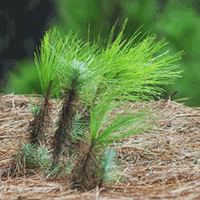
Matching seedling size to planting conditions: interactive response with soil moisture
iForest - Biogeosciences and Forestry, Volume 12, Issue 2, Pages 220-225 (2019)
doi: https://doi.org/10.3832/ifor2801-012
Published: Apr 25, 2019 - Copyright © 2019 SISEF
Research Articles
Abstract
Seedling size is a very important issue when producing plants for restoration projects. Scientific evidence on the appropriate size for drylands is contradictory. Thus, the aim of this study was to evaluate the effect of seedling size during first establishment by conducting a short term greenhouse experiment with Pinus canariensis containerized seedlings. A selection of large (mean height: 33.7 cm) and small (14.3 cm) one-year-old seedlings were planted in pots under two volumetric water content regimes: dry (7%) and wet (15%). Midday shoot water potential was measured in two periods: 10 (prior to root protrusion) and 30 (once the roots had protruded from the plug) days after planting. The length of protruding roots was measured after 30 days. One month after planting, the large seedlings under the dry regime produced more new roots than the small seedlings, but also showed the highest midday water potential values. Therefore, the greater root growth of the former did not offset the higher transpiration demand when planted in dry soils. These results suggest that under uncertainty about the soil humidity levels of dry areas, using small seedlings can improve their short-term survival after planting.
Keywords
Planting Survival, Root Water Uptake, Seedling Size, Seedling Ecophysiology, Transpiration Demand, Water Potential, Seedling Morphology
Introduction
The size of containerized nursery seedlings has been recognized as a major component of plant quality and establishment success in forestation projects. Much literature on different biomes has shown the importance of morphological size-related attributes for the survival of planted seedlings ([8] and references therein). However, there is still a lack of consensus on what size best fits key environmental factors for each biome ([5]). This is particularly an issue for dry sites ([3]) which are one of the most challenging areas for restoration ([41], [29]). In Mediterranean areas with a wet period in the autumn and winter months (when planting is carried out), a vigorous root growth of seedlings is crucial to their survival during the intensely dry summer season ([27]). For this biome, an ecophysiological model stressing the importance of nutrients and carbohydrate remobilization and photosynthesis for root growth has been developed to explain why large seedlings survive better ([43]). However, in very dry areas, or during dry planting years when access to water is uncertain, the most appropriate seedling size for planting continues to be a matter of debate. Under these environmental conditions, planting small seedlings is considered to be a conservative strategy that can be expedient insofar as planting shock is reduced by minimizing shoot water losses ([17], [38], [3], [26]). Notwithstanding this, the specific literature on dry areas is somewhat contradictory, with studies showing that seedling size has positive ([30], [43], [45], [25], [40]) or negative effects ([37], [48], [3], [12]) or both ([22]) on planting performance.
These contradictory results stem from the excessive dependence of performance on study conditions: although the outcome of the trade-off between root growth (a large seedling implies more root growth) and transpiration rate (higher in larger shoots) in dry areas depends to a large extent on soil moisture at transplanting ([8]), there are also other environmental factors, such as wind, temperature and solar radiation, which should be borne in mind. Forestation experiments under field conditions in dry areas often do not provide clear evidence because the aforementioned environmental factors override the effect of plant size. As a consequence, our ability to predict seedling survival in drylands is still poor ([3]). We believe that in order to validate and generalize models there is a need for experimental evidence based on the comparison of large vs. small seedlings under controlled soil moisture conditions. This could help to explain which circumstances lead to small seedlings having a more favorable response ([28]). Experiments assessing the interaction between soil moisture and seedling size when planting containerized seedlings are still few in the literature ([32], [34], [17], [13], [44], [47]), and those carried out did not simultaneously analyze seedling root growth and water status. Most of these studies have been designed in such a way that seedling size factor is confounded with the stock type (i.e., bareroot vs. container), container shape, plant age or hardening treatments. Root growth right after planting is critical for minimizing transplant shock ([7]) and surviving the dry soil season ([43]). Assessing the root growth of different stock sizes under a gradient of soil moisture would help to explain the response of planted seedlings under drought stress. Accordingly, the aim of this experiment was to provide a physiological basis for the selection of the most appropriate seedling size for planting in dry areas, with the following specific objectives in mind: (i) to compare large vs. small seedling water potential during the first weeks (30 days) after planting in drought conditions vs. field capacity levels; (ii) to assess the root growth of large vs. small seedlings as affected by soil moisture at planting. We hypothesize that: (1) freshly planted large seedlings under drought conditions will be more water stressed than small seedlings; and (2) the root growth of large seedlings will be reduced under dry conditions. In summary, this study examines the short-term performance of two seedling sizes (large and small) planted in a greenhouse controlled experiment under two soil moisture regimes.
