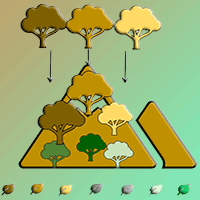
No home-field advantage in upper Andean tropical forests despite strong differences in site environmental characteristics
iForest - Biogeosciences and Forestry, Volume 17, Issue 5, Pages 286-294 (2024)
doi: https://doi.org/10.3832/ifor4518-017
Published: Sep 27, 2024 - Copyright © 2024 SISEF
Research Articles
Abstract
Litter decomposition is not fully explained by the general triangle of climate, litter quality and soil decomposers. Therefore, other theoretical frameworks, such as Home-Field Advantage (HFA), have emerged to explain the remaining variation of decomposition. HFA states that litter decomposes faster in their site of origin (home) than far from it (away). However, there are no consistent patterns of HFA and this can varies depending the ecosystem and plant species analyzed. One of the most variable ecosystems in terms of species biodiversity turnover, topography, and soil conditions are the Upper Andean Tropical Forests (UATF), but to date there is no study testing HFA in this ecosystem. Here, HFA was tested through a reciprocal litterbag translocation field experiment across different UATF. The experiment comprised 2520 litterbags placed in 14 20 × 20 m plots that belonged to four sites to analyze decomposition of 15 plant species for 18 months. Of these 15 species, seven were present at only one site. The mean decomposition was calculated for all 15 species to determine the relative decomposition at each site and the decomposition of the seven species at home and away sites was analyzed through two-way ANOVA (sites × species) and linear mixed models. I contrasted environmental charcteristics between sites including litter depth, slope, leaf area index, canopy openness, and microclimatic variables. The results showed that the pattern of decomposition was always the same, no matter the origin of the species and the decomposition period. Microclimate, litter depth, and slope varied between sites, yet these differences were not enough to influence affinity effects of decomposition, as relative decay rates were similar between home and away sites. Overall, no HFA was found in UATF possibly because: (i) strong environmental filters along montane forests homogenize decomposer communities; (ii) high diversity in litters drive decomposers with high ability to degrade different organic compounds; (iii) little adaptation of decomposers to recurrent litter as they respond mainly to changes in litter quality. These results imply that changes in species composition by current anthropogenic pressures could have profound impacts on carbon cycle and nutrient fluxes depending on the identity of species arriving in UATF.
Keywords
Carbon Cycling, Colombian Andes, Litter Decomposition, Litter Affinity Effects, Montane Forests, Nutrient Cycles, Reciprocal Litter Transplant, Plant-soil Feedbacks
Introduction
Litter decomposition plays a crucial role in the carbon cycling and nutrient release in terrestrial ecosystems ([40], [5]). While several studies have established a general framework in which climate and litter quality drive the influence of decomposers on litter breakdown ([7]), these factors do not entirely account for the variation of this carbon flux. Other factors, including the interactions between the plants and the environmental variables at a specific site, exhibit a high degree of specificity, leading to an increase in decomposition rates ([6], [48]). This phenomenon, often referred to as the “Home-Field Advantage” (henceforth HFA), suggests that litter decomposes more rapidly when located close to its plant origin (home) compared to a distant location (away) ([50], [1]). One of the primary mechanisms proposed to explain this affinity between litter and its site of origin is the specialization of microbial communities that progressively adapt to degrade similar litter quality, which accumulates within microsites over time ([4], [1]). Some studies provide clear evidence showing that plant species create specific conditions over the long-term, leading to increase decomposition in their own litter, thus establishing a stronger affinity between the litter species and their own microenvironment during the advanced stages of decay ([50]). Nonetheless, multiple factors, including interactive effects between soil fauna, pathogens, phyllosphere, ectomycorrhizal associations, trophic cascades, among others, may influence decomposition rates within home sites ([1], [16]).
