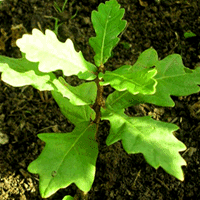
Oak sprouts grow better than seedlings under drought stress
iForest - Biogeosciences and Forestry, Volume 9, Issue 4, Pages 529-535 (2016)
doi: https://doi.org/10.3832/ifor1823-009
Published: Mar 17, 2016 - Copyright © 2016 SISEF
Research Articles
Collection/Special Issue: IUFRO division 8.02 - Mendel University Brno (Czech Republic) 2015
Coppice forests: past, present and future
Guest Editors: Tomas Vrska, Renzo Motta, Alex Mosseler
Abstract
This study focused on the comparison of two contrasting forest regeneration types and their susceptibility to drought stress. Transpiration and biomass production were studied on young sessile oak trees Quercus petraea (Matt.) Liebl. regenerated as sprouts and seedlings, and grown in a coppice experimental site in the Czech Republic. Biomass production was estimated using destructive methods, while transpiration was derived from sap flow measurements and assessed according to the plant biometry and microclimatic conditions. Sprouts were characterized by a significantly higher diameter, height, leaf area and above-ground biomass and by a lower wood density as compared with seedlings of the same age. Moreover, the sap flow of sprouts was higher than that of seedlings, which was explained by the plant dimension. Transpiration, expressed as sap flow scaled to plant leaf area, did not differ between seedlings and sprouts when soil water was not limiting. However, during drought periods, when soil water potential dropped below -1.4 MPa, sprouts transpired significantly more than seedlings. Our results confirm that sprouts have access to a larger water pool via the old stump root system and are able to draw more water under drought. Moreover, sprouts seemed to be less susceptible to water limitations than seedlings of similar age. Less influence of drought on sprouts may partially explain their higher above-ground biomass production. Based on our results, coppice could be an appropriate management system to be adopted in sites characterized by frequent or extreme drought periods.
Keywords
Drought Stress, Sap Flow, Transpiration, Biomass Production, Sessile Oak, Coppice, Sprout, Seedling
Introduction
Coppicing is the oldest silvicultural method used worldwide, and has played an important role in Europe until the second half of the 19th century ([25], [16]). After many years of marginal importance due to the abandonment and conversion of coppices into high forests, a new interest in the coppice system arose for biomass production, nature conservation, biodiversity maintenance ([41], [24]) and as an alternative to urban forestry ([31]). Moreover, the coppice system has proved to be advantageous for slope stabilization, as fast-growing sprouts control soil erosion by intercepting precipitation and maintaining a vigorous, well-developed rooting network ([34]).
Water availability is one of the most limiting factors for plant growth ([21]). Drought affects water relations and water use efficiency ([5]), alters the nutrient uptake and reduces photosynthesis ([15]). Additionally, drought may lead to hydraulic failure and carbon starvation, due to decreased photosynthesis and transport of non-structural carbohydrates, and increase the susceptibility to other abiotic and biotic stress factors ([35], [26]). Global climate change models predicted the occurrence of more frequent and severe drought periods in the future ([19]). The projected increase in temperature will cause greater evapotranspiration and surface drying. Along with unbalanced precipitation (long periods of dryness, extreme storms and increased run-off - [39]), this may cause a severe water deficit during the growing season, especially in shallow soil layers, which are the most important for seedling establishment. Furthermore, drought reduces biomass production, decreases forest stability ([3]), and increases tree mortality rates ([1]).
The regeneration type of stand recruitment (generative for seedlings or vegetative for sprouts) deeply affects the early development of new trees. Indeed, sprouts and seedlings show a different ability to use soil resources (nutrients, water) and compete with the surrounding vegetation. Several studies reported a faster initial growth of sprouts compared to seedlings, as they benefit from the existing root system of the stump ([12], [7] [23], [42]). Moreover, the rapid growth of sprouts can hamper the natural generative regeneration of stands, as sprouts can out-compete seedlings in the early stages ([42]). The initial reduced ability of seedlings to uptake resources from the soil makes them more susceptible to water and nutrient stress ([36]). However, many physiological aspects of growth, biomass production and susceptibility to stress factors related to different regeneration forms have not been studied in detail.