Materials and methods
Plant material and planting experiment
The plant material was obtained from seedlings produced in 230 cm3 containers (SuperLeachTM, Industrias Bardi S.A.L., Navarre, Spain) filled with a 3:1 (v:v) Sphagnum peat (Kekkilä B0, Finland) and perlite mixture with 5 g l-1 of Osmocote Plus 15-11-13 + 2MgO controlled release fertilizer (Scotts, Marysville, OH, USA). Containerized seedlings were chosen as they are most commonly used in dry planting areas ([6]). Seeds from Pinus canariensis Chr. Sm. ex DC - a native species from the Canary Islands that thrives in dry areas ([20]) - were sown in April and May 2013 using the same seed lot. From May to the end of October, the seedlings remained outdoors at the School of Forestry’s nursery, Madrid, Spain (lat. 40° 27′ 06″ N, long. 03° 43′ 23″ W, elevation 657 m a.s.l.). On 30th October 2013, 30 seedlings of each size were selected for the study sample. The small seedlings were chosen from among the population of P. canariensis sown in May, whereas the large ones came from the trays sown in April. The small seedlings had a height (length of stem) of between 9 and 19 cm and the large seedlings between 28 and 44 cm. The average height and coefficient of variation (CV) was 14.3 cm (CV = 19.8%) for the small seedlings and 33.7 cm (CV = 11.5%) for the large ones.
The 60 selected seedlings received a saturating irrigation on November 3rd, 2013. They were then transplanted on November 4th to 3.5 l (16 × 22 cm, diameter × height) pots filled with a 1:2 (v:v) washed river sand and Sphagnum peat (Kekkilä Professional OPM420 Natural, Finland) mixture. Pots were randomly placed on a bench in the greenhouse, with a glass transmittance of 47%, at the School of Forestry. During the experiment, the temperature was kept between 15 (night) and 25 (day) °C. Once in the greenhouse, half of the seedlings of each size were randomly assigned to one of the two soil moisture regimes. Therefore, the experiment had a two-factor factorial design: seedling size (large and small) and soil moisture (dry and wet). The volumetric water content (VWC) of the growing medium was maintained at 7% (dry) and 15% (wet) throughout the study period. The 7% target corresponds to soil moisture values at a depth of 0-15 cm in dry areas during the planting season ([24]). This value causes a high level of water stress that significantly reduces seedling stomatal conductance and photosynthesis in peat-based substrates ([34], [31]). In contrast, 15% corresponds to the field capacity of the peat-sand growing medium employed. This was achieved by saturating the pots and letting the water percolate through the bottom holes (personal observation).
Measurements
To maintain the target levels of soil moisture, the VWC of each pot’s medium was measured immediately after transplanting by using time domain reflectometry (TDR) with a Hydrosense CS620® Water Content Reflectometer (Campbell Scientific Inc., USA). A pair of 20-cm rods were vertically inserted twice from the top of the pots and the measurements averaged. Following this, the water volume required to restore the moisture target level of the potting medium was added. The VWC was measured again after drainage (when this occurred) and new amounts of water were added (when necessary) in a trial and error process. Once the VWC had reached the target value, the pots were weighed and the reference weight for each pot and its soil moisture level were recorded. Successive irrigations were conducted using a gravimetric procedure ([9]). The pots were weighed every two days and water was added as needed to meet the target weight. The gravimetric approach was preferred over TDR to avoid possible damage to protruding roots and alterations in the growing medium structure due to the repeated rod insertions. In order to check that the VWC was within the target values throughout the experiment, 10 days after planting (November 13) TDR measurements were taken again in all the pots. The deviation from the VWC target was minimum, with significant differences by soil moisture treatment but not by seedling size or interactions (Tab. 1). This is particularly important in experiments of this type in which a regression artefact can occur because transpiration rates vary according to shoot size, thus affecting soil moisture ([32]).
Tab. 1 - ANOVA results and mean values (± standard error) of soil volumetric water content (VWC) as affected by seedling size and soil moisture regime (n = 30 per factor level) 10 days after transplanting. ANOVA was conducted with log transformed data, but back transformed VWC means are presented for clarity.
