work is investigating other management strategies that could maintain soil health for the economically important but threatened P. zhennan under adverse environmental conditions.
Conclusions
Our results indicates that P addition does not have an impact on the composition of soil microbial communities or enzyme activities, except alkaline phosphate and β-fructofuranosidase, which are sensitive indicators of soil quality. In this study, the composition of microbial communities tended to be more sensitive to the level of water treatment than to P addition. Drought decreased the soil total PLFAs, AMF, fungal PLFAs, and G+ bacteria compared to well-watered treatments, irrespective of P addition. These results suggest that as P application may not be able to sustain the microbial properties of soil planted with P. zhennan, the negative impact of less water and lower nutrient uptake could impact the production capacity and fitness of P. zhennan when drought persists. The present findings highlight the need to further investigate a wide range of management strategies to maintain soil health for the economically important but threatened P. zhennan under changes in environmental conditions.
Acknowledgements
This study was supported by National Key R&D program of China (Grant no. 2016 YFC0502101 and 2017YFC0505000), the Ministry of Sciences and Technology of China (Grant no. 2015BAD07B050304) and the National Natural Science Foundation of China (Grant nos. 41301315, 31370632 and 31500517). OA Olatunji thankfully acknowledges Chinese Academic of Sciences and The Word Academic of Sciences (CAS-TWAS) for the Fellowship. The authors appreciated the assistance of X. Tan and D. Huang, during the collection and preparation of the materials for this study.
Conflict of Interest
The authors declare that they have no conflict of interest.
References
Gscholar
CrossRef | Gscholar
Gscholar
Authors’ Info
Authors’ Affiliation
Kaiwen Pan
Akash Tariq
Lin Zhang
Xiaogang Wu
Xiaoming Sun
Dagang Song
Key Laboratory of Mountain Ecological Restoration and Bioresource Utilization and Ecological Restoration Biodiversity Conservation Key Laboratory of Sichuan Province, Chengdu Institute of Biology, Chinese Academy of Sciences, Chengdu 610041 (People’s Republic of China)
Akash Tariq
Dagang Song
University of Chinese Academy of Sciences, Beijing 100039 (People’s Republic of China)
Ningning Li
College of Resources and Environment, Southwest University, Chongqing 400715 (People’s Republic of China)
Corresponding author
Paper Info
Citation
Olatunji OA, Pan K, Tariq A, Zhang L, Wu X, Sun X, Luo H, Song D, Li N (2018). The responses of soil microbial community and enzyme activities of Phoebe zhennan cultivated under different soil moisture conditions to phosphorus addition. iForest 11: 751-756. - doi: 10.3832/ifor2725-011
Academic Editor
Tamir Klein
Paper history
Received: Jan 09, 2018
Accepted: Aug 27, 2018
First online: Nov 15, 2018
Publication Date: Dec 31, 2018
Publication Time: 2.67 months
Copyright Information
© SISEF - The Italian Society of Silviculture and Forest Ecology 2018
Open Access
This article is distributed under the terms of the Creative Commons Attribution-Non Commercial 4.0 International (https://creativecommons.org/licenses/by-nc/4.0/), which permits unrestricted use, distribution, and reproduction in any medium, provided you give appropriate credit to the original author(s) and the source, provide a link to the Creative Commons license, and indicate if changes were made.
Web Metrics
Breakdown by View Type
Article Usage
Total Article Views: 40632
(from publication date up to now)
Breakdown by View Type
HTML Page Views: 35454
Abstract Page Views: 2180
PDF Downloads: 2365
Citation/Reference Downloads: 20
XML Downloads: 613
Web Metrics
Days since publication: 1990
Overall contacts: 40632
Avg. contacts per week: 142.93
Article Citations
Article citations are based on data periodically collected from the Clarivate Web of Science web site
(last update: Feb 2023)
Total number of cites (since 2018): 2
Average cites per year: 0.33
Publication Metrics
by Dimensions ©
Articles citing this article
List of the papers citing this article based on CrossRef Cited-by.
Related Contents
iForest Similar Articles
Research Articles
Responses of Taxus chinensis and Phoebe chekiangensis seedlings to controlled-release fertilizer in various formulations and application rates
vol. 12, pp. 254-261 (online: 03 May 2019)
Research Articles
Links between phenology and ecophysiology in a European beech forest
vol. 8, pp. 438-447 (online: 15 December 2014)
Short Communications
Is microbial biomass measurement by the chloroform fumigation extraction method biased by experimental addition of N and P?
vol. 14, pp. 408-412 (online: 04 September 2021)
Research Articles
Oak sprouts grow better than seedlings under drought stress
vol. 9, pp. 529-535 (online: 17 March 2016)
Research Articles
Nutrient uptake, allocation and biochemical changes in two Chinese fir cuttings under heterogeneous phosphorus supply
vol. 11, pp. 411-417 (online: 05 June 2018)
Research Articles
Effect of drought stress on some growth, morphological, physiological, and biochemical parameters of two different populations of Quercus brantii
vol. 11, pp. 212-220 (online: 01 March 2018)
Research Articles
Gas exchange, biomass allocation and water-use efficiency in response to elevated CO2 and drought in andiroba (Carapa surinamensis, Meliaceae)
vol. 12, pp. 61-68 (online: 24 January 2019)
Research Articles
Effects of altitudinal gradients on leaf area index, soil microbial biomass C and microbial activity in a temperate mixed forest ecosystem of Northwestern Turkey
vol. 10, pp. 334-340 (online: 15 December 2016)
Research Articles
Relationship between microbiological, physical, and chemical attributes of different soil types under Pinus taeda plantations in southern Brazil
vol. 17, pp. 29-35 (online: 28 February 2024)
Research Articles
Effect of salt and drought on growth, physiological and biochemical responses of two Tamarix species
vol. 8, pp. 772-779 (online: 25 March 2015)
iForest Database Search
Search By Author
Search By Keyword
Google Scholar Search
Citing Articles
Search By Author
Search By Keywords
PubMed Search
Search By Author
Search By Keyword