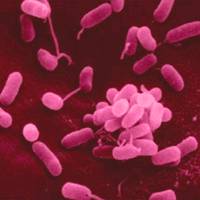
Controlled-release fertilizers combined with Pseudomonas fluorescens rhizobacteria inoculum improve growth in Pinus halepensis seedlings
iForest - Biogeosciences and Forestry, Volume 8, Issue 1, Pages 12-18 (2015)
doi: https://doi.org/10.3832/ifor1110-007
Published: May 12, 2014 - Copyright © 2015 SISEF
Research Articles
Abstract
Pinus halepensis seedlings are currently used to regenerate arid Mediterranean regions. Optimized methods for seedling fertilization in nurseries improve plant growth and are essential for successful reforestation. Previously, we showed that inoculation of P. halepensis seedlings with Pseudomonas fluorescens CECT 844 rhizobacteria improved plant growth and N uptake. The aim of this study was to determine the physiological and morphological response of P. halepensis seedlings to a combined treatment including controlled-release fertilization and inoculation with the rhizobacterium P. fluorescens. P. halepensis seedlings were grown in a nursery under well-watered conditions and were fertilized (F), inoculated with P. fluorescens (Ps) or fertilized and inoculated (F x Ps). Growth and water parameters (osmotic potential at both full and zero turgor and modulus of elasticity) were measured in seedlings under each treatment. The total N, P, K, Ca, Mg and Fe contents and concentrations in seedling roots and shoots were also measured. Finally, root growth potential was estimated. F x Ps increased both seedling growth and nutrient uptake compared with the independent treatments. Interestingly, amendment with rhizobacteria had a slight negative effect on osmotic potential and P uptake, which was lessened by combining Ps with F. The present work shows that F x Ps is highly efficient for improving the quality of forest seedlings in nurseries. As such, F x Ps represents a potential alternative treatment that could reduce contaminant emissions and increase microbiota soil regeneration in degraded soils.
Keywords
Controlled-release Fertilization, Rhizobacteria, Pinus halepensis, Water Parameters, Mineral Nutrition, Nursery, Root Growth Potential, Osmotic Potential
Introduction
Pinus halepensis is one of the most common tree species in the Mediterranean and is also frequently used for reforestation purposes ([16]). Several studies have been carried out with the aim of improving the quality of P. halepensis seedlings obtained from nurseries ([5]). The application of chemical fertilizer treatments to forest seedlings in nurseries is the most common cultivation practice. However, it has been established that soil amendments, including ectomycorrhizal fungi and plant growth-promoting rhizobacteria (PGPR), increase plant survival and seedling quality, especially in soils with low microbial activity ([7], [24]).
Pseudomonas fluorescens has several characteristics of an effective PGPR and may function as a plant growth stimulator that efficiently promotes seed germination, accelerates growth in the early stages, induces root initiation, enhances the formation of roots and root hairs, facilitates root regeneration and helps to control pathogens in certain forest species. P. fluorescens is easily cultivated in vitro and colonizes a wide range of ecological niches, including the rhizospheres of plants ([3]); additionally, P. fluorescens genomes are highly diverse ([30]), which likely increases the survival capacity of this microorganism. P. fluorescens improves plant growth by producing phytohormones, such as auxins (IAA), gibberellins and cytokinins, as well as specific amino acids. Furthermore, these bacteria have a high capacity for phosphorus solubilization and are able to produce siderophores ([17]).
Despite the well-known positive effects of P. fluorescens on plant survival ([30]), only a small number of studies have examined its influence on the growth of forest species ([22], [15]). Some of these effects have been observed specifically in P. halepensis inoculated with P. fluorescens Aur6 ([26]). Recently, we showed that inoculation of Aleppo pine with P. fluorescens CECT 844 improved both its vegetative growth and N uptake ([9]).
It has been demonstrated that the frequent use of chemical fertilizers in nurseries leads to soil contamination, as chemical fertilizers are not completely assimilated by the seedlings. Furthermore, the industrial practices necessary for the chemical synthesis of compounds containing inorganic nitrogen require the use of contaminating petroleum products, high temperatures and high pressures. Considering these issues, the use of natural, “environmentally friendly” microbial inoculums, such as PGPR rhizobacteria or mycorrhizal fungi, as alternative fertilizers can be considered as a potential future solution. These microorganisms are also beneficial for the maintenance of pre-existing soil microflora, contributing to the conservation of biodiversity. The amended soil eventually enhances the vegetative vigor and the morphophysiological attributes of the forest species grown in nurseries for reforestation purposes ([7]).
