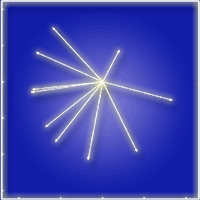
Successional leaf traits of monsoon evergreen broad-leaved forest, Southwest China
iForest - Biogeosciences and Forestry, Volume 10, Issue 2, Pages 391-396 (2017)
doi: https://doi.org/10.3832/ifor2045-009
Published: Mar 16, 2017 - Copyright © 2017 SISEF
Research Articles
Abstract
Understanding the variation of functional traits of plant species along forest successional gradients may provides useful insights into community assemblages. However, species performance during forest succession is controversial. We explored the variation of leaf traits along a forest succession by examining ten leaf traits in four successional stages in a monsoon evergreen broad-leaved forest in Southwest China. Results showed significant differences in all leaf traits except leaf area and leaf carbon content among the successional stages. Five leaf traits were highly correlated to successional stage, while the first principal component showed no correlation with successional stage. The first principal component accounted for 56.1% of the total variation in all ten leaf traits. Almost 50% of the relationships between leaf traits differed along the examined successional gradient, indicating that leaf traits were affected by the successional stage.
Keywords
Specific Leaf Area, Leaf Chemistry, Maximum Photosynthesis, Successional Stage, Monsoon Evergreen Broadleaved Forest
Introduction
Plant traits are biological characteristics of plant species that respond to the dominant processes in an ecosystem ([23]). Plant traits are defined characteristics that relate to plant species’ patterns of establishment, growth and resource allocation. This includes plant evolutionary response to abiotic conditions and interactions with other species ([7], [30]). Therefore, plant traits strongly influence plant fitness and performance ([26]). Plant traits can also be used as indicators of the mechanisms by which species affect ecosystem properties and processes ([31]). For example, specific leaf area (SLA), one of the most widely accepted key traits, has been proven to strongly correlate with relative growth rate ([32]) and was one of the major contributors to an axis of resource capture, usage and availability. Leaf nitrogen (Nmass) and phosphorus (Pmass) concentrations can reflect plant and soil nutrient status, and influence vegetation composition ([15]). Leaf Nmass to Pmass ratios are determined by the uptake and loss of N and P and are useful in assessing N versus P limitation to primary production in terrestrial ecosystems ([17]). Plant traits provide important information about ecological processes and improve our understanding of the mechanisms behind those processes ([13]), especially forest succession ([20]).
The advantage of utilizing plant traits is that different vegetation types can be directly compared with specific processes, resulting in the extraction of general trends ([8]). This allows the prediction of vegetation dynamics by using plant trait responses ([8]). Furthermore, plant trait responses provide insight into the mechanisms underlying successional processes and the basis for thorough experimental studies on those mechanisms involved. Trait-based studies have been used successfully to evaluate complex ecosystem responses to human disturbance occurring in grasslands ([27]) and forest ecosystems ([3]).
The aim of this study is to determine how plant traits relate to successional stages and how plant traits change throughout succession. We selected the 20 most abundant plant species in four successional stages of 15, 30, 40-yr-old forest and old-growth forest (older than 80 years) of monsoon evergreen broad-leaved forests in Southwest China. Ten morphological and physiological leaf traits were used to evaluate variations and correlations between successional stages. We asked two main research questions: (1) are different leaf traits related to different successional stages? (2) If so, how do these relationships change with successional stage?