Different factors have been associated with HFA. For example, the quality of litter has been identified as a main factor contributing to the differences between home and away sites. Such differences are more pronounced in low-quality litter, primarily because recalcitrant species require specific decomposers capable of producing enzymes to break down complex organic compounds ([41]). In contrast, high-quality litter contains labile compounds that are easily degradable by soil biota in most soil communities, which can rapidly degrade these compounds ([3], [4]). Moreover, the composition and diversity of above-ground plant species play a fundamental role in HFA. This is because all litter materials and substrates that interact with soil decomposers are determined by the litter production and quality originating from the above-ground plant community. In cases where forests are dominated by a single species, the recurring litter consists of a specific substrate that promotes local adaptation of the soil communities to the decomposition of litter produced by the same plant species, thereby benefiting these communities by providing faster access to the energy and nutrients within the litter ([50], [4]). Conversely, in forests with multiple plant species, the recurring litter is diverse, characterized by a variety of chemical and physical traits. This diversity encourages the presence of decomposers with a wide-ranging capacity to break down the different types of litter they come into contact with ([18], [26]). In addition, significant dissimilarity in environmental conditions between home and away sites can lead to greater HFA due to the local adaptation of soil communities to microsite conditions ([48], [35]). Specifically, variations in microclimatic factors, such as soil temperature and moisture, as well as differences in forest attributes related to canopy cover, leaf area, slope, among others, may impose filters on decomposers, which in turn affect the decomposition process ([42], [39]).
Over the past few decades, several studies have examined HFA, revealing contrasting results; while some studies support this hypothesis ([50], [3], [4]) others have found no HFA ([43], [46]) or presented inconclusive results ([44], [37]). This variety of outcomes can partially be attributed to the specific habitat conditions, including climatic zones and litter types present in each context ([47]). Furthermore, HFA can also change over time due to the shifts of environmental conditions that affect soil biota in home and away sites ([44]). For instance, it has been found that HFA is more pronounced at early stages of decay due to the specialization of decomposers to labile and high-quality compounds, which are abundant at the beginning of the decomposition process in temperate deciduous forests ([23]), but also the converse pattern have been observed in mixed old-growth forests in temperate South America due the long-term effect of native plant species in the creation of specific conditions that improve decay rates of their own litter ([50]). Additionally, not all plant species conform to the principles of HFA ([46]). Most of the studies investigating HFA in the context of litter decomposition have focused on temperate and tropical lowland ecosystems ([4], [47], [27], [35]). However, tropical upper montane forests have remained understudied, despite their recent recognition as strategic ecosystems for future global carbon storage and climate mitigation ([9], [14]).
One of the most representative ecosystems found in tropical uplands are the Andean mountain forests of Northern South America. These forests, situated between 2600-3200 meters elevation, are known as the Upper Andean Tropical Forests (henceforth UATF), and they stand out for their exceptional biodiversity of plants and animals, many of which are exclusive to this particular region of the planet ([31], [32], [12]). Notably, edaphic and topographical features in this area exhibit dramatic changes over short distances ([30], [38]), and the plant communities are characterized by a high degree of beta-diversity ([20], [8]), showing distinct conditions from one forest to another. This diversity in conditions provides an ideal setting for testing the HFA, which can help to understand factors that largely explain litter decomposition. Importantly, in ecosystems with sluggish metabolism such as UATF ([9], [11]), the impacts of both above- and below-ground factors on decomposition require long-term experimental studies that adequately evaluate the effects of HFA over time, as has been demonstrated in the natural Andean forests of the region ([50]).
In this study, I tested HFA through a fully reciprocal litterbag translocation field experiment that comprised 15 native plant species across different UATF. Seven of the species were only found in one of the sites studied, facilitating a direct comparison of litter decomposition between their home and away sites. For these particular seven species, I hypothesized that: (i) litter decomposition in home sites will be higher than away sites because of the distinctive floristic composition found in each Andean mountain forests, facilitating the establishment of specialized relationships between decomposers and the substrates; (ii) these affinity effects will be reflected in site variations between home and away sites at both forest attributes and microclimatic conditions; and (iii) differences in decomposition between home and away sites will become more pronounced during the later stages of decay, due to the long-term effect of plant species at home sites creating specific conditions that boost decay rates on their own litter, thereby establishing a strong affinity between single-species litter and their respective home microenvironments.
