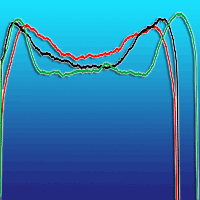
Physical and mechanical properties of particleboards manufactured using charcoal as additives
iForest - Biogeosciences and Forestry, Volume 10, Issue 1, Pages 70-74 (2016)
doi: https://doi.org/10.3832/ifor1963-009
Published: Jun 29, 2016 - Copyright © 2016 SISEF
Research Articles
Abstract
The objective of this work was to evaluate selected physical and mechanical properties of experimental particleboards manufactured from pine and spruce with charcoal particles in their core layer. For all the manufactured boards the average density was 750 kg m-3, while the mass share of charcoal in the core layer was changed (0%, 10% and 50%). The manufactured panels were tested with respect to their mechanical and physical properties, including formaldehyde emission. The results indicated that the share of charcoal significantly influenced mechanical properties, swelling, and water relations of the boards. In addition, a test on formaldehyde emission from panels were carried out, which revealed that the charcoal share has a considerable impact on the amount of formaldehyde released by the manufactured boards. The 50% content of charcoal caused about 80% reduction of formaldehyde emission.
Keywords
Charcoal, Particleboard, Filler, Bending, Formaldehyde, Emission
Introduction
Wood composites are expected to attain higher and higher quality standards as their market demand increases. Emphasis is placed not only on their mechanical properties, but also on the characteristics affecting human health, like the emission of the carcinogenic formaldehyde, which sometimes caused the withdraw of such wood products from the market. Regulations on formaldehyde emissions from wood products is constantly becoming more strict, preventing the use of UF-(urea-formaldehyde) bonded panels in interiors. Indeed, UF resin is widely used as adhesive in wood composites industry, because of its low cost and high reactivity. It is mainly used for production of plywoods, particleboards, and medium density fiberboards, and represents approximately 60% of wood adhesive market ([10]).
A common method used for lowering formaldehyde emissions is the reduction of the molar ratio between formaldehyde and urea ([15]), although this practice reduces the bond strength, water resistance and assembly properties of panels. Alternatively, the addition of melamine to UF resin allow to obtain the MUF resin, which captures the formaldehyde and decrease its emission ([14]). However, melamine addition significantly increases the production cost of panels, thus its use is often limited.
Recently, Zhang et al. ([19]) reported on the reduction of formaldehyde emission from wood products without decreasing the mechanical properties of the UF resin, through the use of nanocrystalline cellullose (NCC) modified by the addiction of APTES (3-Aminopropyl triethoxysilane) and MPS (3-Methacryloxy propyltrimethoxysilane). UF resin supplemented with 1.5% APTES NCC has proven to reduce formaldehyde emission by 53.2% and increase the bonding strength by 23.6%, while the addiction of MPS yielded slightly lower performances ([19]). Moreover, the effect of ammonia and sodium sulfite on formaldehyde emissions was also investigated by Wang et al. ([18]), who used low-molecular-weight phenol-formaldehyde oligomers (PFO) to improve water resistance of a whey protein-based adhesive and decrease formaldehyde emissions from plywoods assembled with PFO-modified adhesive, with non-significant effects on its binding properties.
According to Ding et al. ([3]), surface finishing of wood-based materials also affects their formaldehyde emission. For example, higher formaldehyde emissions have been reported for surface-sealed plywood as compared with plywood not subjected to sealing treatment ([3]). Contrastingly, edge sealing of nine-ply poplar plywood with UF resin decreased formaldehyde emissions by 74.4%. Further, by comparing different coating procedures such as low pressure laminate (LPL), polyvinyl chloride (PVC), coating paper, direct coating and veneer overlay/ UV lacquer, Park et al. ([16]) showed that the latter method has the lowest formaldehyde emission. Chen et al. ([2]) investigated the relation between assembly time of plywood and its properties, and showed that the plywood pre-pressed for 0 to 8 h before hot pressing had the lowest formaldehyde emission.