We hypothesized that sprouts cope better with drought as they better tolerate water limitations compared to seedlings of similar age, resulting in a higher biomass production. The aim of the present study was to compare young sessile oak (Quercus petraea (Matt.) Liebl.) seedlings and sprouts in terms of biomass production, sap flow, transpiration, water use and susceptibility to water deficit in relation to plant biometry and microclimatic conditions.
Material and methods
Site description
The study was conducted at the experimental site of Mendel University in Brno, located near Sobešice, in the southeast of the Czech Republic (49° 14′ N, 16° 35′ E, 300 m a.s.l.). The annual mean temperature of the site is approximately 7.5 °C and the average annual precipitation varies from 550 to 650 mm ([40]). The main forest type of the experimental site (according to the Czech forest typology system) is rich oak-hornbeam with meadow-grass and soft-leaved sedge on a plateau and rounded ridges with some dry oak-hornbeam in exposed stands at lower altitudes ([20]). According to Plíva ([32]), such forest types are significantly influenced by drought. The research site was established in the winter of 2009/2010 by cutting of a 73-year-old forest formed by 95% of sessile oak (Quercus petraea (Matt.) Liebl.) of predominantly coppice origin. The whole area (4 ha) was divided into 16 square-shaped plots of 2500 m2 differing in felling intensity ([20]). The study presented in this paper was conducted in one of the post-clearcut plots, with sessile oak regenerated naturally as seedlings and sprouts.
Biometric characteristics
A total of twenty-two plants (eleven sprouts and eleven seedlings) were harvested to compare their biometric characteristics. Before harvesting, plant height (H, cm) and stem diameter 10 cm above the stump/ground (i.e., base diameter D0.1m, cm) were recorded. The cross-sectional area (CSA, cm2) was calculated on the basis of D0.1m. Leaf samples (ten per plant) were taken randomly from the upper part of the crown. Leaves were scanned fresh, then dried at 105 °C to steady weight, and finally weighed. The specific leaf area (SLA, cm2 g-1) was calculated. Wood samples (ca. 5 cm long) from 10 cm above the ground/base were measured, dried at 105 °C to reach a constant dry weight and then weighed. Plant wood density (ρ, g cm-3) was calculated based on the wood sample volume and its dry weight. The age of plants was determined by counting the annual rings on cross-sections of the wood samples, taken at the same height. All plants were four years old. All the above-ground plant material was sub-divided into leaves, branches and stem, dried as described above and its dry biomass measured (g plant-1). Specific leaf area and leaf biomass were used to calculate the entire plant leaf area (LA, m2 plant-1).
Sap flow and transpiration
Sap flow was measured based on the trunk heat balance method (THB - [22], [10]) and used to estimate the water consumption of seedlings and sprouts. The measurements were conducted from April to September 2014 on five seedlings and five sprouts in the same plot. EMS 62A sensors (EMS, Czech Republic) with external heating and internal temperature sensing were mounted on the plant stem below the foliage at a mean height of 25 cm above the ground/stump. The stem diameter at the position of sensors varied from 8 to 18 mm. The measurement system was set to maintain a 4 °C temperature difference between two thermocouples. The input power needed to maintain a fixed temperature difference was proportional to the amount of water passing the sensor (the sap flow Q, expressed in kg h-1). Measurements were conducted every minute, while data averages were recorded at ten-minute intervals. Heat loss from sensors was eliminated from the total sap flow value on the basis of zero-flow baseline subtraction at 0300 h, conducted using the Mini32 software pack (Mini32, EMS, Czech Republic). Sap flow measurements were used to calculate mean hourly and daily whole plant water fluxes. In order to compare plants of different sizes, sap flow data were scaled using the parameters D0.1m, CSA, H and LA. Plant leaf area was chosen as the best scaling parameter based on the coefficient of determination obtained from regression analysis. Sap flow scaled to plant LA was expressed as seedling and sprout transpiration (E, kg m-2 h-1) and used in further analyses.
Microclimatic measurements
The following microclimatic variables were recorded at the experimental site: global radiation (GR, W m-2); air temperature (Tair, °C); relative air humidity (RH, % - all the three variables measured by Minikin RTHi, EMS, Brno, CZ); precipitation (P, mm - MetOne 380, EMS, Brno, CZ); volumetric water content of soil (VWC, m3 m-3 - 10 HS, Decagon Devices, Inc., Pullman, USA); and soil water potential (SWP, MPa - GB 2, EMS, Brno, CZ). Soil water potential and soil volumetric water content were measured using six sensors at the following three depths: 5, 30 and 55 cm. All microclimatic variables were measured in one-hour intervals during the entire period of study. Microclimatic data were used to calculate the vapor pressure deficit (VPD, Pa) and the potential evapotranspiration (ET0, mm) using the FAO modified Penman-Monteith equation ([2]), in order to detect periods of water stress.
