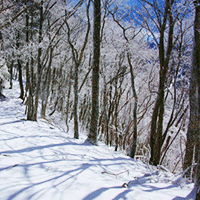
The losses of condensed tannins in six foliar litters vary with gap position and season in an alpine forest
iForest - Biogeosciences and Forestry, Volume 9, Issue 6, Pages 910-918 (2016)
doi: https://doi.org/10.3832/ifor1738-009
Published: Aug 04, 2016 - Copyright © 2016 SISEF
Research Articles
Abstract
Condensed tannins (CTs) have been considered to be intrinsic variables that determine litter decomposition. Forest gaps and the surrounding crown canopies may locally influence the microenvironmental factors, thus affecting the losses of CTs from litter. However, little information is available about the dynamics of CTs loss in forest gaps. In this study, litterbags containing foliar litter of Minjiang fir (Abies faxoniana), red birch (Betula albosinensis), Masters larch (Larix mastersiana), cypress (Sabina saltuaria), Kangding willow (Salix paraplesia), and Lapland azalea (Rhododendron lapponicum), were placed on the forest floor at differet positions from the gap center to the closed canopy in the alpine Minjiang fir forest located in the upper reaches of the Yangtze River and the eastern Tibetan Plateau (China). The samples were retrieved during the periods of snow formation, snow cover, snow melt and in the growing season, and the CTs content was measured at each time point. During the first year, all six types of foliar litter experienced high losses of CTs with values ranging from 70.18% to 96.67%. Forest gaps accelerated litter CTs losses in the winter but inhibited CTs losses in the growing season, which demonstrated significant seasonal differences. Additionally, the litter of conifers exhibited greater CTs losses in the winter, especially during the snow formation period, whereas the litter of broadleaved trees showed greater CTs losses during the growing season. These results indicate that the predicted reductions in snow depth resulting from future winter warming and the loss of forest gaps due to forest regeneration will inhibit the decomposition of CTs in the litter of alpine forest ecosystems, which will slow soil carbon sequestration from foliar litter in cold biomes.
Keywords
Forest Gap, Condensed Tannins, Foliar Litter, Seasonal Snowpack, Alpine Forest
Introduction
Condensed tannins (CTs) are widely occurring plant polyphenols ([16], [13]) which play a critical role in material cycling and energy flow in forest ecosystems ([30], [42], [43]). Because CTs inhibit feeding, their concentration in aboveground plant tissues is proportional to the level of herbivory ([8], [15]), and it has also been suggested to be a good predictor of litter decomposition ([19], [22]). Litter CTs are rapidly lost in the initial decomposition period due to leaching and chemical modification ([21], [43]), but they retard the rate of litter decomposition in the late stage through the formation of recalcitrant complexes resulting from protein and cellulose precipitation, oxidative condensation ([13]), herbivory inhibition and fungal degradation through toxicity ([8], [12], [15]). Therefore, the dynamics of CTs loss could be related to the litter decomposition process and could depend on both environmental factors and the initial substrate. Previous studies have primarily focused on determining the structure of CTs and the changes in CTs during the decomposition of the litter of a single species ([29], [27]). However, knowledge of the losses of plant litter CTs in alpine forest ecosystems, which might elucidate the process of underground CTs loss, is still limited.
Gap formation by tree fall is a basic forest process altering climate factors and soil organisms, and affecting litter CTs loss in high-altitude forest ecosystems ([38], [32], [40]). In winter, the effects of light interception by crown canopies on snow accumulation and the influence of canopy shading on snow ablation could create a snowpack gradient on the forest floor from the gap center to the closed canopy ([41]). These gradients may vary with the seasons due to the cycles of snow cover freezing and thawing. In deep winter, snowpack depth decreases from the gap center to the closed canopy, which results from interception and deep freezing. Contrastingly, snowpack depth in late winter and early spring increases from the gap center to the closed canopy, as its shading prevents the rapid melting of the snow. Additionally, the interception of rainfall and sunlight by the crown canopies in the growing season often affects soil moisture and temperature dynamics ([28]). Theoretically, the effect of forest gaps on litter CTs loss might vary with both the positions of gaps relative to the crown canopies and the seasons, owing to the different dynamics of leaching, mechanical disruption and biological activities, but little information on the effect of forest gaps and crown canopies on the dynamics of litter CTs loss is available.