A further method aimed at decreasing formaldehyde emission from UF resin is the application of wood-derived bio-oil in the production of three-layered plywood ([12]), which also does not decrease its bonding strength. Other studies showed that formaldehyde emission can be reduced by over 42% by decreasing the melamine allocation proportion (in the alkaline hydroxymethylation step - M1 and/or alkaline post amino addition step - M2) in three-layer plywood bonded by MUF resin ([14]). According to these authors, cheap sepiolite can replace wheat flour as glue mass filler (80%) with MUF resin in plywood production, with an observed reduction of emissions by 7.8%. Kumar et al. ([11]) studied the effect of the addition of small amounts of activated carbon on formaldehyde emissions from composite materials, showing an improvement in terms of modulus of rupture and internal bonds, as well as a significant decrease of emissions. Akgül et al. ([1]) investigated the influence of burned wood as a component of panels bonded by melamine-urea-formaldehyde (MDF), and made of various mixtures of burned and unburned wood of pine, beech and oak. All the panels met the European standards in terms of mechanical properties of the MDF, though the surface roughness increased and the dimensional stability decreased with the addition of burned wood; formaldehyde emission was not investigated.
The above mentioned results suggest the existence of a correlation between the presence of thermally processed wood, including charcoal, in selected wood-based composites structure, and formaldehyde emission from these composites. The goal of this study was to evaluate selected physical and mechanical properties of experimental particleboards manufactured from pine and spruce with different content of charcoal particles in the core layer.
Material and methods
Panels
Three layer particleboards with dimensions 320 × 320 × 12 mm3 and average assumed density of 750 kg m-3 were produced from industrial particles for particleboards production. The moisture content of the particles was about 5%. The raw material for particles was 95% chips of softwood species such as Scots pine (Pinus sylvestris L.) and Norway spruce (Picea abies Karst.). The face-to-core layers ratio (by weight) was 32:68. Three different panel variants were produced: (0) the reference panel, with no charcoal particles in the structure; (10) with 10% of the core layer weight made by charcoal particles; and (50) with 50% of the core layer weight made by charcoal particles. For each variant, 4 panels were produced. The origin of the commercially available charcoal was beech (Fagus sylvatica L.) and hornbeam (Carpinus betulus L.) wood, with a moisture content of 5%. The commercial charcoal was ground to particles by a hammer mill, and then sieved with mesh to obtain particles of 1 to 4 mm. As bonding agent, urea-formaldehyde (UF) industrial resin, typically used for particleboard production, was used. The hardener was a water solution of ammonium chloride (NH4Cl), in proportion 8:100 (hardener solution to resin) by weight, to achieve the curing time of glue mass to about 86 s at 100 °C. The resination of face and core layers was 12%. No paraffin vax was used. A glue mass was pneumatically spread onto wood (and charcoal) particles mixed in a drum resinator. After manual forming, the mats were pressed in a hot press under the following conditions: temperature 180 °C; specific pressing pressure 2.5 MPa; pressing time factor 15 s/1 mm of the panel thickness. Panels were then conditioned in 20 °C and 65% of relative humidity (RH) to weight stabilization, reaching an equilibrium moisture content (EMC) of about 8% before testing.
Mechanical properties
At least 10 samples of each panel variant were used in testing their mechanical and physical properties. As for density profile measurements, 3 samples of each variant were used, and 2 samples of each variant for formaldehyde emission. Mechanical tests were carried out by using a computer-controlled INSTRON universal testing machine. The following parameters were assessed:
- Modulus of Rupture (MOR) and Modulus of Elasticity (MOE) in static bending, according to EN310 ([4]),
- Internal bond (IB), according to EN319 ([7]),
- Screw withdrawal resistance (SWR), according to EN320 ([8]).
Physical parameters
Thickness swelling (TS) after 2 and 24 h of soaking in water was measured according to EN317 ([6]) using 10 samples of each panel type. Water absorption (WA) was investigated while measuring TS, and calculated as follows (eqn. 1):
where WA
is the water absorption (%), m
1 is the sample weight before soaking (in g), and m
2 is the sample weight after soaking (in g).
Formaldehyde emission (FE) was measured according to EN717-2 ([9]) standard in 2 samples per each panel type. Density profile was measured using a GreCon Da-X® (x-ray) analyser (Fagus-GreCon Gmbh, Alfeld-Hannover, Germany), with 0.02 mm panel thickness sampling step on 3 samples for each panel type.
Statistical analysis
The analysis of variance (ANOVA) was carried out to test for differences among different panel types (panels 0, panels 10, panels 50) in mean values of all the measured variables, except formaldehyde emission and density profile. Linear regression (α = 0.05) was applied to investigate the relationship between formaldehyde emission and the charcoal particle content of the tested panels.