Materials and methods
Study sites
The study was conducted in a monsoon evergreen broad-leaved forest in the Taiyang river provincial nature reserve (22° 32′ - 22° 37.8′ N, 101° 2.6′ - 101° 18′ E) and the forest farm of the Vocational Education center of Pu’er (22° 43.2′ - 22° 45′ N, 100° 55.8′ - 100° 57′ E) in the Pu’er region, Yunnan Province, China. The elevation of Taiyang river provincial nature reserve ranges from 585 to 1707 m a.s.l., with a mean annual temperature (from 2000 to 2010) of 17.7 °C and mean annual rainfall (from 2000 to 2010) of 1535 mm, which occurs mainly between May and October. The elevation of the forest farm of the Vocational Education center of Pu’er ranges from 1142 to 1468 m a.s.l., with a mean annual temperature (from 2000 to 2010) of 17.7 °C and mean annual rainfall (from 2000 to 2010) of 1535 mm which occurs mainly between May and October. The monsoon evergreen broad-leaved forest is the dominant vegetation type in the two study sites. However, parts of the forest in the forest farm of the Vocational Education center of Pu’er were logged in the 20th century, resulting in four successional stages: 15, 30, 40-yr-old forest and old-growth forest (>80 years). The 15-yr-old forest has a high stand density of relatively small trees. Lithocarpus fenestratus, Helicia nilagirica, Litsea cubeba, Castanopsis echinocarpa and Schimawallichii were the main canopy trees. The dominant understory species were Canthium horridum, Dalbergia rimosa, Scleria herbecarpa and Pteridophyta. In the 30 and 40-yr-old forest, stand density was lower compared with the 15-yr-old forest. The mean plant height was higher with numerous trees diameter at breast height (DBH) > 20 cm. The main canopy trees included C. echinocarpa, L. fenestratus, Aporusa villosa, Machilus robusta and C. hystrix. The dominant understory species were Canthium horridum, Ficus hirta and Pteridophyta. In the old-growth forest, the dominant tree species were C. echinocarpa and C.hystrix, with the understory containing Ardisia maculosa, Fordia microphylla, S. herbecarpa and Pteridophyta. Pteridophyta and Orchidaceae grow epiphytically on stems or the forest canopy occurring frequently in all the surveyed forest types. In addition, rich buttressed trees and lianas formed an important characteristic of monsoon evergreen broad-leaved forests in the Pu’er region.
Data collection
Three 30 × 30 m plots were established in each of four successional stages, for a total of twelve plots. Each plot was located in a representative area of each successional stage with a minimum distance of 100 m from any major trail. The minimum distance between any two plots was 200 m. All plots were established on similar terrain. Each plot was divided into thirty-six 5 × 5 m subplots. All living stems >1 cm diameter (DBH) in the subplots were tagged, identified to species, numbered, and their DBH and height were recorded. In each plot we sampled ten individual trees per species taking two leaves from each individual tree. The 20 most abundant species were sampled. This resulted in more than 70% of the total individuals in each successional stage (15-yr-old forest: 72.4%; 30-yr-old forest: 83.6%; 40-yr-old forest: 83.5%; old-growth forest: 74.2%). Five soil samples were collected from each plot and used to analyze the physical and chemical soil properties (Tab. 1). Elevation and slope of each plot were also recorded (Tab. 1).
Tab. 1 - Environmental factors of the four successional stages. (N): soil nitrogen contents (g kg-1); (P): soil phosphorus contents (g kg-1); (C): soil carbon contents (g kg-1). Different letters in the same row indicate significant differences after Bonferroni’s post-hoc test (p<0.05).
Leaf traits | Successional stages | |||
---|---|---|---|---|
15-yr-old | 30-yr-old | 40-yr-old | Old-growth | |
Elevation (m) | 1612 ± 2 a | 1369 ± 5 b | 1349 ± 5 b | 1383 ± 123 ab |
Slope (deg) | 20.0 ± 1.2 a | 19.7 ± 3.9 a | 18.3 ± 4.4 a | 18.3 ± 6.0 a |
N (g kg-1) | 2.07 ± 0.14 a | 1.03 ± 0.13 b | 1.33 ± 0.11 bc | 1.99 ± 0.37 ac |
P (g kg-1) | 0.35 ± 0.00 ab | 0.23 ± 0.02 a | 0.30 ± 0.03 a | 0.42 ± 0.06 b |
C (g kg-1) | 49.8 ± 3.9 a | 31.2 ± 3.2 b | 30.1 ± 3.4 b | 45.8 ± 8.3 ab |
pH | 4.54 ± 0.06 a | 4.82 ± 0.08 a | 4.80 ± 0.10 a | 5.09 ± 0.25 a |
Soil water content (%) | 24.4 ± 4.18 a | 27.6 ± 2.94 a | 27.2 ± 2.18 a | 32.2 ± 0.89 b |