Materials and methods
Study area
This study was conducted in the Eastern Andes of Colombia, specifically in the Cundiboyacense High Plateau (commonly known as Sabana de Bogotá). In this region, dominant activities in the landscape matrix include agriculture, cattle pastures, urbanization, and mining ([15]). The landscape is comprised of remnants of forest patches at different stages of succession ([8], [10]). The high plains experience two distinct rainy periods throughout the year, occurring from April to June and again from October to December ([22]).
In this region, 14 permanent plots (20 × 20 m) were established as part of the “Rastrojos project” (a larger network consisting of thirty-six 20 × 20 m plots and eight 50 × 50 m plots), which were originally set up in 2013 within the UATF ([20], [10]). These set of 14 plots are placed in four different sites, situated at elevations ranging from 2685 to 3140 m a.s.l. (Fig. 1). Three of the sites are located in municipalities within the Sabana de Bogotá region (i.e., Guasca, Guatavita, Tabio - Fig. 1), and one site is placed in the eastern hills of Bogotá, the capital city of Colombia (i.e., Torca - Fig. 1). The sites encompass secondary and old-growth forests, each exhibiting unique forest structural attributes and environmental conditions ([10]). Within the 14 plots, five dominant plant families were identified: Ericaceae, Melastomataceae, Cunoniaceae, Primulaceae, and Asteraceae, collectively representing 56% of all individuals with a basal diameter above 5 cm. A total of 63 species of shrubs and trees composes the 14 studied plots ([10]).
Fig. 1 - Study area in the Andean mountains of Colombia. In grey circles the plots studied. Sites are represented by different colors in the stars (Guatavita in yellow, Guasca in blue, Tabio in red, and Torca in green).
Experimental set-up
A fully reciprocal translocation field experiment with multiple species was perfomed between October 2021 and April 2023. The experiment was conducted in the 14 permanent plots, each comprising three litter decomposition beds. Within these beds, there were 15 different litter species, each with four decomposition times (Castillo-Figueroa et al., in review). This arrangement led to a total of 2520 litterbags (14 plots × 3 litterbeds × 15 species × 4 harvesting times - see below). Within these species, seven were exclusive to specific sites (3 species in Torca, 3 species in Tabio, and 1 species in Guasca). This design allowed to separate site-specific effects from litter quality effects of the other species on litter decomposition ([3]).
Besides these three sites, Guatavita was included as an additional away site for all the evaluated species. The seven species were: Alnus acuminata Kunth from Guasca, Croton bogotanus Cuatrec., Daphnopsis caracasana Meisn., and Cedrela montana Moritz ex Turcz. from Tabio, and Clusia multiflora Kunth, Prunus buxifolia Koehne, and Ocotea calophylla Mez from Torca. Within each plot, three independent litterbeds per plot were prepared, totaling 42 litterbeds across the study. These experimental units were positioned directly on the forest floor, aiming to minimize soil disturbance and maintaining a minimum distance of 5 m between them. During placement, forest gaps, topographic depressions, and highly irregular soil conditions were avoided. For the seven species, each litterbed consisted of 28 litterbags of fiber glass (10 × 15 cm) with a mesh size of 2 mm, allowing the entrance of micro- and mesofauna. Each litterbag contained 2 g of initial dry mass. Over the course of 1.5 years, litterbags were collected at four distinct incubation periods (3, 6, 12, and 18 months), culminating in a total of 1176 litterbags (14 plots × 3 litterbeds per plot × 7 species per litterbed × 4 harvesting times). In the laboratory, the collected litterbags underwent a gentle brushing to eliminate mineral soil particles. All other contents of the litterbags were discarded, except for the soil fauna, which were carefully collected and preserved in 70% ethanol (Castillo-Figueroa et al., unpublished). Subsequently, the litter material was oven-dried at 60 °C for 72 hours and weighed using a precision scale accurate to 0.1 mg (LX 220A scs).