Results and discussion
Density profile
The density gradient between face and core layers increased with increasing the charcoal content in the core layer (Fig. 1), thus the transition between face and core layers was more marked. Their difference in density was about 146 kg m-3 for reference panels (0), 230 kg m-3 for panels 10 and 258 kg m-3 for panels 50. Mean density of the core layer slightly decreases with increasing charcoal particles share. A slightly lowered average density (712 vs. 750 kg m-3) was recorded for panels 50 as a consequence of their increased thickness (13.58 mm), likely due to the limited densification of charcoal particles. The plasticization process of charcoal under elevated temperature and pressure can be unequal and not as intensive and stable as for wood particles. However, no significant (lower) density regions in the core layer of panels with charcoal were detected on the plot, indicating the absence of undensified particles of charcoal. Indeed, this kind of material has been reported to show density values as low as 300 kg m-3 or even less ([17]).
Fig. 1 - Density profiles of reference panels (0), panels 10 (10% of charcoal particles in the core layer) and panels 50 (50 % of charcoal particles). Average density values (kg m-3) are in parenthesis.
Mechanical properties
The modulus of rupture (MOR) of tested panels significantly decreases with increasing the share of charcoal particles in the core layer (Fig. 2). The MOR for panels 10 (10% of charcoal particles applied in the core layer) decreased by about 19% as compared to panels 0 (reference panels), while for panels 50 (50% of charcoal particles) such reduction was about 46%. All the differences in average MOR values between panels types were statistically significant (p<0.05).
Fig. 2 - Modulus of rupture (MOR) and modulus of elasticity (MOE) of panels with different content of charcoal particles.
The decrease of MOR observed for panels 10 and panels 50 is likely caused by the brittleness of charcoal particles embedded in their core layer. In fact, the bending strength of flat materials, such as particleboards, is known to depend on the tension/compression strength of face layers, as well as on the shear strength of the core zone. It was observed that in the panels 50 (highest content of charcoal) the damage during bending occurs due to shear forces in the core layer, while in the panels 0 the damage occurred in the face layers. During the pressing of layers, charcoal particles may provoke cracks at different scale in the panels, due to their lower thermoplasticity compared to wood particles. This could still determine a high compression resistance of the panels, but a lowered resistance to shear.
Regarding the modulus of elasticity (MOE), significant differences were found between panels 0 and panels 50, as well as between panels 10 and panels 50. In this case, the lower bending strength of charcoal particles is counterbalanced by their rigidity (strongly connected to brittleness), as well as by the higher densification of face layers. In particular, no MOE reduction was detected for panels 10, probably because of the close-to-optimal content ratio of stiff charcoal particles densified closer to the surface layers, which results in a higher load and comparable low deflection. However, according to EN312 ([5]), only panels 0 (reference) and panels 10 meet the requirements of minimal MOR values for P2 type panels (applied to materials for interiors and furniture), while MOE minimal requirements were met by all the tested panels.
Internal bond strength
The differences in density profile described above might potentially affect the internal bond (IB) of the tested panels. However, we found no significant decrease of IB strength by increasing from 0 to 10% the charcoal particles content in the core layer (Fig. 3). In contrast, IB values of panels 50 (50% of charcoal particles) were significantly lower compared with the other two panel types.
Breaks in the surface layer (1-2 mm in depth) were observed in few samples during this test, especially in panels 0. This may indicate that the press closing speed was too slow, so the surface layers dried out before full pressure was applied. In fact, the surface density of reference panels is lower compared to the core layer. Contrastingly, in panels 50 (where the densification of face layers was higher) the cracks occurred in the core layer, which had the lowest density and a higher charcoal content. It is worth noting that the IB standard deviation decreased with increasing the charcoal content of the panels (Fig. 3), which is also due to the higher densification of face layers, thus moving the fracture zone closer to the middle of panel thickness. None of the tested panels met the requirements of minimal IB strength for panel type P2, according to EN312 ([5]) standard.
Screw withdrawal resistance
The screw withdrawal resistance (SRW) significantly decreased with increasing charcoal particles content in the core layer of particleboards. As compared with reference panels (0), SWR decreased by about 30% for panels 10 and over 54% for panels 50 (Fig. 4), with statistically significant differences between all the means of the three panel types. This suggests that the resistance of the rigid charcoal particles incorporated into the particleboard is not sufficient to face the screwing load, that tear and split the charcoal particles like a wedge. In this case, with the increased amount of charcoal particles in the core layer, SWR mostly depends on the strength of the thin surface layers, which represent only 32% by weight of the composite panels.