Drought stress indication
The analysis of precipitation patterns, volumetric soil water content and soil water potential allowed to identify drought periods, defined as those days without precipitation, with a relatively high VPD and temperature (according to site-specific microclimate monitored for several years) and SWP below -1.2 MPa at the three measured soil depths. Drought stress was considered severe when SWP < -1.5 MPa and moderate when -1.2 < SWP ≤ -1.5 MPa after Hsiao ([18]). Climatic variables were used to distinguish periods with limiting and/or normal water conditions. Days under normal and dry soil conditions, but with comparable evaporative demand (GR, VPD, ET0) were selected for the comparison of sprout and seedling transpiration.
According to Bequet et al. ([6]), other indicators of water stress were recorded such as the continuously decreasing sap flow rate with no change in ET0, the decrease in the effective crown area (Aeff, m2) and the small relative effective crown area (aeff, %). Plant sap flow and ET0 were quantitatively compared during days without precipitation, as suggested by Nicolás et al. ([30]). The effective crown area of plants was calculated according to Bequet et al. ([6]) as the ratio Q/ET0, and the relative effective crown area as the ratio of the current-to-maximal effective crown area over the studied growing season (measured on 9th and 10th August). These parameters provide information on the extent to which the plant is able to follow the evaporative demands of the surrounding air. Low Aeff and aeff indicate soil water stress conditions.
Statistical analysis
Statistical analyses were conducted using STATISTICA® version 12 (StatSoft Inc., Tulsa, OK, USA) and SigmaPlot® (Systat Software Inc., Chicago, IL, USA). Biometric data were analyzed by t-test, ANOVA and regression analyses. The Q and E of seedlings and sprouts were compared using the non-parametric Mann-Whitney U test. Simple and multiple regressions were used to analyze the relationships between transpiration, plant biometry, regeneration type and climatic conditions.
Results
Biometric studies
Most of the measured biometric parameters differed significantly between seedlings and sprouts (Fig. 1). Compared to sprouts, seedlings had a lower H and D0.1m by approx. 35% and 53%, respectively. The differences in biomass of the various plant parts varied from 81% (leaves) to 87% (branches). It is worth noticing that the difference in the plant LA was approx. 77% greater for sprouts. Constrastingly, wood density was significantly higher in seedlings (approx. by 40%), which was related to their slower stem diameter increment as compared with sprouts.
Fig. 1 - Comparison of seedling and sprout biometric characteristics. (D0.1m): plant stem diameter 10 cm above the ground/stump base (cm); (CSA): base cross-sectional area (cm2); (H): height (cm); (LB): leaf biomass (g plant-1); (BB): branch biomass (g plant-1); (SB): stem biomass (g plant-1); (TAB): total above-ground biomass (g plant-1); (ρ): wood density (g cm-3); (SLA): specific leaf area (cm2 g-1); (LA): leaf area (m2 plant-1); (*): p < 0.05; (**): p < 0.01; (***): p < 0.001.
Sprouts and seedlings differed in the relations between the individual biometric parameters. The relation between D0.1m [mm] and H [cm] was strong for sprouts (R2 = 0.86, D0.1m = 0.014×H - 0.538, p < 0.001) but not significant for seedlings. Plant leaf area was significantly related to D0.1m in both plant types, with R2 being 0.75 and 0.84 for sprouts and seedlings, respectively. The linear regression function between plant leaf area [m2] and stem diameter [mm] was LA = 0.84×D0.1m - 0.39 for sprouts and LA = 0.75×D0.1m - 0.30 for seedlings, respectively. D0.1m was a suitable predictor of the total above-ground biomass [g], with the coefficient of determination R2 = 0.86 for sprouts (TAB = 0.859× D0.1m - 0.538) and R2 = 0.93 for seedlings (TAB = 0.927×D0.1m - 255.99). The correlation between plant leaf area and plant height was not significant. The specific leaf area did not differ between seedlings and sprouts.