Environmental variables
In the center of each of the 14 plots, TMS-4 soil probes equipped with dataloggers (TOMST, Czech Republic) were installed to record microclimatic variables including soil volumetric water content and temperature at 15-minute intervals. This device features three distinct temperature sensors positioned at three crucial points: 15 cm above the soil surface, at the soil surface itself, and at a depth of 8 cm within the soil. Notably, at the bottom bears a probe that effectively measures volumetric water content at 14 cm beneath the surface ([54]). Data of mean soil temperature, mean surface temperature, mean atmospheric temperature, and mean soil volumetric water content was obtained from February 2022 to April 2023.
For each litterbed, forest measurements of canopy openness and leaf area index were obtained by taking hemispherical photographs (Canon Inc. EW-77 Fisheye Zoom Lens EF 8-15mm 1:4, Japan), which were processed employing the software Gap Light Analyzer (GLA version 2.0, ⇒ https://www.caryinstitute.org/). Slope was measured with a clinometer (Angle Meter app) and elevation was obtained with a sub-metric GPS (Spectra precision, MobileMapper 100). Finally, the depth of leaf-litter was averaged from five random soil points in each litter decomposition bed using an electronic digital caliper (0.1 mm).
Data analysis
Decomposition for each of the seven species was determined as the mass remaining at each of the harvesting times (i.e., the percentage of initial dry mass remaining). Mass loss (%) and decay rates (K rate y-1) were also estimated according to Olson ([36]) and Njoroge et al. ([33]). A two-way ANOVA was conducted to assess any difference between the species at home versus the away sites, with litter species and sites as the main factors, along with their interaction. A significant interaction would suggest the potential occurrence of HFA. This was tested by each time period to determine if there were changes in the HFA over time. Litter decomposition at each site was also standardized by calculating the relative decay rates (K rate relative year-1) as the observed k rate of the home species divided by the mean k rate of the 15 litter species in a given site ([29]). Thus, through one-way ANOVA and linear mixed models, the effect of plant litter origin between incubation sites was tested using K rate relative year-1. In the linear mixed models, litterbeds nested within plots and litter species were employed as random factors, while site was treated as a fixed factor. This approach aimed to develop a more general model of HFA. Regarding the environmental conditions of the sites, a one-way ANOVA was used to determine whether both forest and microclimatic variables varied between sites. Significant differences were considered using a α level of 0.05 for all the statistical analyses. Size effects in ANOVA tests were calculated using eta squared (η²). All the analyses were conducted in JASP v. 0.17.2.1 ([24]).
Results
Litter decomposition along time and HFA
Overall, the examined species displayed different decomposition rates, with the sequence from high to low being as follows: C. bogotanus > D. caracasana > A. acuminata > C. montana > P. buxifolia > C. multiflora > O. calophylla (Fig. 2). Over the course of the study, the average decomposition of the seven species increased with time, ranging from 18.43 ± 11.48% at 3 months to 40.78 ± 19.01% at 18 months. At the 3-month, 6-month, 12-month, and 18-month intervals, significant differences in litter decomposition were observed between the species (3 months: p <0.001, DF = 6, F = 371.969, η² = 0.801; 6 months: p <0.001, DF = 6, F = 308.002, η² = 0.790; 12 months: p <0.001, DF = 6, F = 216.570, η² = 0.745; 18 months: p <0.001, DF = 6, F = 151.303, η² = 0.687 - Fig. 3). Furthermore, significant differences were also observed between the study sites at these time intervals (3 months: p <0.001, DF = 3, F = 13.934, η² = 0.015; 6 months: p = 0.001, DF = 3, F = 5.517, η² = 0.007; 12 months: p <0.001, DF = 3, F = 7.261, η² = 0.012; 18 months: p <0.001, DF = 3, F = 9.247, η² = 0.021 - Fig. 3). It is noteworthy that Tabio consistently exhibited the highest mean decomposition rates for all the seven studied species (Tab. 1), whereas the lowest values varied between Guasca, Guatavita, and Torca depending on the species and stage of decay (Fig. 3). Importantly, the interaction between the study sites and litter species was only significant at the 3-month interval (3 months: p = 0.03, DF = 18, F = 1.726, η² = 0.011), but for the subsequent stages of decay, namely 6 months (6 months: p = 0.141, DF = 18, F = 1.380, η² = 0.011), 12 months (12 months: p = 0.479, DF = 18, F = 0.984, η² = 0.010), and 18 months (18 months: p = 0.514, DF = 18, F = 0.954, η² = 0.013), no significant interaction effect was observed (Fig. 3). However, it is worth noting that there were no significant differences between the home sites and away sites in terms of relative decay rates (p>0.05 - Fig. 4). This finding was further confirmed by the results of the linear mixed models, which indicated that sites had no effect on relative decomposition at any stage of decay (Tab. 2).