There are a variety of mechanisms that can induce significant differences in CTs losses between the gap center and the closed canopy over different decomposition periods. First, some hydrolysable CTs and other easily available nutrients in fresh litter are readily soluble and thus leached immediately ([30], [42]), which results in the rapid loss of CTs in the early period of decomposition, even in winter. Second, during the snow cover and melting periods, the deep snowpack located in the gap center can provide the stable conditions required for degradation and rapid leaching, which result in greater litter CTs losses ([39], [30]). However, the loss of CTs is negatively affected by the increased exposure to sunlight and by the more rapid evaporation in the gap center during the growing season ([28]). In addition, different initial foliar litter substrates may have different effects on the varying degradation characteristics of different species ([2], [31]), which could exert a far-reaching influence on CTs losses. Previous studies have primarily focused on the loss of mass, the release of nutrients and the changes in degradation during litter decomposition in alpine forests in winter ([39], [41], [35], [36], [44], [45], [14]), but little information is available on the dynamics of litter CTs losses. Therefore, based on previous studies, we hypothesized that (1) litter CTs will be more variable under the stable microenvironments caused by deep snow cover in the gap center during winter, as well as under the suitable moisture and temperature conditions under closed canopies throughout the growing season; (2) the litter CTs losses will continue throughout the first year of this litter decomposition study, resulting in greater losses in winter.
We conducted a one-year field experiment to evaluate the loss of CTs from the foliar litter of an alpine forest located in the upper reaches of the Yangtze River and the eastern Tibetan Plateau (China). Six types of foliar litter were used, spanning a wide range of litter quality charateristics and including both conifers and broadleaved trees. Forest gaps and the associated crown canopies create winter snowpack gradient and freeze-thaw cycles in winter and redistribute precipitation, temperature and solar radiation during the growing season ([39], [40], [41], [14]). Previous studies conducted by our team investigated the litter decomposition dynamics in alpine forests, including the loss of mass, release of nutrients, response of humification and effects on microbial biomass, and we concluded that the deep snow patches caused by forest gaps promoted litter decomposition during winter ([35], [36], [45], [23], [24], [41]). Based on these results, we measured the litter CTs content and losses during different decomposition periods from the gap center to the closed canopy. The results are expected to characterize the effects of forest gaps on CTs losses from different types of foliar litter during litter decomposition in winter and the growing season, as well as to provide new information on material cycling in alpine forest ecosystems.
Materials and methods
Site description
This study was conducted at the Miyaluo Nature Reserve (102° 53′ - 102° 57′ E, 31° 14′ - 31° 19′ N, 2458-4619 m a.s.l.), which is located in Li County of Sichuan Province, Southwest China. It is a transitional area located in the upper reaches of the Yangtze River and the eastern Tibetan Plateau ([45]). The annual mean temperature of the study site is 2.7 °C with mean temperatures of -8.7 °C and 9.5 °C in January and July, respectively, and the annual mean precipitation is 850 mm. The freeze-thaw season generally extends from late October to late April, with a maximum snow depth of approximately 50 cm and a freeze-thaw cycle time of approximately 120 days ([34]). The tree canopy is dominated by Minjiang fir (Abies faxoniana), red birch (Betula albosinensis), Masters larch (Larix mastersiana) and cypress (Sabina saltuaria), while the most common shrubs in the understory are Kangding willow (Salix paraplesia), Lapland azalea (Rhododendron lapponicum), Berberis sargentiana, Sorbus rufopilosa, and Hippophae rhamnoides. The herbs are dominated by Cacalia spp., Cystopteris montana and a variety of mosses ([41], [24]).
Experimental design
The foliar litter decomposition was studied using the nylon mesh bag technique ([25]). In early October 2012, freshly senesced leaves of cypress, Minjiang fir, Masters larch, red birch, Kangding willow and Lapland azalea were collected from the floor of the study site; decayed and weak leaves were excluded. Samples of air-dried litter (10 g per bag) were placed in prepared nylon bags (20 × 25 cm in size, with mesh of 1.0 mm on top and 0.5 mm on the bottom). The chemical characteristics (CTs, C, N, P, lignin, cellulose, C/N, C/P, N/P, lignin/N) of the six types of initial litter substrate were analyzed (Tab. 1).
Tab. 1 - Initial quality (%) of the six types of foliar litter (cypress, Minjiang fir, Masters larch, red birch, Kangding willow, Lapland azalea) in an alpine Minjiang fir alpine forest (mean ± SD, n = 3). Different lowercase letters indicate a significant difference (P < 0.05) between species within the same variable (one-way ANOVA).