Thickness swelling
After soaking in water for 2 hours, thickness swelling (TS) was observed to increase with increasing the charcoal particle content in the core layer, with significant differences between panels 0 and panels 50 (Fig. 5). It is likely that panels containing charcoal particles could be penetrated by water more easily than reference panels due to their structure. However, the initial soaking time (2h) was long enough to fully soak the face layers.
After 24h of soaking, the only significant difference in TS was observed between panels 0 and panels 10. Longer soaking time did not cause significant thickness change of panels 50. After 24h of soaking, the highest thickness swelling (34%) was reached by panels with 10% of charcoal. The reason of the high swelling in thickness of panels 10 can be their higher density, compare to the other tested panels. Since the thickness of panels 50 was over 13.5 mm (thus having a lower density), these panels could not reach high thickness swelling. It is worth to mention that according to EN312 ([5]) standard, the maximum thickness swelling after 24h of soaking in water for panels type P3, non-load bearing, to be used in humid conditions, is 17%. None of the tested panels matched the above standard requirement.
Water absorption
Water absorption increased as the charcoal content of the core layer of panels increases (Fig. 6). After 2h of soaking, the difference in water absorption between panels 0 and panels 10 was about 21%, and raised to about 45% by comparing panels 0 and panels 50. After 24h of soaking, water absorption differed by about 22% between panel 0 and panels 10, by about 38% between panels 50 and reference panels. This result indicates that the intensity of water absorption decreases with the increase of soaking time and charcoal content of the panels. This can be explained by differences in the physical structure between wood and charcoal particles. Indeed, the latter showed a greater specific surface, thus they can absorb water more easily and maximal water uptake can be reached in a shorter period of soaking. Contrastingly, wood particles, which are compressed in the panels, need a longer time to reach the maximum water absorption level because of their lower specific area.
Formaldehyde emission
The results of formaldehyde emission from the tested panels are shown in Fig. 7. The emission of formaldehyde linearly decreased as charcoal content in the core layer of panels increases, from 2.57 mg m-2 h-1 for panels 0 to 0.52 mg·m-2 h-1 for panels 50, which means a reduction of about 80% in formaldehyde emission. Extrapolating the linear regression found, formaldehyde emission is expected to completely disappear when the charcoal content in the panels is about 62.6%. Such significant reduction in formaldehyde emission is due to strong ability of charcoal to trap gases in its structure.
Fig. 7 - Results of the linear regression between the charcoal content of the tested panels and their formaldehyde emission.
A similar effect of formaldehyde content reduction was observed by Kumar et al. ([11]) using MDF panels with addition of 0, 2, 5.2 and 10.4% wt. of activated charcoal. According to these results, the increasing content of charcoal caused a linear reduction of formaldehyde content from 9.82 mg 100-1 g of 6.5% MC panels without charcoal to about 7 mg 100-1 g in panels with 10.4% wt. of charcoal added, which means a reduction > 28%.
Conclusions
In this study, the increasing content of charcoal particles in the core layer of particleboards resulted in: (i) a higher densification of face layers; (ii) a significant reduction of the modulus of rupture and a non-significant increase of the modulus of elasticity when adding 10% of charcoal; (iii) a non-significant increase of the internal bond of panels with 10% of charcoal content, as compared with panels without charcoal, as well as a significant internal bond reduction for 50% charcoal content panels compared to reference panels; (iv) a significant, almost linear reduction of screw withdrawal resistance; (v) an increase of thickness swelling and water absorption; (vi) a significant, linear reduction of formaldehyde emission. Since charcoal particleboard panels did not meet most of the standard requirements for furniture, their use for interior equipment is recommended for their remarkably lowered formaldehyde emission.
List of abbreviations
The following abbreviations were used throughout the manuscript:
- APTES: aminpropyltriethoxysilane;
- BUF: bio-oil urea-formaldehyde;
- EMC: equilibrium moisture content;
- FE: formaldehyde emission;
- IB: internal bond;
- LPL: low pressure laminate;
- MDF: medium density fiberboard;
- MOE: modulus of elasticity;
- MOR: modulus of rupture;
- MPS: methacryloxypropyltrimethoxysilane;
- MUF: melamine-urea-formaldehyde;
- NCC: nano crystalline cellulose;
- PFO: phenol-formaldehyde oligomers;
- PVC: polyvinyl chloride;
- RH: relative humidity;
- SWR: screw withdrawal resistance;
- TS: thickness swelling;
- UF: urea-formaldehyde;
- UV: ultraviolet;
- WA: water absorption.