Previous studies examining the effects of chemical fertilization of P. halepensis in nurseries and the trees’ response to transplantation are scarce ([25]). Of the many chemical fertilization methods used on forest seedlings, the “controlled-released fertilization” strategy is widely employed ([21]). The use of controlled-release fertilizers is an effective method that induces an increase in pine growth during the early years after planting ([6]). Although fertilization treatments may negatively impact diversity or special soil microbial communities ([11], [1]), some authors have suggested that controlled-release fertilizers and organic fertilizers could promote the growth of soil-culturable bacteria and IAA-producing bacteria. These may be one of the reasons why organic and controlled- release fertilizers promote crop growth ([38]).
The combined effect of controlled-release fertilizer with other treatments (e.g., rhizobacteria such as Pseudomonas sp.) has yet to be explored, both in forest seedlings and in transplanted trees. In this study, we hypothesized that the combination of controlled-release fertilizers and P. fluorescens CECT 844 inoculation in the nursery would have a synergistic effect on seedling growth, improving the morphophysiological attributes of P. halepensis seedlings. To explore this hypothesis, growth and water parameters, nutrient uptake and root growth potential of P. halepensis seedlings were investigated.
Methods
Plant material
Assays were performed using P. halepensis seeds collected from Almería, Spain. The seeds were maintained in polyethylene bags stored at 4 °C until sowing. In mid-March 2010, Forest Pot 300® trays (Nuevos Sistemas de Cultivo S.L., Girona, Spain) with 50 alveoli were filled with a 3:1 (v:v) mixture of peat:vermiculite at pH 6. The peat was previously sterilized in an autoclave at 120 °C for 2 hours. Subsequently, a slow-release solid fertilizer was added to the substrate in half of the trays as a treatment, and a dose of 2 g per L was homogenized with the prepared substrate.
Seeds were selected by flotation and immersed in water 24 hours before sowing. Immediately prior to sowing, the seeds were immersed in 30% H2O2 for 15 minutes for disinfection and subsequently washed several times in distilled water. Assays were performed after sowing seeds into a total of 16 bins (800 alveoli); half of these contained fertilizer substrates. A total of 3 to 8 seeds were placed in each alveolus, and after germination, one seedling was left in each alveolus. Sowing was performed in the greenhouse in March 2010; plants remained in the saturated soil that was irrigated daily; the temperature of the greenhouse was maintained between 20 and 30 °C prior to inoculation.
Bacterial cultures
The lyophilized P. fluorescens strain CECT 844 (Spanish Type Culture Collection, Valencia University, Spain) was pre-incubated in liquid medium suitable for the growth of this bacterium (1 g meat extract, 2 g yeast extract, 5 g peptone, 5 g NaCl and 1 L distilled water; pH 7.2) at 28 °C with shaking at 200 rpm for 12 h. A second culture was grown at 30 °C for 12 h before the inoculation. The final concentration of the inoculum was 3·107 CFU ml-1 (estimated by plate counting).
Experimental design
The fertilizer used contained 16% nitrogen (6.4% nitric nitrogen, 8.5% ammoniacal nitrogen, 1.1% urea nitrogen), 11% P2O5 (soluble in neutral ammonium citrate, 8.3% soluble in water) and 10% K2O (2% total magnesium oxide - MgO), with an estimated release time of 12 to 14 months at 21 °C.
We used a four-level univariate design: control (C), fertilized (F), inoculated (Ps), and fertilized + inoculated (F x Ps) treatments distributed into 4 randomized blocks (1 x 4 x 4). Each block contained 50 plants per treatment (200 plants per block). The P. fluorescens CECT 844 inoculum was applied in two steps separated by 15 days (June 24th and July 7th, 2010). The seedlings were inoculated by injecting a total inoculum of 10 mL (5 + 5 mL, 3 x 108 CFU per plant) into the substrate, near the root system. After the second inoculation, the plants were removed from the greenhouse and watered daily until saturation. The HOBO® Data Logger was used to measure the temperature and relative humidity. The temperature fluctuated between 11 and 29 °C and the relative humidity ranged from 28 to 80% until the time at which plants were analyzed.
Pressure-volume curves and water parameter analysis
During November 2010, pressure-volume (PV) curves were constructed according to the methods described by Tyree & Hammel ([34]) and Robichaux ([27]) using stem water potential measurements obtained in a Scholander pressure chamber ([29]). Eight randomly chosen plants were analyzed per treatment. From each PV curve, three water parameters were calculated: osmotic potential at saturation (Ψπfull), osmotic potential at turgor loss point (Ψπ0) and modulus of elasticity (Emax - [8], [12], [35], [4]).
Growth
In November 2010, eight plants per treatment were randomly selected for measuring height and diameter. Subsequently, the shoots and roots were dried (65 °C, 48 h) for dry weight (DW) determination.