Species | CTs (%) |
C (%) |
N (%) |
P (%) |
Lignin (%) |
Cel (%) |
C/N | C/P | N/P | Lignin/N |
---|---|---|---|---|---|---|---|---|---|---|
Cypress | 3.45 ± 0.06 b |
51.64 ± 1.77 bc |
0.88 ± 0.01 b |
0.12 ± 0.01 cd |
14.07 ± 0.74 b |
12.22 ± 0.38 a |
58.86 ± 2.21 b |
416.02 ± 14.04 a |
7.08 ± 0.41 ab |
16.04 ± 1.01 a |
Minjiang fir | 12.20 ± 0.39 c |
50.56 ± 2.96 b |
0.88 ± 0.01 b |
0.11 ± 0.01 bc |
15.85 ± 0.36 b |
12.19 ± 0.20 b |
57.77 ± 3.53 b |
443.51 ± 36.69 ab |
7.68 ± 0.72 b |
18.11 ± 0.42 b |
Masters larch | 3.08 ± 0.06 b |
54.35 ± 0.63 c |
0.86 ± 0.04 b |
0.13 ± 0.01 d |
27.21 ± 2.21 d |
16.45 ± 0.44 d |
63.32 ± 3.49 b |
407.08 ± 2.42 a |
6.44 ± 0.38 a |
25.95 ± 1.08 c |
Red birch | 0.28 ± 0.02 a |
49.69 ± 1.45 b |
1.33 ± 0.02 d |
0.09 ± 0.01 a |
36.68 ± 0.62 b |
12.47 ± 0.38 e |
37.24 ± 1.35 a |
544.94 ± 31.72 c |
14.63 ± 0.36 d |
25.99 ± 0.37 c |
Kangding willow | 0.23 ± 0.01 a |
45.23 ± 1.65 a |
1.15 ± 0.03 c |
0.11 ± 0.00 b |
20.22 ± 0.22 a |
10.45 ± 0.59 a |
39.49 ± 20.18 a |
408.12 ± 16.89 a |
10.34 ± 0.18 c |
17.66 ± 0.63 b |
Lapland azalea | 22.49 ± 1.13 d |
50.29 ± 1.60 b |
0.67 ± 0.02 a |
0.11 ± 0.01 b |
20.81 ± 0.18 c |
14.07 ± 0.41 c |
75.54 ± 40.47 c |
471.14 ± 42.04 b |
6.25 ± 0.25 a |
31.24 ± 0.69 d |
Based on the field investigation and previous locally-collected data, three forest gaps greater than 25 × 25 m with similar canopy densities were randomly selected within a representative Minjiang fir forest nature reserve (31° 14′ N, 102° 53′ E, 3579-3582 m). Four experimental positions were evenly distributed within each gap from the gap center to the closed canopy (gap center, canopy gap, expanded gap, and closed canopy), and four 2 × 2-m sampling blocks for the six litter species were established in each position at 2-m intervals to ensure adequate sampling of heterogeneous micro-environmental conditions. A total of 864 litterbags (3 gaps × 4 positions × 6 species × 4 sampling dates × 3 replicates) were placed on the forest floor from the gap center to the closed canopy on November 15, 2012. The temperature in the litterbags was measured every 2-hours using iButton recorders (iButton DS1923-F5, Maxim/Dallas Semiconductor, Sunnyvale, CA, USA) placed in two litterbags in each gap position. The air temperature and the temperature in the litterbags from November 15, 2012 to October 30, 2013 are displayed in Fig. 1. The values of the following factors were calculated to clearly describe the temperature characteristics: daily mean temperature (DMT), positive accumulated temperature (PAT, sum of temperatures above 0 °C), negative accumulated temperature (NAT, sum of temperatures below 0 °C - [14], [23], [24]) and the frequency of soil freeze-thaw cycles (FSFC, the total number of times the temperature changed from above/below 0 °C to below/above 0 °C in a 3-hour period, [18] - Tab. 2).
Fig. 1 - Daily mean air and soil surface temperature of four gap positions (gap center, canopy gap, expanded gap, closed gap) during the first year of litter decomposition year in an alpine Minjiang fir forest (from November 15, 2012 to October 30, 2013). The light grey region indicates the freeze-thaw season (from November 15, 2012 to April 24, 2013 - n = 6).
Tab. 2 - Characteristics of the daily mean temperature (DMT, °C), positive accumulated temperature (PAT, °C), negative accumulated temperature (NAT,°C) and frequency of soil freeze-thaw cycles (FSFC, times) across each decomposition period of the four gap positions (gap center, canopy gap, expanded gap, closed gap) over the first year of litter decomposition in an alpine Minjiang fir forest.