Ten easy-to-measure leaf traits were selected to reflect the processes of forest dynamics. Three traits were related to energy (LA: leaf area; Amass: maximum photosynthesis based on mass; Aarea: maximum photosynthesis based on area), five traits were related to nutrient (Nmass: leaf N content based on mass; Pmass: leaf P content based on mass; Nmass: Pmass; Narea: leaf N content based on area; Parea: leaf P content based on area), and two traits were linked to plant growth (SLA; LCC: leaf C content). LA was measured with a portable area meter (Li-Cor 3000A®, Lincoln, NE, USA). Leaves were dried at 70 °C to a constant weight ([2]) and weighed (dry leaf weight, DLW) for SLA determination (SLA = LA/DLW). All leaves from the same species in the same plot were pooled for chemical analysis. Nmass was measured using an elemental analyzer (NCS2500®, Carlo Erba Instruments, Milan, Italy). Pmass was determined using inductively coupled plasma emission spectroscopy (Southwest Forestry University, China). The ratio of Nmass: Pmass was also calculated. LCC was determined using an elemental analyzer (model EA 1108®, Carlo Erba Instruments, Milan, Italy). Area- and mass-based traits can be inter-converted via SLA (for example, Narea = Nmass/SLA, Parea = Pmass/ SLA - [40]), which can be used to estimate Amass and Aarea. Amass was estimated from SLA and Nmass, while Aarea was estimated using SLA and Narea, using the multiple regression model proposed by Wright et al. ([40]).
Data analysis
We first calculated the means of LA and SLA for each species in each successional stage and then analyzed these mean values using one-way analyses of variance (ANOVA). Differences in leaf traits and the physical and chemical properties of soil for each successional stage were tested with ANOVA. Further analyses using Bonferroni’s post-hoc tests (Student-Newmans-Keuls) were performed where required. Dependent variables were log10 transformed where necessary to conform to homoscedasticity assumptions.
For multivariate analyses we used the indirect ordination method. Principal Components Analysis (PCA) was conducted on the leaf traits using the software PC Ord® ver. 5.02 (MJM Software Design, Gleneden Beach, OR, USA). To detect relationships between successional stage and leaf traits we applied the Spearman’s correlation analysis with the leaf traits and PC1 and PC2. Regression analysis was conducted when a significant correlation occurred.
Standardized major axis (SMA) analysis was used to describe the relationship between each possible pairwise combination of leaf traits. SMA has become a standard procedure in leaf trait studies where bivariate relationships are quantified between variables that are measured with error ([35]). Our aim was to estimate the line of best-fit describing the bivariate scatter of two traits. SMA regression is used to estimate lines with greater precision than major axis regression ([35]). On log-log axes, SMA regression describes the best-fit scaling relationship between pairs of traits. SMA regression analyses were performed using the free software package SMATR ver. 2.0 ([11]). Other statistical computations were carried out with SPSS® ver. 17.0 (SPSS Inc., Chicago, IL, USA). All tests were carried out at a significance level of α = 0.05.
Results
Leaf traits variations with successional stages
All leaf traits except two (LA and LCC) were significantly different among successional stages. As vegetation recovered, SLA decreased (Tab. 2). Conversely, Nmass: Pmass was positively associated with forest succession (Tab. 2). The 15-yr-old forest had the highest Nmass, Amass and Pmass. The 40-yr-old forest had the lowest Nmass and Pmass. The Narea, Parea and Aarea were the highest in the old-growth forest and lowest in 30-yr-old and 40-yr-old forests (Tab. 2).
Tab. 2 - Leaf traits at the four successional stages. (LA): leaf area (cm2); (SLA): specific leaf area (m2 kg-1); (Nmass): leaf nitrogen contents (mass basis, g kg-1); (Pmass): leaf phosphorus contents (mass basis, g kg-1); (Nmass:Pmass): the ratio of leaf nitrogen contents (mass basis) to leaf phosphorus contents (mass basis); (LCC): leaf carbon contents (g kg-1); (Narea): leaf nitrogen contents (area basis, g m-2); (Parea): leaf phosphorus contents (area basis, g m-2); (Amass): maximum photosynthesis (mass basis, nmol g-1 s-1); (Aarea): maximum photosynthesis (area basis, μmol m-2 s-1). Different letters in the same row indicate significant differences after Bonferroni’s post-hoc test (p<0.05).