Nutrient analysis
In late November, the concentrations and contents of key nutrients (N, P, K, Ca, Mg and Fe) in the shoots and roots of seedlings were analyzed. In each block, 12 seedlings per treatment were collected and grouped into one sample. Next, the aerial parts and roots of each sample were separated, cleaned and dried in an oven at 65-70 °C for at least 48 hours. The tissues were finely ground and homogenized. Analyses of P, K, Ca, Mg and Fe were performed by inductively coupled optical emission spectrophotometry (ICP-OES) after digestion of subsamples in a closed microwave system using concentrated HNO3. The N concentration was determined using a mass analyzer (LECO CHN-600) according to the manufacturer’s instructions.
Root growth potential
Root growth potential (RGP) was determined by randomly sampling 20 new plants per treatment (5 per block) on February 21st, 2011. The height and basal diameter of each plant were measured. Subsequently, each plant along with its root ball was carefully transplanted to a 3-L prismatic pot filled with inert white perlite. The pots were randomly arranged in the greenhouse at the ETSI Montes under optimal growth conditions ([31]). Irrigation was applied daily, and the air temperature was maintained between 10 and 27 °C with a relative humidity between 80 and 95%. One month later, each plant was carefully removed from the soil, and the number and length of new roots greater than 1 cm in length were determined for each plant.
Data analysis
All statistical analyses were performed by the use of the software package StatGraphics Plus® (StatPoint Technologies, Inc.). A one-way analysis of variance (ANOVA) and Duncan’s mean comparison test were performed for the proposed parameters at α = 0.05. In the case of non-homogeneous variance, a nonparametric Kruskal-Wallis test was applied. For statistical analysis of RGP, the height and diameter were selected as covariates. Similar results were obtained regardless the selected covariate; thus, the results presented here are those obtained using the the diameter as covariate.
Additionally, for each treatment data were expressed as percentage increases with respect to the control. Both one-way ANOVA and Duncan’s mean comparison test were performed to analyze these increases at α = 0.05. In the case of non-homogeneous variance, a nonparametric Kruskal-Wallis test was applied. Graphs displayed below show the most highly significant results.
Results
For the unfertilized seedlings, inoculation of P. fluorescens (Ps) resulted in significantly lower osmotic potentials (in absolute value) at both Ψπfull (0.85 ± 0.05 MPa in inoculated plants vs. 1.18 ± 0.10 MPa in control plants) and Ψπ0 (1.36 ± 0.10 MPa in inoculated plants vs. 1.75 ±0.08 MPa in control plants); however, this decrease was not observed when the plants were fertilized (F and F x Ps). For non-inoculated seedlings, fertilization (F) significantly increased the modulus of elasticity (i.e., decreased the elasticity of the cell walls), whereas inoculation with P. fluorescens did not significantly affect the elasticity (Tab. 1).
Tab. 1 - Water relations of Pinus halepensis seedlings. (F): controlled-release fertilization; (Ps): Pseudomonas fluorescens inoculation; (C): control. (Ψπfull): osmotic potential at full turgor; (Ψπ0): osmotic potential at zero turgor; (Emax): modulus of elasticity near full turgor. Mean values and standard errors (n=8) are reported. Values in the same column with different letters differ significantly (p<0.05) according to the Duncan’s test.
Treatment | Ψπfull (MPa) | Ψπ0 (MPa) | Emax(MPa) |
---|---|---|---|
C | -1.18 ± 0.10 a | -1.75 ± 0.08 a | 1.67 ± 0.65 b |
F | -0.99 ± 0.072 ab | -1.51 ± 0.08 ab | 9.80 ± 1.21 a |
Ps | -0.85 ± 0.05 b | -1.36 ± 0.10 b | 5.52 ± 1.74 ab |
F x Ps | -1.05 ± 0.07 ab | -1.53 ± 0.11 ab | 12.60 ± 2.74 a |
The F and Ps amendments significantly increased the morphological parameters, with the exception of the effect of Ps on root dry weight and height in unfertilized seedlings (Tab. 2). However, when the treatments were combined, we observed an additive increase in the beneficial effects on plant growth compared with the independent treatments (Fig. 1); these effects were the most significant for height and root dry weight of seedlings.
Tab. 2 - Morphological parameters of Pinus halepensis seedlings. (F): controlled-release fertilization; (Ps): Pseudomonas fluorescens inoculation; (C): control; (H): height; (D): basal diameter; (SDW): shoot dry weight; (RDW): root dry weight. Mean values and standard errors (n=8) are reported. Values in the same column with different letters differ significantly (p<0.05) according to the Duncan’s test.