Sap flow and transpiration
During the entire measurement period, sprouts had a significantly higher sap flow (Q) compared to that of seedlings, with a mean daily Q (±SE) of 0.20 ± 0.02 kg day-1 for seedlings, and 0.50 ± 0.04 kg day-1 for sprouts. The higher Q of sprouts was monitored during entire study period, despite the soil water potential. The analysis of the relation between biometric data and Q showed a high correlation of the latter parameter with the plant size. Regardless of plant type (vegetative or generative origin), the mean daily sap flow was best explained by the plant leaf area, then by CSA and D0.1m. A low though significant relation was also found between Q and H (Tab. 1).
Tab. 1 - Linear regressions of mean daily sap flow (Q) as a function of plant height (H), base diameter (D0.1m), base cross-sectional area (CSA) and plant leaf area (LA).
Parameter | Intercept | b-value | R2 | adjusted R2 | p- value |
---|---|---|---|---|---|
H | -0.547 | 0.007 | 0.67 | 0.39 | 0.0324 |
D0.1m | -0.586 | 0.066 | 0.86 | 0.84 | 0.0001 |
CSA | -0.133 | 0.296 | 0.92 | 0.85 | 0.0002 |
LA | -0.019 | 0.262 | 0.94 | 0.93 | 0.0000 |
As the mean daily sap flow was best explained by the plant LA, the observed Q values were scaled to the LA and expressed as plant transpiration (E). Thus, the water consumption of different plant types was properly compared without the influence of the plant size. No significant differences in the seasonal E were found between seedlings and sprouts. However, the relation between the transpiration of different plant types changed with SWP. The variable E was analyzed in several SWP classes of 0.1 MPa. Differences in transpiration occurred when the soil water potential dropped below -1.4 MPa, which is close to the severe water stress indicated by Hsiao ([18]). Days with SWP < -1.4 MPa made up almost 40% of the studied period; therefore the difference in transpiration between sprouts and seedlings was meaningful in this growing season.
Two four-day periods with similar evaporative demand (i.e., ET0 values non-significantly different) but with contrasting soil water conditions, were chosen for a detailed comparison of seedling and sprout water relations (Fig. 2). The drought period selected for monitoring was 21st-24th June while the non-limiting water conditions period was 6th-9th August. Two-factorial ANOVA tests showed significant differences in daily transpiration between these two periods in seedlings and sprouts (p = 0.00168). In the selected dry period, mean daily transpiration (± SE) was 0.08 ± 0.01 kg m-2 day-1 for seedlings and 0.24 ± 0.02 kg m-2 day-1 for sprouts. Under non-limiting water conditions, these values were 0.61 ± 0.05 kg m-2 day-1 and 0.56 ± 0.03 kg m-2 day-1 for seedlings and sprouts, respectively. Seedlings transpired under normal conditions eight times as much during drought, while sprouts approx. twice as much, which means a much greater decrease in transpiration of seedlings. Evidently, seedlings and sprouts differed in transpiration only during drought (p < 0.0001).
Fig. 2 - Seedling and sprout transpiration in relation to microclimatic variables in normal, non-limiting soil water conditions and in drought. (E): plant transpiration of seedlings (broken line) and sprouts (solid line); (VPD): vapor pressure deficit; (GR): global radiation.
The diurnal curves of transpiration also changed during drought periods (Fig. 2). In non-limiting water conditions, transpiration showed a dome-shaped diurnal course, following changes in global radiation and the vapor pressure deficit. This pattern changed for seedlings during drought conditions, when sap flow decreased before midday despite of transpiration demands, and continued to fall in the afternoon. In non-limiting water conditions, seedling and sprout transpiration strongly correlated (R2 = 0.98 - Fig. 3). This relation changed during drought stress, when R2 decreased and the slope of the regression function increased.
Fig. 3 - The relation between mean transpiration of seedlings and sprouts in drought (open circles) and non-limiting soil water conditions (full circles). Slopes (a) of the linear function equations and coefficients of determination R2 are shown in the graph.