Fig. 2 - Average mass remaining (%) of the seven species studied along time. Each color depicts a different species as follows: Ocotea calophylla in yellow, Clusia multiflora in green, Prunus buxifolia in dark blue, Cedrela montana in dark golden, Alnus acuminata in light blue, Daphnopsis caracasana in orange, Croton bogotanus in grey.
Fig. 3 - Two-way ANOVA between seven species and four sites at (a) 3 months, (b) 6 months, (c) 12 months, and (d) 18 months. House symbol represents the home site for each species. Each symbol depicts a different species as follows: Ocotea calophylla in filled diamonds, Clusia multiflora in open squares, Prunus buxifolia in open triangles, Cedrela montana in filled circles, Alnus acuminata in open circles, Daphnopsis caracasana in open diamonds, Croton bogotanus in filled squares.
Tab. 1 - Mean ± standard deviation of mass loss (%) of the seven species at every stage of decay per site.
Site | 3 months | 6 months | 12 months | 18 months |
---|---|---|---|---|
Guasca | 16.68 ± 10.89 | 24.01 ± 13.33 | 33.99 ± 16.60 | 38.93 ± 17.06 |
Guatavita | 17.14 ± 11.28 | 22.19 ± 13.62 | 32.24 ± 15.99 | 38.47 ± 16.39 |
Tabio | 19.44 ± 12.32 | 25.70 ± 14.89 | 36.62 ± 20.11 | 45.10 ± 23.05 |
Torca | 19.84 ± 11.18 | 25.20 ± 13.90 | 31.83 ± 15.42 | 39.46 ± 17.14 |
Total | 18.43 ± 11.48 | 24.57 ± 13.97 | 33.87 ± 17.31 | 40.78 ± 19.01 |
Fig. 4 - Raincloud plots showing the relative decomposition rate of home species (to the mean value of all 15 litter species in a given site) in (a) Guasca, (b) Tabio and (c) Torca. House symbol represents the origin of litter species analyzed in each site.
Tab. 2 - Linear mixed models including absolute and relative decay rate (K rate year-1) as dependent variables. Litterbeds nested within plots, and litter species as random factors, while site was considered as fixed factor.
Fixed effect | K rate year-1 | Time | df | F | p-value |
---|---|---|---|---|---|
Site | Absolute decay | 3 months | 3, 7.83 | 1.932 | 0.205 |
6 months | 3, 8.79 | 0.848 | 0.502 | ||
12 months | 3, 10.38 | 0.968 | 0.444 | ||
18 months | 3, 10.12 | 0.522 | 0.677 | ||
Relative decay | 3 months | 3, 7.92 | 0.102 | 0.957 | |
6 months | 3, 6.23 | 0.065 | 0.976 | ||
12 months | 3, 8.65 | 0.083 | 0.968 | ||
18 months | 3, 10.37 | 0.031 | 0.992 |
Variations between sites
Microclimatic conditions exhibited notable variations between sites, particularly in terms of soil temperature (p <0.001, DF = 3, F = 332.142, η² = 0.829), surface temperature (p <0.001, DF = 3, F = 273.403, η² = 0.799), air temperature (p <0.001, DF = 3, F = 264.241, η² =0.794), and soil volumetric water content (p<0.001, DF = 3, F = 8.28, η² = 0.114), with Tabio showing higher temperatures and soil moisture levels (Fig. 5a-d). Forest attributes also exhibited variations between sites, particularly regarding litter depth (p < 0.01; DF = 3; F = 23.853, η² = 0.653) and slope (p = 0.04; DF = 3; F = 2.912, η² = 0.187). However, no significant differences were observed in terms of canopy openness (p = 0.849; DF = 3; F = 0.267, η² = 0.021) and leaf area index (p = 0.814; DF = 3; F = 0.315, η² = 0.024 - Fig. 5e-h).