Gap Position | Temp. Variable |
Snow Formation Stage | Snow Cover Stage | Snow Melt Stage | All winter |
Growing season |
1st year |
---|---|---|---|---|---|---|---|
Gap center | DMT | -3.97 | -3.97 | 2.14 | -1.97 | 9.62 | 4.11 |
PAT | 378.47 | 1004.85 | 1604.30 | 2987.62 | 20713.40 | 23701.02 | |
NAT | -2296.28 | -4433.95 | -482.58 | -7212.81 | -217.40 | -7430.21 | |
FSFC | 43 | 75 | 54 | 172 | - | 172 | |
Canopy gap | DMT | -2.90 | -3.25 | 1.13 | -1.77 | 7.81 | 3.43 |
PAT | 408.92 | 1066.50 | 922.70 | 2398.12 | 17454.67 | 19853.79 | |
NAT | -1799.92 | -3875.67 | -287.33 | -5962.92 | -98.00 | -6061.92 | |
FSFC | 56 | 100 | 29 | 185 | - | 185 | |
Expanded gap | DMT | -3.46 | -4.15 | 1.82 | -2.22 | 7.87 | 3.26 |
PAT | 356.75 | 1039.04 | 1418.50 | 2814.29 | 17971.93 | 20786.22 | |
NAT | -2017.08 | -4624.67 | -391.25 | -7033.00 | -140.25 | -7173.25 | |
FSFC | 50 | 92 | 49 | 191 | - | 191 | |
Closed canopy | DMT | -3.54 | -3.67 | 1.24 | -2.15 | 7.79 | 3.29 |
PAT | 448.50 | 1328.92 | 1005.50 | 2782.92 | 17598.58 | 20382.50 | |
NAT | -2149.17 | -4501.00 | -305.08 | -6955.25 | -61.89 | -7017.14 | |
FSFC | 59 | 105 | 38 | 201 | - | 201 |
To quantify the CTs contents in the litter at different critical periods, which were identified based on the Olsson’s division of cold season periods ([26]) and the field investigations from our previous studies ([45], [41], [24]), we sampled the litterbags on 26 December 2012 (snow formation period, SFP - 41 days after the beginning of the experiment), 8 March 2013 (snow cover period, SCP - 72 days), 24 April 2013 (snow melt period, SMP - 47 days), and 30 October 2013 (growing season, GS - 189 days). Arthropods and foreign roots were removed, and the litterbags were returned to the lab for analysis. The depth of the snow cover was measured with a ruler at multiple points in each sampling date (Fig. 2).
Fig. 2 - Snow depth (cm) at the four gap positions (gap center, canopy gap, expanded gap, closed canopy) during the first year of litter decomposition in an alpine Minjiang fir forest (n = 3).
Analyses and calculations
After the roots and foreign materials were removed, the remaining litter was collected from the litterbags, and oven-dried at 65 °C for at least 48 hours to a constant weight. Samples were then very finely ground with a mill and passed through a 0.3-mm mesh. A conventional vanillin-HCl assay method was used to measure the CTs in the six types of foliar litter ([11]). A sample of foliar litter (200 ± 50 mg) was extracted with 5 ml of 70% methanol, maintained at room temperature for 24 hours and then centrifuged at 4 °C and 5000 r min-1 for 10 minutes. Next, 3 ml of a 4% vanillin methanol solution and 1.5 ml of HCl were added to 0.5 ml of the supernatant liquid; the solution was mixed well and reacted in a water bath at 20 ± 2 °C for 30 minutes, and the absorbance was measured at 510 nm. Catechin was used as a standard sample to draw the standard curve to calculate the extracted CTs content. All of the analyses were performed in triplicate. The loss of litter mass ([1]) and CTs ([33]) over time were calculated as follows (eqn. 1, eqn. 2):
where M
t and M
t-1 represent the remaining litter dry mass between the current (t) and previous (t-1) sampling dates, respectively; C
t and C
t-1 represent the CTs content (%) between the current and previous sampling dates, respectively; M
0 and C
0 are the initial litter dry mass and CTs content, respectively.
Statistical analyses
Differences in the initial substrate qualities, litter mass losses, CTs contents and CTs losses in the six types of foliar litter for each decomposition period among the four gap positions were evaluated using one-way ANOVA (analysis of variance) and LSD (least significant difference) test with α = 0.05. The effects of the gap positions, species and decomposition period on the loss of CTs were analyzed using a repeated measures ANOVA (Tab. 3). The influence of environmental factors (DMT, PAT, NAT, FSFC) and the initial substrate qualities (initial CTs, C, initial N, initial P, initial cellulose, initial lignin, initial C/N, initial C/P, initial N/P, initial lignin/N, initial cellulose/lignin) on the loss of CTs at each decomposition period were screened through a step-wise (backward) regression analysis (Tab. 4 - [24]). All of the statistical analyses were performed using the software package SPSS for Windows v. 17.0 (SPSS Inc., IL, USA). The data for all of the tests conformed to a normal distribution.