Source of variation | F | P>F | Seedling size/ Treatment |
Soil VWC (%) |
---|---|---|---|---|
Seedling size (SS) | 1.544 | 0.219 | Large | 11.4 ± 0.8 |
Small | 12.2 ± 0.8 | |||
Soil moisture (SM) | 116.402 | <0.001 | Dry | 8.3 ± 0.3 |
Wet | 15.3 ± 0.6 | |||
SS × SM | 0.029 | 0.075 | - | - |
The study period covered the first weeks after planting in which the first roots protrude from the plug and their subsequent coupling with the soil-atmosphere system occurs ([7]). The response variables evaluated were root growth and shoot water potential. Both were measured simultaneously twice during the experiment. Water potential was chosen as a surrogate of water stress that may occur when transpiration demand exceeds the ability of root systems to uptake and transport water ([4]). To characterize the water stress levels of the seedlings, midday xylem water potential was measured twice: 10 days after planting (DAP), before the protrusion of any root from the plug; and 30 DAP, when the seedlings had developed a significant amount of root tissue outside the plug. Midday water potential (ψMD) was measured in six (10 DAP) or eight-nine (30 DAP) randomly selected seedlings per treatment (factor combination) as follows: at 14:00h, the shoots of all the pine seedlings were excised from the root collar diameter and stored in plastic bags in a cooler until measurement. Shoot water potential was measured within one hour in a pressurized chamber (Model 600®, PMS Instruments, Inc., Corvallis, OR, USA). Midday water potential was chosen because it closely reflects the maximum values of water stress in plants during a daily cycle ([1], [15], [16]). Root development can also affect the ability to transport water to seedlings and, in turn, plant water status. Thus, plugs of the same seedlings used for water potential measurements were lifted from the pots to assess the length of new root growth. At 10 DAP, no roots longer than 0.5 cm had protruded from the plugs. One month after planting, all new roots protruding from the plug were excised and their total length was measured with a ruler. Additionally, seedling dry mass from the 30 DAP water potential assessment was measured after carefully washing root systems (excluding new protruding roots) to remove the medium and oven drying roots and shoots for 48 h at 65 °C.
Data analysis
A two-way analysis of variance (ANOVA) was performed on the data, with soil moisture and seedling size as fixed factors and a seedling serving as the experimental unit. Data homocedasticity was checked using Levenne’s test. When the ANOVA assumptions were not met (VWC, midday water potential, root and shoot biomass 30 DAP and new root length), the data were log-transformed. When the ANOVA showed significance for the interaction between factors, the differences among means were identified by a Tukey post-hoc test. Effects were considered significant when P<0.05. Throughout this work, the significance level for each ANOVA (P) is presented with degrees of freedom for each factor (df1) and for error term (df2) as subscripts (Pdf1, df2). All the analyses were carried out with the SPSS® ver. 19.0 statistical package (SPSS Inc., Chicago, IL, USA).
Results
On average, the ψMD of seedlings transplanted in humid soil decreased from -1.10 ± 0.05 (SE) to -1.45 ± 0.05 MPa and in dry soil from -1.00 ± 0.05 to -1.83 ± 0.11 MPa between the 10th and the 30th DAP, respectively. However, the variation pattern of this trait by factor was date dependent (Tab. 2). While in the 10th DAP no significant differences were found in soil moisture, seedling size or interactions (Fig. 1a), by 30 DAP the measured ψMD was significantly affected by seedling size × soil moisture interaction. The large seedlings planted in the dry substrate for at least 30 days were more stressed than the small seedlings under the same soil conditions. On the other hand, the ψMD of the seedlings planted for 30 days in wet conditions was unaffected by their size and was not significantly different from that of the small seedlings planted in dry soil (Fig. 1b).
Tab. 2 - ANOVA results for midday water potential prior (10 DAP) and after (30 DAP) new root growth outside the plug, and length of new roots outside the plug 30 DAP in relation to seedling size and soil moisture regime. (*): For midday water potential 30 DAP and new root length, ANOVA was conducted with log transformed data.