Water stress indicators
The effective crown area (Aeff) was on average (± SE) 0.13 ± 0.008 m2 for sprouts and 0.05 ± 0.004 m2 for seedlings. The maximum (reference) Aeff was 0.27 m2 for sprouts and 0.13 m2 for seedlings. The mean relative effective crown area (aeff) was 39.7% for seedlings and 47.2% for sprouts. The Aeff and aeff were much lower during the drought stress period, especially for seedlings. Aeff was approx. one-third of these values lower in drought for sprouts, and almost one-tenth of these values for seedlings than during non-limiting water conditions (Tab. 2). The differences were not great during the non-limiting water conditions period.
Tab. 2 - Mean effective crown area (Aeff, m2) and relative effective crown area (aeff, %) of seedlings and sprouts during non-limiting water conditions (Normal) and during drought. (DOY): day of the year.
Plant type | Normal | Drought | ||||
---|---|---|---|---|---|---|
DOY | Aeff | aeff | DOY | Aeff | aeff | |
seedling | 218 | 0.112 | 84.9 | 141 | 0.017 | 12.8 |
219 | 0.114 | 86.4 | 142 | 0.016 | 12.0 | |
220 | 0.112 | 84.5 | 143 | 0.008 | 6.4 | |
221 | 0.095 | 71.6 | 144 | 0.008 | 6.4 | |
sprout | 218 | 0.265 | 97.4 | 141 | 0.087 | 31.9 |
219 | 0.263 | 96.6 | 142 | 0.109 | 40.0 | |
220 | 0.237 | 87.0 | 143 | 0.075 | 27.5 | |
221 | 0.213 | 78.1 | 144 | 0.072 | 26.5 |
Discussion
In our study, sprouts had a higher biomass and size compared to seedlings of the same age. Sprouts were characterized by a higher H, D0.1m, TAB and LA. Similar results have been previously reported by Dickmann et al. ([12]), Lloret et al. ([23]) and Vartiamäki ([42]). Initial growth of sprouts is associated with some degree of mobilization of previously accumulated reserves of nutrients, starch, sugars and other soluble and insoluble energy reserves ([43]). Sprouts benefit from the well-developed root system of a previously established tree ([7]), thus more biomass can be allocated to above-ground structures. Moreover, compared to seedlings in the early development stage, water-use efficiency of sprouts can be greater due to the surplus of water and nutrients, thus their photosynthetic activity is not limited by resource availability ([14]). The slower above-ground growth rate of seedlings can be attributed to their higher investment of assimilates into the root system, in order to improve water and nutrient uptake.
The observed differences in growth rate and above-ground biomass production between the two studied plant types can also be related to the influence of the drought. Stomata are environmentally controlled gateways of plants, controlling CO2 uptake and transpiration, thus regulating photosynthesis, cooling and nutrient uptake ([15]). Since transpiration and CO2 uptake occur in the leaf stomata, these two processes are strongly related ([11]). We found similar leaf-scale transpiration for seedlings and sprouts, suggesting similar photosynthetic rates during non-limiting water conditions. Contrastingly, seedlings had much lower transpiration during the drought period, as a consequence of the closure of stomata and soil water deficit. This response affects the net CO2 assimilation rate ([27]) and leads to lower plant biomass production ([9], [28]). Additionally, drought often limits nutrient uptake ([5]), as water is required for their solubilization and translocation ([37]). Also, drought may lead to carbon starvation by interfering with the transportation of carbohydrates from the storage in the roots to above-ground tissue ([35]). Therefore, frequent and severe droughts dramatically affect biomass production. At drier sites, seedling growth may even be slower than that of sprouts due to a lower water uptake, as observed in this study and previously reported by Drake et al. ([14]). As a result, in drought conditions sprouts have higher survival ability and faster growth than seedlings in the earliest ontogenetic stage ([23]).
Our results indicate lower water availability for seedlings during drought as a consequence of shallow roots and/or a smaller root absorptive area. Lloret et al. ([23]) studied the response of Phillyrea latifolia seedlings and Quercus ilex sprouts to experimental drought. Generally, Quercus ilex is more susceptible to drought than P. latifolia in the adult stage of development. However, these authors reported a greater water use efficiency (WUE) and ability to regulate water use under stressful conditions for Q. ilex sprouts as compared with P. latifolia seedlings. This suggests that sprouts are less stressed by water limitation since they benefit from resources stored in parent trees. Therefore, the type of regeneration alters the competition among species in mixed forests, as regeneration by sprouting gives to susceptible species a better chance of survival to drought.