Treatment | H (cm) | D (mm) | SDW (g) | RDW (g) |
---|---|---|---|---|
C | 6.13 ± 0.26 c | 1.03 ± 0.03 c | 0.074 ± 0.006 c | 0.068 ± 0.008 b |
F | 21.81 ± 0.86 b | 1.96 ± 0.08 a | 0.710 ± 0.078 a | 0.378 ± 0.063 a |
Ps | 7.66 ± 0.66 c | 1.30 ± 0.08 b | 0.155 ± 0.026 b | 0.107 ± 0.018 b |
F x Ps | 25.40 ± 0.64 a | 2.15 ± 0.08 a | 0.899 ± 0.054 a | 0.481 ± 0.087 a |
Fig. 1 - Increased height (A), diameter (B), shoot dry weight (C) and root dry weight (D) of Pinus halepensis seedlings. (Δ): increase (%); (F): controlled-release fertilization; (Ps): Pseudomonas fluorescens inoculation; (F x Ps): controlled-release fertilization and P. fluorescens inoculation treatment. Bars represent the standard error (n=8). Different letters indicate significant differences among means (p<0.05) after Duncan’s test.
F treatment significantly increased the concentrations of N, P and K but reduced the concentrations of Ca, Mg and Fe in the shoots (Tab. 3). In unfertilized seedling shoots, Ps increased the N concentration (8.90 ± 0.25 mg g-1 in inoculated plants vs. 5.32 ± 0.16 mg g-1 in the control) but decreased the concentrations of P (0.40 mg g-1 in inoculated plants vs. 0.65 ± 0.05 mg g-1 in the control), Ca (3.50 ± 0.13 mg g-1 in inoculated plants vs. 4.82 ± 0.17 mg g-1 in the control), Mg (2.45 ± 0.12 mg g-1 in inoculated plants vs. 3.22 ± 0.10 mg g-1 in the control) and Fe (0.072 ±0.004 mg g-1 in inoculated plants vs. 0.095 ±0.004 mg g-1 in the control). Additionally, F significantly increased the N, P, K and Fe concentrations in the roots (Tab. 3).
Tab. 3 - Nutrient concentrations in the shoots and roots of Pinus halepensis seedlings. (F): controlled-release fertilization; (Ps): Pseudomonas fluorescens inoculation; (C): control. Values in parentheses represent the standard error (n=4). Values in the same column with different letters differ significantly (p<0.05) according to Duncan’s test.
Portion | Treatment | N (mg/g) | P (mg/g) | K (mg/g) | Ca (mg/g) | Mg (mg/g) | Fe (mg/g) |
---|---|---|---|---|---|---|---|
Shoot | C | 5.32 ± 0.16 c | 0.65 ± 0.05 b | 8.32 ± 0.51 b | 4.82 ± 0.17 a | 3.22 ± 0.10 a | 0.095 ± 0.004 a |
F | 16.47 ± 0.81 a | 2.17 ± 0.10 a | 11.10 ± 0.23 a | 3.25 ± 0.13 b | 2.07 ± 0.02 c | 0.062 ± 0.008 b | |
Ps | 8.90 ± 0.25 b | 0.4 ± 0 c | 6.90 ± 0.64 b | 3.5 ± 0.13 b | 2.45 ± 0.12 b | 0.072 ± 0.004 b | |
F x Ps | 16.45 ± 0.85 a | 2.05 ± 0.12 a | 11.07 ± 0.16 a | 3.22 ± 0.07 b | 1.92 ± 0.07 c | 0.060 ± 0.008 b | |
Root | C | 4.82 ± 0.33 b | 0.50 ± 0 b | 9.57 ± 0.29 b | 8.52 ± 0.25 a | 2.95 ± 0.14 ab | 0.55 ± 0.09 b |
F | 11.02 ± 0.59 a | 1.90 ± 0.11 a | 12.70 ± 0.41 a | 7.82 ± 0.14 a | 4.22 ± 0.55 a | 1.50 ± 0.27 a | |
Ps | 5.8 ± 0.45 b | 0.42 ± 0.02 b | 10.15 ± 0.29 b | 7.50 ± 0.16 a | 2.57 ± 0.25 b | 0.55 ± 0.12 b | |
F x Ps | 11.4 ± 1.21 a | 1.92 ± 0.16 a | 11.70 ± 0.33 a | 8.22 ± 0.87 a | 3.00 ± 0.54 ab | 0.87 ± 0.31 ab |
F treatment induced significant increases in shoot nutrient content compared to the control, whereas Ps treatment significantly affected N, K, Ca and Mg contents in shoots (Tab. 4). In unfertilized plants, inoculation with P. fluorescens resulted in an increase in K content in shoots compared with that in the control plants (from 0.62 ± 0.04 to 1.06 ± 0.10 mg plant-1). In fertilized plants, the presence of P. fluorescens induced an increase in N (from 11.70 ± 0.58 to 14.77 ± 0.76 mg plant-1), P (from 1.54 ± 0.07 to 1.84 ± 0.11 mg plant-1), K (from 7.88 ± 0.17 to 9.94 ± 0.15 mg plant-1), Ca (from 2.31 ± 0.10 to 2.90 ± 0.07 mg plant-1) and Mg (from 1.47 ± 0.02 to 1.73 ± 0.07 mg plant-1). In the roots, F and Ps treatments both significantly increased the N, K and Ca contents. In unfertilized plants, inoculation with P. fluorescens resulted in a higher Mg content (from 0.20 ± 0.01 to 0.27 ± 0.03 mg plant-1). In fertilized plants, Ps increased the P content in roots (from 0.718 ± 0.04 to 0.924 ± 0.079 mg plant-1). Additionally, F increased the Fe content in roots (Tab. 4). The combination of both treatments had an additive effect on the observed increases in all nutrients (Fig. 2); these effects were most significant for N, K, Ca and Mg contents of shoots and P, K and Ca contents of roots.