Fig. 5 - Comparisons of environmental variables from both microclimate and forest characteristics between sites with 95% confidence intervals. For microclimate variables: (a) soil temperature; (b) surface temperature; (c) air temperature; (d) soil volumetric water content. For forest attributes: (e) canopy openness; (f) leaf area index; (g) depth of litter; (h) slope.
Discussion
The findings of this study do not support the hypothesis of HFA in UATF. Additionally, the comparisons of forest attributes and microclimatic conditions between sites suggest that these environmental conditions do not exert a major control over litter decomposition despite of their variation. Instead, other factors related to litter quality and species identity may play a more significant role in driving this process within UATF. The consistent pattern observed in the ranking of species by decay rate across all sites suggests that decomposers do not exhibit specialization towards specific recurrent litter materials from their site of origin. Alternatively, they seem to preferentially target litter with high quality, regardless of the site or decomposition period (Castillo-Figueroa et al., in review). Overall, this study unveils the lack of affinity effects between microsite conditions and litter species from their site of origin, even though variations in some characteristics of topography, temperature, soil volumetric water content, and litter layer were found across sites.
Litter effects on decomposition and HFA
Contrary to the first hypothesis, no HFA was identified for any of the analyzed species (Fig. 3). Despite UATF show a high singularity in plant biodiversity from place to place ([20], [8]), as well as exhibit a diverse range of topographical features ([38]), and edaphic conditions ([30]), such diversity does not manifest in distinct patterns of decomposer adaptation to the litter matrix at the study sites. This could be attributed by three potential explanations: (i) indirect effects caused by the narrow climatic gradient studied that may influence the composition and functional redundancy of microbial decomposers; (ii) the high beta-diversity and heterogeneity in litter production and quality in UATF may result in a diverse decomposer community with a high ability to degrade a wide range of organic compounds and litter types; and (iii) limited decomposers adaptation to abundant litter, as they respond mainly to changes in litter quality.
Andean montane forests exhibit similar environmental characteristics such as low temperatures ([39]), low soil pH ([52]), high soil organic carbon ([52]), low nitrogen concentration in soils ([53]) and high-water retention ([17]). Although microbial diversity generally decreases with higher elevations ([34]), these microorganisms show notable variations between functional groups; for instance, the diversity of free-living saprotrophic fungi and arbuscular mycorrhizas experiences marked declines in upper mountain regions, in contrast to ectomycorrhizal fungal diversity, which consistently increases with elevation ([28]). The narrow environmental gradient along the upper mountain forests, especially in terms of climate could influence the functional redundancy of microbial communities related to decomposition. Indeed, growing evidence suggests that climate is a primary driver of soil fungal diversity ([28], [41]). Given that the sites studied presented a minor variation in abiotic factors such as mean atmospheric temperature (ranging from 9.37 oC to 11.91 oC) and soil volumetric water content (ranging from 33.5% to 41.6 %), it is likely that the similar climatic conditions exert a large influence on soil microbial decomposer homogenization. This, in turn, could lead to a notable functional redundancy in decomposition, thus weakening the effect of HFA.