Drake et al. ([14]) compared the transpiration and water use efficiency of young seedlings and sprouts of Eucalyptus globulus, finding a higher production of stem volume for sprouts compared to that of seedlings of 1-2 years. Transpiration was also higher in the case of sprouts both at individual and stand level. In their study, the difference in stand transpiration was mostly related to a significantly higher leaf area index (a greater surface area for transpiration) of the coppice. However, they did not find that the differences in transpiration were caused by a different level of stomatal control. In our case, differences in leaf area-scaled transpiration between seedlings and sprouts were observed under drought conditions, suggesting that plant origin can influence stomatal control when soil water is limited (i.e., SWP < -1.4 MPa).
It has been reported that differences in transpiration rate between seedlings and sprouts are primarily related to the transmissive surface area (sap wood area or leaf area) for water transport ([14]). This implies that plants with similar basal and leaf area would transpire at similar rates regardless they are seedlings or sprouts. This is in contrast with our results, as the influence of drought on seedling and sprout transpiration was significant in this study.
Simultaneous measurements of sap flow and microclimatic factors allowed us to obtain valuable information on the water relations of trees and their response to changes in evaporative conditions and in physiological processes related to the water balance ([29]), especially under limited soil water availability ([6]). Such approach also enabled the analysis of several drought indicators. Our results showed a significant decrease in the effective crown area and in the relative effective crown area during drought for both seedlings and sprouts, which shows the limited ability of plants to meet the evaporative demands during drought stress. A low effective crown area suggests stressful conditions, water deficit and stomatal closure. In our study, the significant difference in Aeff confirmed a more severe stress for seedlings, supporting the results of the transpiration analysis.
From the point of view of forest management, it is important to know how long the coppice system has an advantage over high forests in terms of production and water use, especially during droughts. Such advantage is connected to the differences in plant’s biomass allocation and growth dynamics. Transpiration of coppice trees will likely be higher as long as its leaf area is higher than that of high forest trees. As plant leaf area can be explained by allometric relations to biomass and basal diameter of plants (as showed in this paper), the difference in transpiration would probably exist until seedlings (or young trees) reach a similar size to coppice trees. However, the absorptive root area and the root-to-shoot ratio are the most important factors determining the water availability and transpiration of plants differing in their regeneration form ([36]). Therefore, seedlings may experience more severe water limitations than stump sprouts until they develop an extensive root system to withdraw water in drought periods. On the other hand, to our best knowledge, no studies comparing the root system of coppice and high forest trees and its development over time are available in the literature. However, in a study focused on the production at the stand scale, Giurgiu & Draghiciu ([17]) found that the total yield of a sessile oak coppice forest was equal to that of a high forest at the age of 40 years. Differences in water availability between coppice and high forest trees might theoretically exists at that age (or even later), though site-specific conditions should be taken into account.
Sessile oak is described as intermediately tolerant to drought ([4], [13]). Several studies demonstrated that net assimilation of CO2, stomatal conductance and transpiration of sessile oak decrease to overcome drought, and remain substantially constant during severe stress ([8], [33]). In this study, the observed small (though still measurable) transpiration of seedlings and sprouts suggests an incomplete stomata closure under stress conditions. According to Thomas & Gausling ([38]), stomatal conductance strongly contributes to drought tolerance in oaks. Sessile oak is able to overcome a long-term water shortage ([8]), although this may impair its biomass production and competition ability.
The increased initial biomass production and transpiration rate of sprouts under drought stress detected in this study suggest that coppice could be an appropriate management system in sites characterized by frequent droughts. In the context of the current climate change, a better understanding of drought response, survival, and mortality of trees ([26]), as well as the choice of the most appropriate management system, are critical to ensure long-term persistence of forests under the projected extreme drought.
Conclusion
Coppice and high forest differ in their regeneration form, and this affects the initial growth of trees, their utilization of resources and their susceptibility to stress factors such as drought. Significantly higher transpiration of seedlings during drought, together with the higher biomass production of sprouts under water shortage, confirmed the better performance of the coppice system as compared with high forest, at least during the initial stage of the forest development cycle.
Acknowledgments
This work was supported by the project “Coppice” no. CZ.1.07/2.3.00/20.0267. The authors thank the Internal Grant Agency of Mendel University in Brno for supporting this study with grant no. 36/2015. We would also like to thank Tereza Šlancarová and Pavlína Konrádová for their help with biomass analysis, Eva Darenová for her help with data processing, Jan Hobl for linguistic corrections and Matjaž Cater for his valuable comments and remarks.