Tab. 3 - Results of the repeated measures ANOVA for the effects of period, gap position and species on litter condensed tannins loss (%) over the first year of litter decomposition in the alpine Minjiang fir forest (n = 72).
Source of variance |
df | F-value | P-value |
---|---|---|---|
Period (P) | 3 | 68.447 | 0.000 |
Gap position (G) | 3 | 1.232 | 0.308 |
Species (S) | 5 | 50.829 | 0.000 |
P×G | 9 | 13.930 | 0.000 |
P×S | 15 | 28.906 | 0.000 |
G×S | 15 | 3.592 | 0.000 |
P×G×S | 45 | 9.289 | 0.000 |
Tab. 4 - Determination coefficients (R2) and dominant factors (in parenthesis) of the step-wise regression analysis of litter condensed tannins loss, effected by environmental factors. (DMT): daily mean temperature; (PAT): positive accumulated temperature; (NAT): negative accumulated temperature; (FSFC): frequency of soil freeze-thaw cycles) and substrate qualities (Initial CTs/C/N/P…, initial quality of the foliar litter) at each decomposition period in the first year of litter decomposition in the alpine Minjiang fir forest. (CTs): condensed tannins; (Lig): lignin; (Cel): cellulose.
Parameter | Snow Formation Stage |
Snow Cover Stage |
Snow Melt Stage |
All of winter |
Growing Season |
1st year |
---|---|---|---|---|---|---|
DMT | - | 0.219 (2) | - | - | - | - |
PAT | 0.636 (4) | - | - | - | - | - |
NAT | - | - | - | - | - | - |
FSFC | 0.608 (3) | - | - | - | - | - |
Initial CTs | - | - | - | 0.712 (4) | - | - |
Initial C | - | - | 0.107 (1) | - | 0.211 (1) | - |
Initial N | 0.312 (1) | - | - | - | - | - |
Initial P | - | 0.112 (1) | - | 0.405 (1) | - | - |
Initial Lig | 0.511 (2) | - | - | 0.561 (2) | - | - |
Initial Cel | - | - | - | - | - | 0.631 (3) |
Initial C/N | - | - | - | - | - | - |
Initial C/P | - | - | - | - | - | - |
Initial N/P | - | - | - | 0.678 (3) | - | 0.525 (1) |
Initial Lig/N | - | - | - | - | 0.355 (2) | 0.606 (2) |
Results
Litter condensed tannins content
The initial CTs content was significantly higher in the conifers compared with the broadleaved trees, with the exception of Lapland azalea which had the highest initial CTs content (Tab. 1). Throughout the first year of this study, the CTs content of all six types of foliar litter decreased and eventually reached low levels (< 4%) in the growing season (Fig. 3), especially the CTs of the Lapland azalea and Minjiang fir litter, which declined by 18.51% and 11.51%, respectively.
Fig. 3 - Condensed tannins content (%) of the six types of foliar litter (cypress, Minjiang fir, Masters larch, red birch, Kangding willow, Lapland azalea) at the four gap positions (gap center, canopy gap, expanded gap, closed canopy) at each sampling time over the first year of litter decomposition in an alpine Minjiang fir forest. Different lowercase letters indicate significant differences (P < 0.05) between different gap positions for the same sampling time (mean ± SE, n = 3).
Litter condensed tannins loss
As shown in Tab. 3, litter CTs loss was considerably affected by sampling time, gap position, species and the interaction of these factors. Over the first year of decomposition, the litters exhibited an overall mass loss of 18.73 % to 46.80%, varying by species (Fig. 4); all six types of foliar litter exhibited significant CTs losses in the range of 70.18% to 96.57% of the initial values depending on the species (Fig. 5). The conifers showed a greater loss of CTs (92.87% to 96.57%) than broadleaved trees (70.18% to 85.98%), thus the initial substrate quality of the litter could be a major factors affecting CTs loss (Tab. 4). Additionally, a substantial loss of CTs from both conifers and broadleaved trees occurred both in winter and during the growing season. In the snow formation period, all of the studied litter showed higher losses of CTs in the gap center and in the canopy gap, with the exception of the red birch and Kangding willow litters, which only exhibited a decline in CTs in the gap center and an increase in the other gap positions. Except Lapland azalea, all other types of foliar litter exhibited the greatest losses of CTs in the canopy gaps or in the expanded gaps during the snow cover period. In the snow melt period, most foliar litter showed the greatest declines in CTs in the expanded gap or in the closed canopy, although the Lapland azalea litter did not show a significant decrease in CTs during this period. Overall, there were greater losses of CTs throughout the winter from litter that was primarily located in the gap centers and in the canopy gaps as compared with that in the expanded gaps and in the closed canopies. In contrast, the pattern of CTs loss was inconsistent among species throughout the growing season. The litters of Minjiang fir, Lapland azalea and red birch showed the greatest loss of CTs in the closed canopy, but cypress and Kangding willow exhibited greater losses of CTs in the canopy gap and in the expanded gap, while Masters larch showed the opposite pattern. There were non-significant differences in the one-year loss of CTs among the litters in the different gap positions, although the cypress and Lapland azalea litters showed greater losses in the gap center.