Alternatively, the high diversity of plant species composition ([20], [8]) as well as the variation in litter production rates between different UATF (Castillo-Figueroa & Posada, in review), could create different litter-substrate matrices on the soil where decomposers can interact with. The diversity of substrates may result in a limited decomposer specialization due to their high ability to degrade a wide range of litter types and organic compounds ([18], [26], [35]). This functional flexibility can be attributed to the capacity of bacteria and fungi to dynamically adjust their community structure over short timescales through rapid population growth or the expansion of hyphae during the decomposition of different substrates ([18]). Nonetheless, further research is required to delve deeper into the decomposer community and gain a better understanding of whether specialized decomposers are absent or play a limited role in litter breakdown in UATF. Additionally, it is essential to assess the soil community’s ability to efficiently degrade a diverse array of organic substrates.
Consistent with this idea, decomposers exhibited little adaptation to individual litter of their respective home environments. Given that in UATF the plant communities are characterized by leaf traits associated with low litter quality ([19]), and that there is a high biodiversity turnover between forests with substantial shifts in plant dominance ([20]), one would expect a higher degree of specialization in decomposers across different litter types from place to place. Such specialization could potentially result in a reduced ability of the decomposer community to break down foreign litter material ([18]). However, I found highly consistent effects on litter identity no matter the site, which indicates that microbial decomposers may select litters with higher quality from the environment. This phenomenon could be a consequence of nitrogen limitation and low soil fertility in UATF ([49], [45], [53]), which lead decomposers to preferentially break down litter material with elevated nutrient concentrations regardless of whether they originate from native or exotic plant species. The implications of these results highlight the fact that changes in litter input due to the arrival of foreign species whatever the reason (e.g., thermophilisation, land-use management, invasive species), have the potential to induce dramatic changes in litter decomposition dynamics.
Likewise, HFA has often been found in forests dominated by a single species ([50], [4], [25]) rather than multiple plant species ([46], [35]) as in this study. Considering that no species was monodominant in the plots, and instead, they comprised diverse plant communities (see [20]), litter matrix in the soil should consist of different shapes, forms, and chemical compositions that preclude for decomposer specialization, as mentioned above. Forests dominated by a single or few species with low litter quality tend to show a high specialization in the decomposers at home sites, favoring HFA ([25]). It is likely that the same may occur in Andean forests dominated by oak species that produce recalcitrant litter such as Quercus humboldtii and Colombobalanus excelsa as some studies have found HFA in species of this family ([23], [25]). Future studies should explore HFA in these oak forests since they also extend across the three mountain ranges of the Colombian Andes ([2]).
Site variations
Contrary to the second hypothesis, although the results of this study show some differences in forest structure between sites in UATF (Fig. 5), these variations were not enough to influence affinity effects between litter substrate and associated decomposers as relative decay rates were similar between home and away sites (Fig. 4). Some studies have found that microsite variations resulting from plants associated to leaf litter detritus play a central role in shaping belowground decomposition fluxes ([50], [1]). Although differences in litter depth between forests were observed (Fig. 5), these variations did not appear to be directly linked to HFA. This might be attributed to the fact that, as previously mentioned, what primarily governs affinity effects is not only the quantity of litter in the soil matrix but also its quality. The variation in slope between sites was also evident, yet it did not exhibit a discernible influence on HFA. Some studies have suggested that topographic variation can influence leaching and nutrient accumulation, thereby affecting decomposition rates. For instance, flatter microsites, such as valleys, tend to accumulate nutrients to a greater extent, fostering decomposition. Conversely, areas with steeper slopes often experience more nutrient loss, resulting in reduced decomposition rates ([51], [42], [13]). However, in the context of this study, slope variation did not appear to be a critical factor driving affinity effects between litter and decomposers within the study plots.
Recent studies have found that high dissimilarity between home and away sites in key forest attributes such as soil nutrient concentration, as well as litter nutrient limitation can increase HFA due to decoupled relationships between litter characteristics and decomposers ([48], [35]). In this sense, it is probable that factors more directly related to decomposers, such as soil chemistry, could provide an explanation for the absence of HFA in the study plots. Moreover, HFA occurs generally over small spatial scales within sites close to the home plant, instead of between sites, as was the approach of this study. Hence, litter from a single species can, to some extent, resemble a “monoculture” underneath an individual tree, creating a small-scale mosaic of few litter types with the microbial decomposer communities specialized for each type of litter and particular microenvironmental conditions. When viewed from this small-scale perspective, the conditions are comparable to forests with a limited number of tree species, where HFA effects are expected to be prevalent ([18]). Thus, microenvironmental conditions linked to litter decomposition and affinity effects such as temperature, soil moisture, and soil chemistry should be analyzed at small-scales beneath the home plant to better understand the factors that control HFA.