Tab. 4 - Nutrient contents of the shoots and roots of Pinus halepensis seedlings. (F): controlled-release fertilization; (Ps): Pseudomonas fluorescens inoculation; (C): control. Values in parentheses represent the standard error (n=4). Values in the same column with different letters differ significantly (p<0.05) according to Duncan’s test.
Portion | Treatment | N (mg/plant) | P (mg/plant) | K (mg/plant) | Ca (mg/plant) | Mg (mg/plant) | Fe (mg/plant) |
---|---|---|---|---|---|---|---|
Shoot | C | 0.40 ± 0.01 d | 0.049 ± 0.003 c | 0.62 ± 0.04 d | 0.36 ± 0.01 d | 0.24 ± 0.01 d | 0.007 ± 0.0003 c |
F | 11.70 ± 0.58 b | 1.54 ± 0.07 b | 7.88 ± 0.17 b | 2.31 ± 0.10 b | 1.47 ± 0.02 b | 0.044 ± 0.006 a | |
Ps | 1.37 ± 0.04 c | 0.062 ± 0 c | 1.06 ± 0.10 c | 0.54 ± 0.02 c | 0.38 ± 0.02 c | 0.011 ± 0.0006 b | |
F x Ps | 14.77 ± 0.76 a | 1.84 ± 0.11 a | 9.94 ± 0.15 a | 2.90 ± 0.07 a | 1.73 ± 0.07 a | 0.053 ± 0.007 a | |
Root | C | 0.33 ± 0.02 d | 0.033 ± 0 c | 0.65 ± 0.02 d | 0.58 ± 0.02 d | 0.20 ± 0.01 c | 0.037 ± 0.006 b |
F | 4.17 ± 0.22 b | 0.718 ± 0.040 b | 4.80 ± 0.16 b | 2.96 ± 0.05 b | 1.60 ± 0.21 a | 0.568 ± 0.104 a | |
Ps | 0.62 ± 0.05 c | 0.045 ± 0.002 c | 1.09 ± 0.03 c | 0.80 ± 0.02 c | 0.27 ± 0.03 b | 0.058 ± 0.013 b | |
F x Ps | 5.47 ± 0.58 a | 0.924 ± 0.079 a | 5.61 ± 0.16 a | 3.95 ± 0.42 a | 1.44 ± 0.26 a | 0.416 ± 0.150 a |
Fig. 2 - Increase in nutrient content of Pinus halepensis seedlings. (Δ): increase (%); (F): controlled-release fertilization; (Ps): Pseudomonas fluorescens inoculation; (F x Ps): controlled-release fertilization and P. fluorescens inoculation treatment. Bars represent the standard error (n=4). Columns with different letters differ significantly (p<0.05) according to Duncan’s test.
Finally, in the RGP test, F caused a significant increase in the number and length of the new roots, whereas Ps did not affect either of these parameters (Tab. 5).
Tab. 5 - Root growth potential of Pinus halepensis seedlings. (F): controlled-release fertilization; (Ps): Pseudomonas fluorescens inoculation; (C): control; (1): total number of new root tips/plant; (2): total length of new roots/plant (basal diameter was used as a covariate). Mean values and standard errors (n=20) are reported. Values in the same column with different letters differ significantly (p<0.05) according to Duncan’s test.
Treatment | No. Roots (1) | Length (2) (cm) |
---|---|---|
C | 3 ± 1 b | 5.19 ± 4.8 b |
F | 7 ± 1 a | 36.7 ± 4.3 a |
Ps | 1 ± 1 b | 1.8 ± 4.5 b |
F x Ps | 7 ± 1 a | 29.3 ± 5.5 a |
The examined parameters were subjected to ANOVA or Kruskal-Wallis test, and the results are presented in Tab. 6. With the exception of the osmotic potential and the Ca and Mg concentrations in roots, all other parameters were significantly affected by the treatments.
Tab. 6 - Results of the analysis of variance on the parameters considered. (1): Ψπfull: osmotic potential at full turgor; Ψπ0: osmotic potential at zero turgor; Emax: modulus of elasticity near full turgor. (2): H: height; D: basal diameter; SDW: shoot dry weight; RDW: root dry weight. (3): Number and total length of new roots per plant; basal diameter was used as a covariate.