Leaf traits | Successional stages | |||
---|---|---|---|---|
15-yr-old | 30-yr-old | 40-yr-old | Old-growth | |
LA (cm2) | 40.6 ± 5.2 a | 48.6 ± 6.3 a | 41.5 ± 4.8 a | 44.8 ± 4.9 a |
SLA (m2 kg-1) | 21.5 ± 2.0 a | 17.6 ± 0.9 ab | 15.7 ± 0.6 ab | 15.1 ± 0.7 b |
Nmass (g kg-1) | 20.5 ± 1.5 a | 14.8 ± 0.6 b | 14.3 ± 0.6 b | 19.2 ± 1.1 a |
Pmass (g kg-1) | 1.64 ± 0.22 a | 1.04 ± 0.08 ab | 0.85 ± 0.04 b | 1.10 ± 0.04 a |
Nmass:Pmass | 14.10 ± 0.80 a | 15.10 ± 0.40 a | 17.60 ± 0.60 b | 17.80 ± 0.80 b |
LCC (g kg-1) | 465.10 ± 28.2 a | 472.70 ± 37.6 a | 465.80 ± 37.2 a | 468.90 ± 34.3 a |
Narea (g m-2) | 1.02 ± 0.29 ac | 0.79 ± 0.23 b | 0.93 ± 0.17 ab | 1.41 ± 0.67 c |
Parea (g m-2) | 0.07 ± 0.02 ac | 0.06 ± 0.02 ab | 0.05 ± 0.01 b | 0.08 ± 0.03 c |
Amass (nmolg-1 s-1) | 4.70 ± 0.04 a | 4.55 ± 0.02 bc | 4.49 ± 0.02 b | 4.61 ± 0.11 ac |
Aarea (μmol m-2 s-1) | 1.77 ± 0.02 a | 1.68 ± 0.01 b | 1.71 ± 0.01 b | 1.81 ± 0.02 a |
Correlations between leaf traits and successional stage
The PCA of the 10 measured traits captured 90.6% of the variance in two principal components, with the first axis accounting for 56.1% (eigenvalue = 2.929 - Fig. 1). The first axis mainly described resource use and was positively associated with LA, SLA and LCC and negatively associated with Narea, Nmass, Parea, Amass and Aarea. PC1 was unrelated to successional stage (Tab. 3). The second axis (accounting for 34.5% of the total variance) mainly described resource availability and was positively associated with LA, Nmass: Pmass and Aarea and negatively associated with SLA, Nmass, Pmass and Parea. PC2 was unrelated to successional stage (Tab. 3).
Fig. 1 - Principal Components Analysis (PCA) of the ten leaf traits. (LA): leaf area (cm2); (SLA): specific leaf area (m2 kg-1); (NP): the ratio of leaf nitrogen contents (mass basis) to leaf phosphorus contents (mass basis); (Nmass): leaf nitrogen contents (mass basis, g kg-1); (Pmass): leaf phosphorus contents (mass basis, g kg-1); (LCC): leaf carbon contents (g kg-1); (Narea): leaf nitrogen contents (area basis, g m-2); (Parea): leaf phosphorus contents (area basis, g m-2); (Amass): maximum photosynthesis (mass basis, nmol g-1 s-1); (Aarea): maximum photosynthesis (area basis, μmol m-2 s-1).
Tab. 3 - Correlations between leaf traits and successional stage. (LA): leaf area (cm2); (SLA): specific leaf area (m2 kg-1); (Nmass): leaf nitrogen contents (mass basis, g kg-1); (Pmass): leaf phosphorus contents (mass basis, g kg-1); (Nmass: Pmass): the ratio of leaf nitrogen contents (mass basis) to leaf phosphorus contents (mass basis); (LCC): leaf carbon contents (g kg-1); (Narea): leaf nitrogen contents (area basis, g m-2); (Parea): leaf phosphorus contents (area basis, g m-2); (Amass): maximum photosynthesis (mass basis, nmol g-1 s-1); (Aarea): maximum photosynthesis (area basis, μmol m-2 s-1).