Variable | Source of variation | F | P>F |
---|---|---|---|
Midday water potential (ψMD) 10 DAP (df1 =1; df2 = 20) |
Seedling size (SS) | 0.624 | 0.439 |
Soil Moisture (SM) | 2.306 | 0.145 | |
SS × SM | 0.181 | 0.675 | |
Midday water potential (ψMD) 30 DAP*(df1 = 1; df2 = 30) |
Seedling size (SS) | 5.382 | 0.027 |
Soil Moisture (SM) | 0.001 | 0.001 | |
SS × SM | 4.371 | 0.045 | |
New root length 30 DAP*(df1 =1; df2 = 20) |
Seedling size (SS) | 9.598 | 0.006 |
Soil Moisture (SM) | 0.013 | 0.912 | |
SS × SM | 1.576 | 0.224 |
Fig. 1 - Midday water potential (ψMD ± SE) of large and small one-year-old Pinus canariensis seedlings (a) 10 (n = 6) or (b) 30 (n = 8-9) days after planting (DAP) as affected by soil moisture: Wet (15% VWC) and Dry (7% VWC). Different letters among treatments for 30 DAP (b) denote significant differences (p<0.05) according to the Tukey post-hoc test.
No significant root growth had occurred 10 DAP (as revealed by seedlings extracted at this date for water potential measurements - data not shown), but after 30 days root growth protruding from the plugs was noticeable. Interestingly, by this time the total length of these emerging roots was only significantly affected by seedling size (Tab. 2), with the large seedlings producing longer roots (Fig. 2). The shoot or root biomass 30 DAP was affected by seedling size (P1, 28 < 0.001, data log-transformed), but not by soil moisture or its interactions. At this point, the shoot and root biomass of the large seedlings (1.5 ± 0.1 and 2.4 ± 0.2 g, respectively) was three times greater than that of the small seedlings (0.5 ± 0.1 and 0.8 ± 0.1 g, respectively). By then, a significant seedling size × soil moisture interaction had occurred for the shoot/root ratio (P1, 28 =0.022), with all ratios being similar (0.65 ± 0.03 on average) except for the small seedlings planted in wet soils, whose ratio dropped to 0.42 ± 0.06.
Fig. 2 - Length of new roots (± SE, n = 6) of large and small one-year-old Pinus canariensis seedlings protruding from the plug 30 days after planting under different soil moisture conditions: Wet (15% VWC) and dry (7% VWC). According to Tab. 2, only seedling size is significant.
Discussion
This study indicates that seedling size affects water stress levels after planting, but this effect is soil moisture dependent. Under dry soil conditions, the large seedlings showed high levels of water stress. Other field or controlled experiments have substantiated this relationship between seedling size and water stress ([18], [26]), but only a few of them have analyzed how the relationship between seedling size and soil moisture impacts ψMD ([34], [17]). Furthermore, the controlled conditions experiment performed here shows that large seedlings are not more stressed during the first weeks after planting than small ones if soil moisture is high. It also provides an experimental basis for the model constructed by Villar-Salvador et al. ([43]), stating that large containerized seedlings do not experience planting shock, provided that the soil is moist. Moving on, the results obtained here show that during the first 10 DAP, seedling water status was unaffected by seedling size, soil moisture or its interaction, despite the significant differences in soil VWC at the time (Tab. 1). This indicates the positive role of a saturated plug in reducing immediate planting shock ([6]) even under dry postplanting conditions. However, this effect is ephemeral: water either diffuses through the saturated plug to the adjacent soil ([10]) or is taken up by the roots within the plug. This depletion of water reserves was reflected in the water potential reduction of seedlings from 10 to 30 DAP even in wet soil (Fig. 1 and Results). In a similar experiment, containerized seedlings under dry conditions also took four weeks to reach a significantly lower water potential than seedlings under well-watered conditions ([13]). The rate at which plug water reserves are depleted will depend not only on the transpiration demand of the seedlings, but also on soil water content. Dry soils will generate a more intense water potential gradient from plug to soil or substrate ([34], [10]). Our results also underline the relevance of shoot size over shoot/root ratio for water balance at the planting stage. The higher evaporative demands of larger shoots are not offset by larger root systems within the plug. This agrees with other studies that have played down the importance of the shoot/root ratio as an estimator of water stress avoidance for containerized seedlings ([8] and references therein). Regardless of the root system size or the new growth of roots protruding from the plug, large shoot seedlings planted in dry soils will be more prone to suffer from water stress than small ones, as they deplete plug water reserves sooner. Moreover, coarse-textured soils have a lower conductance than fine-textured ones when water is scarce ([21]). Thus, a higher level of water stress can be expected when planting takes place in coarse textured dry soils such as the potting medium employed in this study ([2]).