Type | Organ | Parameters | ANOVA | Kruskal-Wallis | |||
---|---|---|---|---|---|---|---|
df | F | P value | K-W statistic |
P value | |||
Water relations parameters1 |
- | Ψπfull | 3 | 3.37 | 0.0325* | - | - |
Ψπ0 | 3 | 2.87 | 0.0543 | - | - | ||
E max | - | - | - | 16.12 | 0.0011* | ||
Growth2 | - | H | 3 | 229.97 | 0.0000* | - | - |
D | 3 | 54.5 | 0.0000* | - | - | ||
SDW | - | - | - | 26.01 | 0.0000* | ||
RDW | - | - | - | 13.73 | 0.0000* | ||
Nutrient concentration |
Shoot | N | - | - | - | 12.75 | 0.0052* |
P | - | - | - | 13.21 | 0.0042* | ||
K | - | - | - | 12.02 | 0.0073* | ||
Ca | 3 | 32.83 | 0.0000* | - | - | ||
Mg | - | - | - | 13.52 | 0.0036* | ||
Fe | 3 | 5.87 | 0.0105* | - | - | ||
Root | N | 3 | 22.1 | 0.0000* | - | - | |
P | 3 | 70.93 | 0.0000* | - | - | ||
K | 3 | 18.1 | 0.0001* | - | - | ||
Ca | - | - | - | 4.25 | 0.2360 | ||
Mg | 3 | 3.05 | 0.0698 | - | - | ||
Fe | 3 | 4.13 | 0.0317* | - | - | ||
Nutrient content |
Shoot | N | - | - | - | 13.81 | 0.0032* |
P | - | - | - | 14.10 | 0.0028* | ||
K | - | - | - | 14.14 | 0.0027* | ||
Ca | - | - | - | 14.20 | 0.0026* | ||
Mg | - | - | - | 14.24 | 0.0026* | ||
Fe | - | - | - | 12.90 | 0.0048* | ||
Root | N | - | - | - | 13.79 | 0.0032* | |
P | 3 | 105.81 | 0.0000* | - | - | ||
K | - | - | - | 14.14 | 0.0027* | ||
Ca | - | - | - | 14.12 | 0.0027* | ||
Mg | - | - | - | 12.73 | 0.0053* | ||
Fe | - | - | - | 12.04 | 0.0072* | ||
Root growth potential3 |
- | N° Roots | 3 | 9.94 | 0.0000* | - | - |
Length | 3 | 12.65 | 0.0000* | - | - |
Discussion
The parameter RGP is widely used for the characterization of the physiological quality of seedlings ([31]). Controlled-release fertilizer has been shown to improve RGP ([20]), as observed in this study. Although there is a possible relationship between carbohydrate reserves and RGP in some forest species, the relationship between rooting ability and carbohydrate reserve content in P. halepensis has not been well studied ([33]). Additionally, very recent results indicate that RGP appears to be more closely related to N concentration ([36]).
In this study, we showed that the P. fluorescens CECT 844 isolate did not affect root initiation capacity (RGP test) in P. halepensis seedlings, which is in agreement with previous results ([9]). It should be noted that the root total dry weights of seedlings increased slightly with respect to the control due to Ps inoculation; however, these results were not significant. The RGP results we obtained with P. fluorescens CECT 844 do not agree with the observations made in previous studies using other P. fluorescens strains ([13]). Such discrepancy suggests that the effect on root regeneration might depend on the particular P. fluorescens strain used. Additionally, it might be beneficial to study root growth potential over longer periods in order to detect more patent effects of the treatments.
Although we did not observe any synergistic effects of the combined F x Ps treatment on the regeneration of new roots, each of the independent treatments increased the growth parameters; obviously, these increases were more apparent in the case of F compared with Ps. We previously reported the positive effect of the CECT 844 P. fluorescens strain on the growth of P. halepensis seedlings ([9]); however, height and RDW were improved in the aforementioned study, whereas in the present work, diameter and SDW were improved. In this investigation, a positive interaction between P. fluorescens and F at relatively low doses was observed in terms of growth; although F masked the effect of Pf on diameter and SDW, F enhanced the effect of Pf on height.
Analogously, F increased the concentrations of N, P and K (Tab. 3) as well as the contents of most nutrients in both shoots and roots (Tab. 4). Oliet et al. ([19]) reported strong correlations between the doses of controlled-release fertilizer applied to seedlings in a P. halepensis nursery and shoot concentrations of N, P and, more weakly, K. In the present work, the fertilizer used (16 + 11 + 10) appears to have more balanced proportions of N, P and K than those used by Oliet et al. ([19]); thus, significant increases in the N, P and K concentrations compared to the control were obtained using a relatively low dose of 2 g per L.