Leaf traits | Pearson’s correlation | P |
---|---|---|
LA (cm2) | 0.063 | 0.580 |
SLA (m2 kg-1) | -0.373** | 0.001 |
Nmass (g kg-1) | -0.099 | 0.381 |
Pmass (g kg-1) | -0.338** | 0.002 |
Nmass: Pmass | 0.460** | 0.000 |
LCC (g kg-1) | 0.015 | 0.896 |
Narea (g m-2) | 0.325** | 0.003 |
Parea (g m-2) | 0.086 | 0.446 |
Amass (nmolg-1 s-1) | -0.244* | 0.029 |
Aarea (μmol m-2 s-1) | 0.202 | 0.072 |
PC1 | -0.673 | 0.327 |
PC2 | 0.704 | 0.248 |
Significant correlations were found between most leaf traits and successional stage. SLA, Pmass and Amass were strongly negatively correlated to successional stage (Tab. 3). In contrast, Nmass: Pmass and Narea were positively correlated to successional stage (Tab. 3). The best-fitting regression models of the relationships between leaf traits and the successional stage are shown in Fig. 2. However, Nmass, Parea and Aarea were unrelated to successional stage, although they showed significant differences between different stages. Likewise, LA and LCC were uncorrelated to successional stage.
Fig. 2 - Regression analysis between successional stage and leaf traits. (SLA): specific leaf area (m2 kg-1); (Nmass): leaf nitrogen contents (mass basis, g kg-1); (Pmass): leaf phosphorus contents (mass basis, g kg-1); (Narea): leaf nitrogen contents (area basis, g m-2); (Amass): maximum photosynthesis (mass basis, nmol g-1 s-1); successional stages (x-axis): (1) 15-yr-old; (2) 30-yr-old; (3) 40-yr-old; (4) Old-growth forests (>80 years).
Relationship between plant traits in different successional stages
The relationship between SLA and LA, Nmass: Pmass, and LCC were non-significant in all successional stages except for Nmass: Pmass in the 30-yr-old forest (negative association, P = 0.000) and LCC in the 15-yr-old forest (negative association, P = 0.012). The SMA slopes for the SLA - LA, SLA - Nmass: Pmass and SLA - LCC relationships were homogeneous among all successional stages (Tab. S3 in Supplementary material). SLA was positively associated with Nmass in all successional stages except the 30-yr-old forest (P = 0.100) and with Pmass in all four successional stages (P < 0.05). The SMA slopes for the SLA - Nmass and SLA - Pmass relationships were homogeneous among successional stages. SLA was negatively associated with Narea, Amass and Aarea in all successional stages (P < 0.05). The SMA slopes for the SLA - Narea, SLA - Amass and SLA - Aarea relationships were heterogeneous among successional stages, the greatest SMA slopes were in the 15-yr-old forest for the SLA - Narea and the SLA - Amass relationships and in the 40-yr-old forest for the SLA - Aarea relationships (Tab. S3 in Supplementary material). The SMA slope for the SLA - Parea relationship was homogeneous among successional stages (Tab. S3).
The SMA slope for the LA - Nmass:Pmass, LA - Nmass, LA - Pmass, LA - LCC, LA - Narea, LA - Parea and LA - Amass relationships were homogeneous among successional stages (P > 0.05), but the SMA slope for the LA - Aarea was heterogeneous among successional stages with the highest slope in the 40-yr-old forest.
The SMA slope for the Nmass:Pmass - Nmass and Nmass:Pmass - Pmass relationships were homogeneous among successional stages (P > 0.05), but the SMA slope for the Nmass: Pmass - LCC, Nmass:Pmass - Narea, Nmass:Pmass - Parea, Nmass:Pmass - Amass and Nmass:Pmass - Aarea were heterogeneous among successional stages. The 15-yr-old forest had the highest SMA slope in the SLA - Narea. In the Nmass: Pmass - LCC, Nmass:Pmass - Narea, Nmass:Pmass - Parea and Nmass:Pmass - Amass relationships, the 40-yr-old forest had the highest SMA slope in the Nmass: Pmass - Aarea relationship (Tab. S3).