Fig. 4 - Litter mass loss (%) of the six types of foliar litter (cypress, Minjiang fir, Masters larch, red birch, Kangding willow, Lapland azalea) at the four gap positions (gap center, canopy gap, expanded gap, closed canopy) for each decomposition period (SFP: snow formation period; SCP: snow cover period; SMP: snow melt period; Winter: all of winter; GS: growing season; 1 st Year: the fist year of litter decomposition) over the first year of litter decomposition in an alpine Minjiang fir forest. Different lowercase letters indicate significant differences (P < 0.05) between different gap positions at the same decomposition period (mean ± SE, n = 3).
Fig. 5 - Condensed tannins loss (%) from the six types of foliar litter (cypress, Minjiang fir, Masters larch, red birch, Kangding willow, Lapland azalea) at the four gap positions (gap center, canopy gap, expanded gap, closed canopy) for each decomposition period (SFP: snow formation period; SCP: snow cover period; SMP: snow melt period; Winter: all of winter; GS: growing season; 1 st Year: the fist year of litter decomposition) over the first year of litter decomposition in an alpine Minjiang fir forest. Different lowercase letters indicate significant differences (P < 0.05) between different gap positions for the same decomposition period (mean ± SE, n = 3).
Factors influencing the loss of condensed tannins from litter
Based on the stepwise multiple regression correlations (Tab. 4), CTs losses were more strongly correlated with the initial chemical qualities of the litter than with the temperatures of each decomposition period, and all of these correlations were positive. The daily mean temperature was only correlated with CTs losses during the snow cover period, whereas the positive accumulated temperature and frequency of soil freeze-thaw cycles were both correlated with CTs losses during the snow formation period. Among the chemical qualities of the litter, the initial CTs, N and cellulose contents were correlated with CTs losses during the entire winter, the snow formation period and the first year. The initial CTs, P and cellulose contents and the N/P ratio were correlated with CTs losses throughout the entire winter, but the initial cellulose content and the N/P and lignin/N ratios were correlated with CTs losses during the first year. In addition, the initial P content and the N/P ratio exhibited strong correlations with CTs losses throughout the winter and the first year, respectively.
Discussion
Forest gaps and their surrounding crown canopies affect the snowpack depth in winter, as well as regulate the temperature, precipitation and solar radiation in the growing season ([41]), which may influence litter decomposition through intense leaching, freeze-thaw cycling and subsequently regulate and control CTs losses. Our results revealed that the loss of litter CTs was significantly affected by the gap position, species, season and their interactions over the first year of litter decomposition (Tab. 3).
As CTs molecules are complex and energetically costly to synthesize, their widespread occurrence and abundance suggest that they play an important role in the function and degradation of foliar litter and may slow the litter decomposition process ([43]). Throughout the experimental period, all six types of foliar litter exhibited high losses of CTs, and the differences among the different gap positions were not significant, except in the gap center; Minjiang fir showed a lower loss of CTs, while cypress and Lapland azalea exhibited a distinctively increased loss (Fig. 5). Such result suggests that the interspecific differences shown in Tab. 1 had a strong influence on CTs losses ([3], [4]). Indeed, this is consistent with the results of the stepwise regression analysis that proved a strong correlation between CTs losses and the chemical characteristics of the initial substrate (N/P, lignin/N, cellulose - Tab. 4). Leaching is an important mechanism driving the removal of CTs from litter, especially in the early decomposition period ([16], [43]). The fresh litter of late autumn ([9]) is characterized by relatively abundant available nutrients, and the rapid loss of soluble and low-molecular-weight compounds such as methanol-extractable CTs ([3], [20]) accounts for the rapid losses of CTs from all of the litters during the snow formation period in our study. During this period, strong eluviation occasionally occurred as a result of rain followed by rapid, deep snow coverage in the gap center, which caused a fast decrease in temperature, and this process might promote CTs leaching and microbial assimilation ([40], [41]) that would cause a greater loss of CTs from all of the litters in the gap center.