Rincón et al. ([26]) reported that P. halepensis seedlings inoculated with the P. fluorescens strain Aur6 showed increased P and K concentrations following inoculation in the presence of local bacterial colonies, whereas in the absence of such colonies, the inoculation with P. fluorescens Aur6 increased the concentrations of N, Mg and Mn. Previously, we found that P. fluorescens CECT 844 increased the N concentration and decreased the P concentration in P. halepensis seedlings ([9]). Using the same strain, very similar results were obtained in this study only for the shoot tissue; additionally, we observed a significant decrease in shoot Ca and Mg concentrations, which is in agreement with our previous report.
It should be noted that in Mediterranean areas, P is a limiting nutrient during the early stages of P. halepensis growth ([28]). In this study, due to Ps inoculation, the P concentration in the shoots of unfertilized seedlings decreased. The P content remained unchanged with respect to the control, whereas it increased in the combined treatment (F x Ps). When plants were grown on a nutrient-poor culture substrate (e.g., peat), the addition of P (soluble and insoluble) via F could provide more evidence for a possible P-solubilizing effect of Ps. Such results confirm our previous findings obtained under identical conditions ([9]), strenghtening the evidence that the observed decrease in P concentration is not always correlated with a decrease in P uptake. For example, in non-fertilized seedlings, the P concentration might decrease as a result of dilution due to the biomass increase caused by Ps. Additionally, the combined F x Ps treatment did not improve the macronutrient concentration compared with the independent treatments, although the contents of most macronutrients were additively increased (Fig. 2).
The adjustment of the osmotic potential and the capacity to increase the cell wall elasticity (elastic adjustment) are essential for plants to withstand the water stress. Cell osmotic potential and elasticity regulation allow plants to maintain turgor potential, thereby improving the capacity for growth and photosynthesis as well as the ability to tolerate more negative water potentials and lower water availability ([36]). It has been suggested, however, that rigid cell walls may be more conducive to cell survival and to the maintenance of tissue integrity necessary for recovery and rehydration after a drought stress period. This observation has been made principally in species able to make osmotic adjustments through the accumulation of large amounts of organic solutes ([23]).
According to previous studies ([10]) we observed that under optimal water conditions, Ps caused a decrease in the absolute value of the osmotic potential (i.e., a negative effect on the maintenance of cell turgor caused by a possible decrease in the cellular concentration of organic solutes). However, the addition of F could increase the concentration of dissolved solutes and thus dampen the effect of Ps, even at low doses. Osmotic forces result from local differences in water potentials created largely by gradients in the concentrations of dissolved solutes ([14]). K is an important solute associated with the regulation of cell turgor, water economy and stomatal opening ([2]). Thus, according to our results, there was no clear relationship between the regulation of cellular osmotic potential and the K concentration in the seedlings; although this element was increased due to F, no significant osmotic adjustment was detected in seedlings due to F or F x Ps treatments.
It was observed that F caused a significant increase in the modulus of elasticity (i.e., an increase in the stiffness of cell walls), as F likely accelerated the process of lignification of the cell walls. We also observed that Ps did not have a similar effect. In fact, Ps has been reported to increase cell wall elasticity ([10]). Changes in the wall stiffness may be partially related to cellular structural changes induced by the plant defense response to bacterial flagellin. Plant detection of flagellin has been shown to induce stomatal closure ([18]) and modifications of the protein content of the cell wall ([37], [32]). However, the effect of P. fluorescens on cell elasticity is not clear. Further detailed analyses are required on the effects of Ps and F treatments on cell elasticity and osmotic potential under drought or other abiotic stress conditions.
Conclusion
We conclude that a combined treatment including controlled-release fertilization (F) and Pseudomonas fluorescens CECT 844 inoculation (Ps) had a net positive effect on P. halepensis seedling growth and nutrient uptake; the negative effects observed as a result of Ps treatment alone were dampened by the effects of F.
The use of chemical fertilizers as agroforestry crop treatments in nurseries may cause environmental problems. Thus, the inclusion of P. fluorescens, a plant growth-promoting rhizobacterium, in soils with low microbial activity might be an interesting solution, regardless of its effects on the morphophysiology of the host plant. Although the capacity of the combined F x Ps treatment for maintaining and improving the soil microbiota is currently under study, our results show that F x Ps is a highly efficient alternative for improving the quality of forest seedlings in nurseries. As such, F x Ps represents a promising potential alternative treatment that could reduce contaminant emissions and increase microbiota soil regeneration in degraded soils.
List of abbreviations
The following abbreviations have been used throughout the paper:
- F: Controlled-release fertilization
- Ps: P. fluorescens inoculation
- F x Ps: Controlled-release fertilization and P. fluorescens inoculation treatment
- PGPR: Plant growth-promoting rhizobacteria
- CFU: Colony forming units
- PV: Pressure-volume
- Ψπfull: Osmotic potential at saturation
- Ψπ0: Osmotic potential at turgor loss point
- Emax: Modulus of elasticity
- ICP-OES: Inductively coupled optical emission spectrophotometry
- RGP: Root growth potential
Acknowledgments
This work was funded as part of project A/025191/09, “Use of inoculants for sustainable agricultural and forestry production systems,” by the PCI-AECID (Program of Cooperation between Universities and Scientific Research between Spain and Latin America; Spanish International Cooperation Agency for Development).