The SMA slope for the LCC - Pmass, LCC - Narea, LCC - Parea, LCC - Amass, Narea - Parea, Parea - Amass and Parea - Aarea relationships were homogeneous among successional stages (P > 0.05). However, all the SMA slope for the Nmass - Pmass, Nmass - LCC, Nmass - Narea, Nmass - Parea, Nmass - Amass, Nmass - Aarea, LCC - Aarea, Pmass - Narea, Pmass - Parea, Pmass - Amass, Pmass - Aarea, Narea - Amass, Narea - Aarea and Amass - Aarea relationships were heterogeneous among successional stages (Tab. S3 in Supplementary material).
Discussion
Our analysis of a chronosequence of successional stages in a monsoon evergreen broad-leaved forest showed that species with relatively high rates of photosynthesis and leaf traits related to fast growth (high SLA) were progressively replaced by species with low SLA over time. This pattern is consistent with the shift of species along the leaf economics spectrum, as documented in other primary ([4]) and secondary successional series ([5], [13], [28], [29]). SLA is one of the most widely accepted key leaf traits ([19], [22]) and is strongly linked to relative growth rate and resource use in plants ([32]). It is also a major contributor to resource capture, usage and availability ([12], [37]). Used as a proxy for relative growth rate of a species, SLA is positively related to resource richness ([36], [38]). After mature trees are felled the priority of retaining resources decreases in forest gaps. Complex litter produced by early successional communities is shown to decompose more rapidly than litter produced by communities from more advanced successional stages ([13]). Therefore, species with high SLA should increase in abundance as resources availability increases ([20]). With vegetation succession, the fast-growing, light-demanding species are progressively replaced by slow-growing, shade-tolerant species, which tend to conserve resources more efficiently as succession proceeds. It has been shown that slow-growing, shade-tolerant species have low SLA ([12]), which may explain why SLA was the lowest in old-growth forests.
Nitrogen and phosphorus are essential nutrients and play an important role in plant growth, development and behavior. The circulation of nitrogen and phosphorus limit most terrestrial ecosystem processes ([1]). In this study Nmass, which generally co-varies with SLA ([40]), was higher in the initial and latter stages of succession likely due to the consequences of the relatively low nutrient availability in the intermediate stages ([24]). This shows that, although Nmass and SLA are positively correlated as part of the leaf economics spectrum, they can be partly decoupled, possibly to optimize Narea and Amass ([39]). Previous studies have reported variable N patterns with succession including leaf N increases consistently with succession ([9]), non-significant shifts ([28]) and slightly negative shifts during the early stages of succession ([29]). After tree felling operations, branches and leaves are usually left in situ. Decomposition of tree litter during the initial phase of succession has shown to result in the high concentrations of soil nutrients and thriving microbial communities ([9]). However, in old-growth forests, decomposition of litter generated by the forests themselves also increases soil nutrients. Unlike Nmass, Pmass tended to decrease as succession proceeded. Our results are in agreement with previous studies ([6]). These difference in Nmass and Pmass use demonstrates the differences in life history strategy among plants during succession, and reflects the important fundamental niche axes along which the species are differentiated ([37]). The high Pmass indicates high photosynthetic capacity of the plant leaves and a fast-growing, light-demanding species with a high potential for successful establishment in forest gaps ([14]).