The new root growth of the large seedlings during the first weeks of establishment was higher than that of the small seedlings, irrespective of soil moisture. Therefore, soil moisture did not limit the root growth of the large seedlings during the study period. The depleting effect of soil drought on root growth could take longer to become significant; this effect was detected after eight weeks in a controlled experiment with Pinus taeda L. ([36]). Under water scarcity, root growth can be inhibited by, among other factors, high water stress levels in the plant that preclude cell division ([16]) and carbon gain ([46]). As shown by the root growth rates of the large seedlings in the dry soils used in this study, the ψMD 30 DAP was not yet so low as to preclude stomata closure and cell division, despite the low soil moisture of the 7% VWC treatment. The soil water potential limit at which plants are able to regenerate new roots after transplanting depends to a great extent on soil moisture, temperature ([35]) and the species ([14]).
According to the literature, the swiftness with which seedlings are able to establish new roots in the soil is key to reducing water stress and transplant shock ([7], [43]). However, it has been shown here that this is not the case with the largest seedlings under drought conditions, since they produce more new roots than smaller sized ones but, at the same time, register the lowest ψMD. Large seedlings can produce more roots and reduce water stress after transplanting, but only if soil moisture is high ([11], [15]). In this study, the water requirements of the large seedlings was not offset by the improved uptake of a new extended root system exploring dry soil and, accordingly, planting shock was not reduced as indicated by the lower values of the ψMD. The small seedlings planted in dry soils showed minimum root growth 30 DAP, but their ψMD was significantly higher than that of the large seedlings and equal to that of the seedlings planted in wet conditions (Fig. 1b). This stresses the crucial role of a saturated plug to supply water during the first weeks after transplanting. Finally, as small seedlings do not grow roots neither in humid nor in dry soils, the results of this study emphasize the importance of seedling size in promoting root growth shortly after planting.
This transplanting experiment was carried out under controlled conditions in a growing medium with a low hydraulic conductivity that favored the growth of new roots. The results point to the high capacity of large seedlings to produce new roots even under dry conditions, with minimum values of ψMD (maximum water stress). Field conditions involve a much more complex set of environmental factors affecting plant hydraulics and physiology than the controlled conditions of this experiment. However, the results provide some information with which to model the response of large vs. small seedlings transplanted in dry areas. Some authors claim that the success of large vs. small seedlings is dependent, among other factors, on the time between planting and the next rain event ([39], [3]). Water potential decreased over the study period, but as already remarked, this drop was especially intense for large seedlings under drought conditions, despite the growth of new protruding roots. This effect makes them more prone to hydraulic failure or starvation ([15], [21]) during the initial weeks. On the contrary, the small seedlings were more capable of reducing planting shock than the large ones, maintaining values of ψMD as high as those under wet conditions for at least one month. Additionally, the water potential of the large seedlings under dry conditions dropped to -2.01 ± 0.14 MPa 30 DAP, equivalent to a 12% loss of hydraulic conductance, when embolism begins for this species ([19]). However, under field conditions, direct radiation, winds or high temperatures could increase the evaporative demands of seedling or reduce their tolerance to water stress ([21], [23]). If a rain event occurs or seedlings reach a wet soil horizon before cavitation, large seedlings could take advantage of their more developed root systems and avoid summer drought stress damage by connecting their roots to deeper, ever-moist horizons ([27]). But in a rainless scenario after planting, the specific functional water-saving or water-spending strategy of the seedling species becomes important ([16]) and interacts with size. Thus, we expect that the potential negative effects of the size of seedlings on their water status may be lower in water-saver than in water-spender plants ([33], [43], [42]).
Conclusions
The study results, besides providing an empirical basis, contribute to propose practical guidelines for selecting seedlings for forestation projects in dry areas. The main idea is the overriding importance of size when planting seedlings in dry soils, with large seedlings experiencing lower water potential levels despite their larger root systems produced under dry conditions. The results of forestation in dry lands could be improved by adapting seedling size to the specific environmental conditions of the planting site, with a tendency towards using small seedlings, especially in areas with high evaporative demands at planting. Additional research should be conducted to refine the understanding of plant response under dry conditions and to achieving a better match between seedling quality and particular restoration environments. Future controlled and field studies of plant size and planting response should analyze key interactions between specific functional traits (plant hydraulics, water use strategy, foliar habits and the ability to produce new roots in dry soils) and environmental conditions at planting, specifically vapor pressure deficit and certain soil characteristics (texture, water retention capacity and hydraulic conductance).