With the accumulation of snowfall and the low-temperature environment during the snow cover period, there were significant differences in the conditions for degradation among the different gap positions ([41]). Three types of conifer foliar litter showed greater CTs losses, which was consistent with previous results that indicated that snow cover can increase litter decomposition ([39], [40], [44]), but degradation or oxidation caused by microorganisms also plays an important role in litter CTs losses ([43]). For example, Enterobacter has the capacity to degrade CTs, and the actinomycete Debaryomyces hansenii can produce tannin-degrading enzymes ([5]). Thus, both enzymatic breakdown and the complexation of CTs can contribute to the loss of the extractable CTs in litter under snow coverage ([7]). The deep snow conditions in the gap center were favorable for CTs biodegradation because of the relatively warmer and wetter conditions, which supported a higher variety and activity of organisms ([37], [6], [41]). However, the intense freeze-thaw mechanical disruptions under the closed canopy were favorable to the complexation of CTs because they caused the production of additional proteins and cell-wall polysaccharides ([10]). Nonetheless, all of the foliar litter in our study (except for the Lapland azalea litter) exhibited higher CTs losses in the canopy gap and in the expanded gap, which implied that the combined action of the freeze-thaw mechanical disruptions and biodegradation could promote CTs losses during the snow cover period.
Along with increasing temperature, the intense leaching and frequent freeze-thaw cycles caused by melting snow play an important role in litter decomposition during the snow melt period ([39], [40]). Compared with the CTs in the gap center and in the canopy gap, the CTs of all of the foliar litter in the expanded gap and under the closed canopy exhibited greater losses during the snow melt period, which might have resulted from the slow though continuous leaching caused by the melting of sheltered snow that escaped solar radiation under the canopy crown. In addition, the increased moisture, which is beneficial to microbial activity, also led to the biodegradation of the CTs ([10], [17]).
The Growing season, which is characterized by warmer temperatures and sufficient moisture, is beneficial to the recovery of CTs decomposers ([7]), especially in the readily available litter of broadleaved trees ([4]). Therefore, the litter of all three types of broadleaved trees exhibited relatively higher losses of CTs during the growing season than in winter. Due to the extreme decomposition environment resulting from greater sunlight exposure, precipitation and more rapid evaporation in the gap center ([28]), there could have been adverse effects on the loss of CTs. Comparatively, the combination of more suitable environmental conditions for microbial assimilation and complexation might contribute to a greater loss of CTs under the closed canopy and in the expanded gap, which would be consistent with our results.
In addition, all six types of foliar litter exhibited continuously high losses of CTs (70.18% to 96.57%) throughout the study, with the conifers litters showing a greater CTs loss (92.87% to 96.57%) than the broadleaved trees litters (70.18% to 85.98%) (Tab. 1). In our study, only the methanol-extractable CTs fraction was studied, while that of the bound CTs was not analyzed. In this context, our results the the highly extractable CTs losses are consistent with those of previous studies that showed that the extractable phenolics and tannins decreased along with litter decomposition ([30]). The CTs losses in the conifers litter might largely depend on the mechanical disruptions caused by the freeze-thaw cycle and leaching, which would result in a greater loss of CTs from conifers litter during the winter. In addition, due to the higher amounts of easily leachable CTs remaining in conifers litter (as it was less decomposed in winter), the higher litter CTs loss might also might be depended on changes in litter quality during the decomposition process. However, the broadleaved trees litter CTs losses might largely depend on chemical modification by microbial assimilation that is influenced by temperature and moisture conditions, which would result in a greater loss of CTs from broadleaved trees litters CTs loss during the growing season. In addition to environmental factors, these variations may not only be caused by the significant differences in the initial substrate among the species, but also by changes in litter quality during decomposition ([4]).
Conclusions
This study describes the decomposition dynamics of methanol-extractable CTs in relation to forest gap and season in an alpine Minjiang fir forest. Over the first year of decomposition, all six types of foliar litter experienced high CTs losses, with values ranging from 70.18% to 96.67%. The gap center exhibited higher litter CTs losses over the whole year compared with the other gap positions. Forest gaps accelerated litter CTs losses in the winter but inhibited CTs losses in the growing season, which showed significant seasonal differences in litter CTs decomposition. Additionally, the litter of conifers showed greater CTs losses in winter, especially during the snow formation period, whereas the litter of broadleaved trees exhibited greater CTs losses in the growing season. Our results suggested that the formation of forest gaps would promote litter CTs losses in the winter, but retard CTs losses in the growing season in the alpine Minjiang fir forest. Furthermore, they also indicate that the predicted reduction in snow depth resulting from winter warming or the loss of forest gaps caused by forest regeneration will inhibit the decomposition of litter CTs in alpine forest ecosystems, thus slowing the soil carbon sequestration from foliar litter in cold biomes.