The N:P ratio drives plant community structure and function, and is negatively related to growth rate ([10]). However, in the present study Nmass:Pmass increased with succession, which is in agreement with a previous study ([5]). Two theories could explain this phenomenon. First, species differ in their optimal N:P ratios for growth and reproduction, with species-specific homeostasis achieved by nutrient-balancing mechanisms ([21], [18]). Although the study sites all are in the Pu’er region, environmental filters only determine the possible species pool within a biogeographic region. Successional stage determines species composition and different species composition alone could lead to local-scale and biome-scale differences in N:P ratios. Over 50% of species in this study were different among four successional stages (Tab. S2 in Supplementary material). Species with different biological properties have separate nutrient use strategies, resulting in different N:P ratios among four successional stages. Second, regardless of species composition, different processes drive N and P availability in different communities. P is mainly derived from weathering and leaching, which is a slow biogeochemical process and its availability in the soil reflects the physical and chemical characteristics of the local parent material. In contrast, many processes drive N availability ([1]), and N mineralization and release is more abundant than elemental P in litter ([15]). Large differences exist in the availability and the uptake efficiency between elemental N and P. Changes in environmental conditions and community structure affect the availability and uptake of N and P element in successional stages of evergreen broad-leaved forest ([41]). This likely results in different N:P ratios in different species. Moreover, although plant N and P concentrations co-vary consistently, when N and P sources differ, environmental conditions are extreme and the variability of nutrients storage by plants, a decoupling of leaf N and P appears. Based on numerous studies the threshold of foliar N:P ratios are < 14 and > 16 for N and P limitation, respectively ([16], [25]). In this study, Nmass: Pmass ratios in the 40-yr-old and the old-growth forest were > 16, clearly indicating that P was the main limiting factor in succession. Wardle et al. ([34]) found that in the later stages of forest succession, productivity declines and N:P ratios in the humic matter and fresh litter increase, suggesting that P was the limiting factor in succession.
Significant differences in leaf area and leaf carbon content were not observed, but maximum photosynthesis (mass- and area-basis) was different between the four successional stages. The changes in maximum photosynthesis with forest succession point to the replacement of fast-growing species with slower growing species, which tend to conserve internal resources more efficiently as succession proceeds ([13]). In early successional stages, the fast-growing species have higher maximum photosynthesis in order to obtain higher relative growth rate and rapid biomass gain. Carbon gain is maximized by the constant renewal of the leaf area in early succession, as new leaves have high levels of photosynthetic capacity ([28]). Low photosynthesis values may reflect shade adaptation ([33]) and relatively low nutrient availability in the intermediate successional stages ([24]). Conversely, in late successional stages carbon gain is maximized by the production of long-lived leaves, which have low photosynthetic capacity ([28]) and relatively high nutrient content.
The relationships between leaf traits in succession stages indicate that successional stage influences leaf characteristics. For SMA, over half of the regression slopes showed significant differences with forest succession. Although the same negative or positive relationship occurred for some trait pairs in all four successional stages, the SMA slope showed a significant difference with the greatest slope occurring in the old-growth or 15-yr-old forests. Although Pmass and Amass co-vary consistently in the world-wide leaf economics spectrum ([40]), Pmass was negatively associated to Amass in the 15-yr-old, and was positively associated to Amass in the 30-yr-old, 40-yr-old forests and old-growth forest. Moreover, we found the relationship of 11 trait-pairs where the SMA slope showed a non-significant difference. For example, SLA was negatively associated to Nmass: Pmass in the 15-yr-old, 30-yr-old and 40-yr-old forests, but not in old-growth forest; LA were positively associated to Parea in the 15-yr-old, 30-yr-old and 40-yr-old forests, but not in old-growth forest. The results indicate that covariation in leaf traits is not universal and will be affected by forest succession. As succession proceeds, fast-growing species are progressively replaced by slow-growing species, which results in the differences in resource use and life history strategy and the changes in leaf trait relationships.
Conclusions
Although consistent changes of all leaf traits with succession were not observed, the mean trait values of eight out of ten leaf traits differed between the four successional stages. The SLA decreased as the vegetation recovered and Nmass:Pmass was positively associated with forest succession. Meanwhile, five leaf traits were highly correlated to successional stage. SLA, Pmass and Amass were strongly negatively correlated to successional stage and Nmass:Pmass and Narea were strongly positively correlated. Additionally, the SMA analysis showed that the relationship between leaf traits changes with succession. These results show that leaf traits are affected by forest succession and one or a combination of several leaf traits can determine successional stages in the monsoon evergreen broad-leaved forest.
Acknowledgements
LW carried out the field measurements and drafted the manuscript. SJ conceived the study and revised the manuscript. We are also very thankful to Damon Hartley and Zhen Yu for revising the English text. We would also like to thank Alison Beamish at the University of British Columbia for her assistance with English language and grammatical editing of the manuscript.