Acknowledgements
Part of this work has been done under statutory research of Department of Technology and Entrepreneurship in Wood Industry, Faculty of Wood Technology, Warsaw University of Life Sciences - SGGW, Poland.
References
Authors’ Info
Authors’ Affiliation
Mateusz Zajac
Ewelina Czubak
Radoslaw Auriga
Faculty of Wood Technology, Warsaw University of Life Sciences, Nowoursynowska Str. 159, 02-776 Warsaw (Poland)
Corresponding author
Paper Info
Citation
Kowaluk G, Zajac M, Czubak E, Auriga R (2016). Physical and mechanical properties of particleboards manufactured using charcoal as additives. iForest 10: 70-74. - doi: 10.3832/ifor1963-009
Academic Editor
Giacomo Goli
Paper history
Received: Dec 22, 2015
Accepted: Feb 29, 2016
First online: Jun 29, 2016
Publication Date: Feb 28, 2017
Publication Time: 4.03 months
Copyright Information
© SISEF - The Italian Society of Silviculture and Forest Ecology 2016
Open Access
This article is distributed under the terms of the Creative Commons Attribution-Non Commercial 4.0 International (https://creativecommons.org/licenses/by-nc/4.0/), which permits unrestricted use, distribution, and reproduction in any medium, provided you give appropriate credit to the original author(s) and the source, provide a link to the Creative Commons license, and indicate if changes were made.
Web Metrics
Breakdown by View Type
Article Usage
Total Article Views: 41415
(from publication date up to now)
Breakdown by View Type
HTML Page Views: 34918
Abstract Page Views: 1459
PDF Downloads: 4047
Citation/Reference Downloads: 42
XML Downloads: 949
Web Metrics
Days since publication: 2950
Overall contacts: 41415
Avg. contacts per week: 98.27
Article Citations
Article citations are based on data periodically collected from the Clarivate Web of Science web site
(last update: Feb 2023)
Total number of cites (since 2017): 3
Average cites per year: 0.43
Publication Metrics
by Dimensions ©
Articles citing this article
List of the papers citing this article based on CrossRef Cited-by.
Related Contents
iForest Similar Articles
Short Communications
Influence of thermo-vacuum treatment on bending properties of poplar rotary-cut veneer
vol. 10, pp. 161-163 (online: 13 June 2016)
Research Articles
Characterization of VOC emission profile of different wood species during moisture cycles
vol. 10, pp. 576-584 (online: 08 May 2017)
Research Articles
The physicomechanical and thermal properties of Algerian Aleppo pine (Pinus halepensis) wood as a component of sandwich panels
vol. 15, pp. 106-111 (online: 21 March 2022)
Research Articles
Physical, chemical and mechanical properties of Pinus sylvestris wood at five sites in Portugal
vol. 10, pp. 669-679 (online: 11 July 2017)
Research Articles
Physical and mechanical characteristics of poor-quality wood after heat treatment
vol. 8, pp. 884-891 (online: 22 May 2015)
Research Articles
Heat treatment of poplar plywood: modifications in physical, mechanical and durability properties
vol. 16, pp. 1-9 (online: 09 January 2023)
Research Articles
Identification of wood from the Amazon by characteristics of Haralick and Neural Network: image segmentation and polishing of the surface
vol. 15, pp. 234-239 (online: 14 July 2022)
Research Articles
Comparison of alternative harvesting systems for selective thinning in a Mediterranean pine afforestation (Pinus halepensis Mill.) for bioenergy use
vol. 14, pp. 465-472 (online: 16 October 2021)
Research Articles
Physical-mechanical properties and bonding quality of heat treated poplar (I-214 clone) and ceiba plywood
vol. 8, pp. 687-692 (online: 17 December 2014)
Research Articles
NIR-based models for estimating selected physical and chemical wood properties from fast-growing plantations
vol. 15, pp. 372-380 (online: 05 October 2022)
iForest Database Search
Search By Author
Search By Keyword
Google Scholar Search
Citing Articles
Search By Author
Search By Keywords
PubMed Search
Search By Author
Search By Keyword