Acknowledgments
The study was supported by the AGL2011-24296 ECOLPIN project (Department of Science and Innovation, Spanish Government) and the PhD educational program of the Technical University of Madrid, Spain.
Author contributions
JAO conceived and designed the research; EOU, MSP, GTC performed the experiments, JAO, EOU, MSP, GTC analyzed the data; JAO wrote the manuscript; MSP, GTC edited the manuscript.
References
Gscholar
Gscholar
Gscholar
Gscholar
CrossRef | Gscholar
Authors’ Info
Authors’ Affiliation
Esther Ortiz de Urbina
Guillermo Tardío-Cerrillo
Escuela Técnica Superior de Ingeniería de Montes, Forestal y del Medio Natural, Universidad Politécnica de Madrid, Ciudad Universitaria, 28040 Madrid (Spain)
Forest Sciences Centre of Catalonia (CEMFOR-CTFC), 25280 Solsona (Spain)
Corresponding author
Paper Info
Citation
Oliet JA, Ortiz de Urbina E, Sánchez-Pinillos M, Tardío-Cerrillo G (2019). Matching seedling size to planting conditions: interactive response with soil moisture. iForest 12: 220-225. - doi: 10.3832/ifor2801-012
Academic Editor
Rossella Guerrieri
Paper history
Received: Mar 29, 2018
Accepted: Feb 22, 2019
First online: Apr 25, 2019
Publication Date: Apr 30, 2019
Publication Time: 2.07 months
Copyright Information
© SISEF - The Italian Society of Silviculture and Forest Ecology 2019
Open Access
This article is distributed under the terms of the Creative Commons Attribution-Non Commercial 4.0 International (https://creativecommons.org/licenses/by-nc/4.0/), which permits unrestricted use, distribution, and reproduction in any medium, provided you give appropriate credit to the original author(s) and the source, provide a link to the Creative Commons license, and indicate if changes were made.
Web Metrics
Breakdown by View Type
Article Usage
Total Article Views: 42948
(from publication date up to now)
Breakdown by View Type
HTML Page Views: 36609
Abstract Page Views: 2879
PDF Downloads: 2579
Citation/Reference Downloads: 5
XML Downloads: 876
Web Metrics
Days since publication: 2270
Overall contacts: 42948
Avg. contacts per week: 132.44
Article Citations
Article citations are based on data periodically collected from the Clarivate Web of Science web site
(last update: Mar 2025)
Total number of cites (since 2019): 8
Average cites per year: 1.14
Publication Metrics
by Dimensions ©
Articles citing this article
List of the papers citing this article based on CrossRef Cited-by.
Related Contents
iForest Similar Articles
Research Articles
Optimum light transmittance for seed germination and early seedling recruitment of Pinus koraiensis: implications for natural regeneration
vol. 8, pp. 853-859 (online: 22 May 2015)
Research Articles
Effects of cultural treatments, seedling type and morphological characteristics on survival and growth of wild cherry seedlings in Turkey
vol. 5, pp. 283-289 (online: 17 December 2012)
Research Articles
Addressing post-transplant summer water stress in Pinus pinea and Quercus ilex seedlings
vol. 8, pp. 348-358 (online: 16 September 2014)
Research Articles
Oak sprouts grow better than seedlings under drought stress
vol. 9, pp. 529-535 (online: 17 March 2016)
Technical Reports
Nursery practices increase seedling performance on nutrient-poor soils in Swietenia humilis
vol. 8, pp. 552-557 (online: 09 December 2014)
Research Articles
Use of alternative containers for promoting deep rooting of native forest species used for dryland restoration: the case of Acacia caven
vol. 10, pp. 776-782 (online: 02 September 2017)
Research Articles
Effects of soil compaction on seedling morphology, growth, and architecture of chestnut-leaved oak (Quercus castaneifolia)
vol. 10, pp. 145-153 (online: 13 June 2016)
Research Articles
Forecasting the field performance of Austrian pine seedlings using morphological attributes
vol. 10, pp. 99-107 (online: 13 October 2016)
Research Articles
Size and age: intrinsic confounding factors affecting the responses to a water deficit in black spruce seedlings
vol. 8, pp. 401-409 (online: 09 December 2014)
Research Articles
Linking nursery nutritional status and water availability post-planting under intense summer drought: the case of a South American Mediterranean tree species
vol. 9, pp. 758-765 (online: 03 June 2016)
iForest Database Search
Google Scholar Search
Citing Articles
Search By Author
Search By Keywords