Financial support came from National Natural Science Foundation of China (31370592, 31200461, 31470617 and 31460195) and Forestry industry research special funds for public welfare projects (201404211).
References
Gscholar
Gscholar
CrossRef | Gscholar
Gscholar
Authors’ Info
Authors’ Affiliation
Jianrong Su
Research Institute of Resources Insect, Chinese Academy of Forestry, Kunming 650224 (China)
Jianrong Su
Puer Forest Ecosystem Research Station, State Forestry Bureau, Kunming 650224 (China)
Corresponding author
Paper Info
Citation
Liu W, Su J (2017). Successional leaf traits of monsoon evergreen broad-leaved forest, Southwest China. iForest 10: 391-396. - doi: 10.3832/ifor2045-009
Academic Editor
Chris Eastaugh
Paper history
Received: Mar 08, 2016
Accepted: Nov 18, 2016
First online: Mar 16, 2017
Publication Date: Apr 30, 2017
Publication Time: 3.93 months
Copyright Information
© SISEF - The Italian Society of Silviculture and Forest Ecology 2017
Open Access
This article is distributed under the terms of the Creative Commons Attribution-Non Commercial 4.0 International (https://creativecommons.org/licenses/by-nc/4.0/), which permits unrestricted use, distribution, and reproduction in any medium, provided you give appropriate credit to the original author(s) and the source, provide a link to the Creative Commons license, and indicate if changes were made.
Web Metrics
Breakdown by View Type
Article Usage
Total Article Views: 42714
(from publication date up to now)
Breakdown by View Type
HTML Page Views: 36390
Abstract Page Views: 2367
PDF Downloads: 3050
Citation/Reference Downloads: 20
XML Downloads: 887
Web Metrics
Days since publication: 2690
Overall contacts: 42714
Avg. contacts per week: 111.15
Article Citations
Article citations are based on data periodically collected from the Clarivate Web of Science web site
(last update: Feb 2023)
Total number of cites (since 2017): 4
Average cites per year: 0.57
Publication Metrics
by Dimensions ©
Articles citing this article
List of the papers citing this article based on CrossRef Cited-by.
Related Contents
iForest Similar Articles
Research Articles
Relationships between leaf physiognomy and sensitivity of photosynthetic processes to freezing for subtropical evergreen woody plants
vol. 12, pp. 551-557 (online: 17 December 2019)
Research Articles
Evergreen species response to Mediterranean climate stress factors
vol. 9, pp. 946-953 (online: 07 July 2016)
Research Articles
Gas exchange characteristics of the hybrid Azadirachta indica × Melia azedarach
vol. 8, pp. 431-437 (online: 17 December 2014)
Research Articles
Photosynthesis of three evergreen broad-leaved tree species, Castanopsis sieboldii, Quercus glauca, and Q. myrsinaefolia, under elevated ozone
vol. 11, pp. 360-366 (online: 04 May 2018)
Research Articles
Effect of environmental gradients on leaf morphological traits in the Fandoghlo forest region (NW Iran)
vol. 13, pp. 523-530 (online: 13 November 2020)
Research Articles
First vs. second rotation of a poplar short rotation coppice: leaf area development, light interception and radiation use efficiency
vol. 8, pp. 565-573 (online: 27 April 2015)
Technical Advances
A simplified methodology for the correction of Leaf Area Index (LAI) measurements obtained by ceptometer with reference to Pinus Portuguese forests
vol. 7, pp. 186-192 (online: 17 February 2014)
Research Articles
Estimation of forest leaf area index using satellite multispectral and synthetic aperture radar data in Iran
vol. 14, pp. 278-284 (online: 29 May 2021)
Research Articles
Evaluation and correction of optically derived leaf area index in different temperate forests
vol. 9, pp. 55-62 (online: 11 June 2015)
Research Articles
Gas exchange, biomass allocation and water-use efficiency in response to elevated CO2 and drought in andiroba (Carapa surinamensis, Meliaceae)
vol. 12, pp. 61-68 (online: 24 January 2019)
iForest Database Search
Search By Author
Search By Keyword
Google Scholar Search
Citing Articles
Search By Author
Search By Keywords
PubMed Search
Search By Author
Search By Keyword