References
Gscholar
Gscholar
CrossRef | Gscholar
Gscholar
Gscholar
CrossRef | Gscholar
CrossRef | Gscholar
Gscholar
CrossRef | Gscholar
Authors’ Info
Authors’ Affiliation
Daniel Delgado-Alvez
Marta Berrocal-Lobo
E.T.S.I Montes & E.U.I.T Forestal, Polytechnic University of Madrid, Av/da Ciudad Universitaria s/n, E-28040 Madrid (Spain)
Ada Albanesi
Faculty of Agronomy & Agroindustries, National University of Santiago del Estero, Av/da Belgrano (S) 1912, 4200 Santiago del Estero (Argentina)
Corresponding author
Paper Info
Citation
Dominguez-Nuñez JA, Delgado-Alvez D, Berrocal-Lobo M, Anriquez A, Albanesi A (2015). Controlled-release fertilizers combined with Pseudomonas fluorescens rhizobacteria inoculum improve growth in Pinus halepensis seedlings. iForest 8: 12-18. - doi: 10.3832/ifor1110-007
Academic Editor
Roberto Tognetti
Paper history
Received: Aug 20, 2013
Accepted: Dec 26, 2013
First online: May 12, 2014
Publication Date: Feb 02, 2015
Publication Time: 4.57 months
Copyright Information
© SISEF - The Italian Society of Silviculture and Forest Ecology 2015
Open Access
This article is distributed under the terms of the Creative Commons Attribution-Non Commercial 4.0 International (https://creativecommons.org/licenses/by-nc/4.0/), which permits unrestricted use, distribution, and reproduction in any medium, provided you give appropriate credit to the original author(s) and the source, provide a link to the Creative Commons license, and indicate if changes were made.
Web Metrics
Breakdown by View Type
Article Usage
Total Article Views: 47074
(from publication date up to now)
Breakdown by View Type
HTML Page Views: 40039
Abstract Page Views: 2410
PDF Downloads: 3519
Citation/Reference Downloads: 18
XML Downloads: 1088
Web Metrics
Days since publication: 3729
Overall contacts: 47074
Avg. contacts per week: 88.37
Article Citations
Article citations are based on data periodically collected from the Clarivate Web of Science web site
(last update: Feb 2023)
Total number of cites (since 2015): 3
Average cites per year: 0.33
Publication Metrics
by Dimensions ©
Articles citing this article
List of the papers citing this article based on CrossRef Cited-by.
Related Contents
iForest Similar Articles
Research Articles
The combined effects of Pseudomonas fluorescens CECT 844 and the black truffle co-inoculation on Pinus nigra seedlings
vol. 8, pp. 624-630 (online: 08 January 2015)
Research Articles
Linking nursery nutritional status and water availability post-planting under intense summer drought: the case of a South American Mediterranean tree species
vol. 9, pp. 758-765 (online: 03 June 2016)
Research Articles
Conservation of Betula oycoviensis, an endangered rare taxon, using vegetative propagation methods
vol. 13, pp. 107-113 (online: 23 March 2020)
Research Articles
Combined pre-hardening and fall fertilization facilitates N storage and field performance of Pinus tabulaeformis seedlings
vol. 9, pp. 483-489 (online: 07 January 2016)
Research Articles
Forest litter as the mulch improving growth and ectomycorrhizal diversity of bare-root Scots pine (Pinus sylvestris) seedlings
vol. 8, pp. 394-400 (online: 20 August 2014)
Research Articles
Substrates and nutrient addition rates affect morphology and physiology of Pinus leiophylla seedlings in the nursery stage
vol. 10, pp. 115-120 (online: 02 October 2016)
Technical Reports
Nursery practices increase seedling performance on nutrient-poor soils in Swietenia humilis
vol. 8, pp. 552-557 (online: 09 December 2014)
Research Articles
Use of alternative containers for promoting deep rooting of native forest species used for dryland restoration: the case of Acacia caven
vol. 10, pp. 776-782 (online: 02 September 2017)
Short Communications
The effects of salicylic acid, oxalic acid and chitosan on damping-off control and growth in Scots pine in a forest nursery
vol. 13, pp. 441-446 (online: 24 September 2020)
Research Articles
Nursery fertilization affected field performance and nutrient resorption of Populus tomentosa Carr. ploidy levels
vol. 15, pp. 16-23 (online: 24 January 2022)
iForest Database Search
Google Scholar Search
Citing Articles
Search By Author
Search By Keywords