References
CrossRef | Gscholar
Gscholar
Gscholar
Gscholar
Gscholar
Gscholar
Online | Gscholar
Authors’ Info
Authors’ Affiliation
Marko Stojanović
Radek Pokorný
Department of Biomass Production and Water Balance, Global Change Research Center, Academy of Sciences of the Czech Republic, v.v.i., Belidla 4a, 603 00 Brno (Czech Republic)
Marko Stojanović
Robert Knott
Radek Pokorný
Department of Silviculture, Faculty of Forestry and Wood Technology, Mendel University in Brno, Zemedelská 3, 613 00 Brno (Czech Republic)
Corresponding author
Paper Info
Citation
Pietras J, Stojanović M, Knott R, Pokorný R (2016). Oak sprouts grow better than seedlings under drought stress. iForest 9: 529-535. - doi: 10.3832/ifor1823-009
Academic Editor
Tamir Klein
Paper history
Received: Aug 26, 2015
Accepted: Jan 02, 2016
First online: Mar 17, 2016
Publication Date: Aug 09, 2016
Publication Time: 2.50 months
Copyright Information
© SISEF - The Italian Society of Silviculture and Forest Ecology 2016
Open Access
This article is distributed under the terms of the Creative Commons Attribution-Non Commercial 4.0 International (https://creativecommons.org/licenses/by-nc/4.0/), which permits unrestricted use, distribution, and reproduction in any medium, provided you give appropriate credit to the original author(s) and the source, provide a link to the Creative Commons license, and indicate if changes were made.
Web Metrics
Breakdown by View Type
Article Usage
Total Article Views: 53269
(from publication date up to now)
Breakdown by View Type
HTML Page Views: 44173
Abstract Page Views: 3492
PDF Downloads: 4176
Citation/Reference Downloads: 46
XML Downloads: 1382
Web Metrics
Days since publication: 3407
Overall contacts: 53269
Avg. contacts per week: 109.45
Article Citations
Article citations are based on data periodically collected from the Clarivate Web of Science web site
(last update: Mar 2025)
Total number of cites (since 2016): 22
Average cites per year: 2.20
Publication Metrics
by Dimensions ©
Articles citing this article
List of the papers citing this article based on CrossRef Cited-by.
Related Contents
iForest Similar Articles
Research Articles
Links between phenology and ecophysiology in a European beech forest
vol. 8, pp. 438-447 (online: 15 December 2014)
Research Articles
Leaf transpiration of drought tolerant plant can be captured by hyperspectral reflectance using PLSR analysis
vol. 9, pp. 30-37 (online: 05 October 2015)
Research Articles
Sap flow, leaf-level gas exchange and spectral responses to drought in Pinus sylvestris, Pinus pinea and Pinus halepensis
vol. 10, pp. 204-214 (online: 01 November 2016)
Research Articles
Comparison of assimilation parameters of coppiced and non-coppiced sessile oaks
vol. 9, pp. 553-559 (online: 25 March 2016)
Research Articles
Matching seedling size to planting conditions: interactive response with soil moisture
vol. 12, pp. 220-225 (online: 25 April 2019)
Research Articles
Effect of drought stress on some growth, morphological, physiological, and biochemical parameters of two different populations of Quercus brantii
vol. 11, pp. 212-220 (online: 01 March 2018)
Research Articles
Developing stand transpiration model relating canopy conductance to stand sapwood area in a Korean pine plantation
vol. 14, pp. 186-194 (online: 14 April 2021)
Research Articles
Response of juvenile progeny of seven forest tree species and their populations to simulated climate change-related stressors, heat, elevated humidity and drought
vol. 11, pp. 374-388 (online: 15 May 2018)
Research Articles
Diurnal dynamics of water transport, storage and hydraulic conductivity in pine trees under seasonal drought
vol. 9, pp. 710-719 (online: 21 August 2016)
Research Articles
Effects of mild drought on the morphology of sun and shade needles in 20-year-old Norway spruce trees
vol. 12, pp. 27-34 (online: 10 January 2019)
iForest Database Search
Search By Author
Search By Keyword
Google Scholar Search
Citing Articles
Search By Author
Search By Keywords
PubMed Search
Search By Author
Search By Keyword