Acknowledgements
We are very grateful to Wei He for assistance with the laboratory analyses. This project was supported by the National Natural Science Foundation of China (Project no. 31170423 and 31270498), the National Key Technologies R and D Program (2011BAC09B05), the Sichuan Excellent Youth Science and Technology Foundation (2012JQ0008, 2012JQ0059) and the special Funding of the China Postdoctoral Science Foundation (2012T50782).
References
Gscholar
Gscholar
Gscholar
Authors’ Info
Authors’ Affiliation
Fuzhong Wu
Wanqin Yang
Liya Xu
Xiangyin Ni
Jie He
Bo Tan
Yi Hu
Meta Francis Justin
Long-term Research Station of Alpine Forest Ecosystems, Institute of Ecology and Forestry, Sichuan Agricultural University, Chengdu 611130 (China)
Wanqin Yang
Bo Tan
Collaborative Innovation Center of Ecological Security in the Upper Reaches of Yangtze River, Chengdu 611130 (China)
Corresponding author
Paper Info
Citation
Li H, Wu F, Yang W, Xu L, Ni X, He J, Tan B, Hu Y, Justin MF (2016). The losses of condensed tannins in six foliar litters vary with gap position and season in an alpine forest. iForest 9: 910-918. - doi: 10.3832/ifor1738-009
Academic Editor
Giustino Tonon
Paper history
Received: Jun 10, 2015
Accepted: May 02, 2016
First online: Aug 04, 2016
Publication Date: Dec 14, 2016
Publication Time: 3.13 months
Copyright Information
© SISEF - The Italian Society of Silviculture and Forest Ecology 2016
Open Access
This article is distributed under the terms of the Creative Commons Attribution-Non Commercial 4.0 International (https://creativecommons.org/licenses/by-nc/4.0/), which permits unrestricted use, distribution, and reproduction in any medium, provided you give appropriate credit to the original author(s) and the source, provide a link to the Creative Commons license, and indicate if changes were made.
Web Metrics
Breakdown by View Type
Article Usage
Total Article Views: 47347
(from publication date up to now)
Breakdown by View Type
HTML Page Views: 40654
Abstract Page Views: 2362
PDF Downloads: 3220
Citation/Reference Downloads: 55
XML Downloads: 1056
Web Metrics
Days since publication: 3254
Overall contacts: 47347
Avg. contacts per week: 101.85
Article Citations
Article citations are based on data periodically collected from the Clarivate Web of Science web site
(last update: Mar 2025)
Total number of cites (since 2016): 6
Average cites per year: 0.60
Publication Metrics
by Dimensions ©
Articles citing this article
List of the papers citing this article based on CrossRef Cited-by.
Related Contents
iForest Similar Articles
Research Articles
The manipulation of aboveground litter input affects soil CO2 efflux in a subtropical liquidambar forest in China
vol. 12, pp. 181-186 (online: 10 April 2019)
Research Articles
Soil fauna communities and microbial activities response to litter and soil properties under degraded and restored forests of Hyrcania
vol. 14, pp. 490-498 (online: 11 November 2021)
Research Articles
Soil respiration along an altitudinal gradient in a subalpine secondary forest in China
vol. 8, pp. 526-532 (online: 01 December 2014)
Research Articles
Effects of arbuscular mycorrhizal fungi on microbial activity and nutrient release are sensitive to acid deposition during litter decomposition in a subtropical Cinnamomum camphora forest
vol. 16, pp. 314-324 (online: 13 November 2023)
Research Articles
Effect of plant species on P cycle-related microorganisms associated with litter decomposition and P soil availability: implications for agroforestry management
vol. 9, pp. 294-302 (online: 05 October 2015)
Research Articles
Seasonal dynamics of soil respiration and nitrification in three subtropical plantations in southern China
vol. 9, pp. 813-821 (online: 29 May 2016)
Research Articles
Retranslocation of foliar nutrients of deciduous tree seedlings in different soil condition under free-air O3 enrichment
vol. 9, pp. 835-841 (online: 17 June 2016)
Research Articles
Effects of stand age on litter quality, decomposition rate and nutrient release of Kazdagi fir (Abies nordmanniana subsp. equi-trojani)
vol. 13, pp. 396-403 (online: 03 September 2020)
Research Articles
No home-field advantage in upper Andean tropical forests despite strong differences in site environmental characteristics
vol. 17, pp. 286-294 (online: 27 September 2024)
Research Articles
Mid-rotation fertilization and liming of Pinus taeda: growth, litter, fine root mass, and elemental composition
vol. 14, pp. 195-202 (online: 24 April 2021)
iForest Database Search
Search By Author
Search By Keyword
Google Scholar Search
Citing Articles
Search By Author
Search By Keywords
PubMed Search
Search By Author
Search By Keyword