The fact that no HFA was found in this study highlights the context-dependency of this phenomenon, suggesting that it may not be applicable to every ecosystem or species ([25], [47], [35], [41]). As pointed out by Veen et al. ([47]), HFA becomes more generalizable when different functional groups and ecosystems are contrasted. Besides the narrow environmental gradient, the high ability of decomposers to degrade a variety of organic compounds, and the limited litter adaptation of decomposers to recurrent litter, another potential reason for the absence of HFA in my findings could be the similarity between the ecosystems and functional groups under comparison. That is, all sites encompassed Andean forests from the same slope of the Eastern mountain range, and all the seven species analyzed were trees. However, further research should encompass broader environmental gradients, including different functional groups and mountain ranges within the Andean region, to test whether HFA depends on the dissimilarity of species, functional groups, and sites. This study comprises an important step toward the understanding of litter affinity effects and HFA in a strategic region characterized by high soil carbon pools and plant biodiversity.
Time effects on decomposition
Contrary to the third hypothesis, despite an increase in litter decomposition over time, no differences were observed between home and away sites (Fig. 3). This suggests that there may not be a specialization of decomposers at specific stages of decomposition, as it has been observed in previous studies ([50], [44]). While the UATF experiences a bimodal annual precipitation pattern ([22]), it is plausible that variations in environmental conditions over time are more pronounced in ecosystems with significant seasonal changes in temperature, such as temperate forests ([44]). Furthermore, this lack of differentiation could also be attributed to interannual climatic variations (e.g., ENSO, La Niña). Notably, the litter experiment extended across a substantial portion of the exceptionally rainy year in 2022 ([21]), potentially leading to a homogenization of environmental conditions for decomposers throughout the course of the experiment.
Conclusions
The take-home message of this study is that UATF in the Eastern Andes of Colombia do not exhibit HFA, which rejects my main hypothesis. Instead, decomposition processes are primarily governed by litter identity, as decomposers exhibit substantial responses to litter quality rather than a strong adaptation to the recurring litter from their own origins. The limited nutrient availability in Andean mountain soils may contribute to making high-quality litter inputs more easily consumable and preferred by decomposers, regardless of their origin. Furthermore, the substantial variation in litter quality and production within forests comprising multiple plant species could constrain decomposer specialization, owing to their capacity to efficiently decompose a broad spectrum of litter types and organic compounds. Although variations in forest attributes were observed between sites such as litter depth, slope, and microclimatic conditions (e.g., soil temperature and moisture), these differences did not manifest in shifts in affinity effects on litter decomposition. This suggest that forest and microclimate attributes other than the analyzed here may explain the lack of HFA (e.g., soil chemistry) and/or that the primary driver of decomposition is litter quality itself. This implies that changes in species composition resulting from ongoing environmental shifts or anthropogenic pressures, such as invasive species, thermophilization, and landscape transformation, could significantly influence the carbon cycle and nutrient fluxes over these ecosystems. The magnitude of these impacts would depend on the functional traits of the specific species introduced to the ecosystem. While this study represents the first attempt to test HFA in UATF, further research is necessary to gain a comprehensive understanding of the context-dependent nature of HFA. This should include litter translocation experiments at small spatial scales within the sites, broader environmental gradients encompassing a wider array of species, functional groups, and different ecosystems composed of multiple species, but also dominated by single species such as native oak forests. Only through such endeavors can we gain a more complete understanding of how HFA operates in the tropical Andean mountains.
List of abbreviations
HFA: Home Field Advantage; UATF: Upper Andean Tropical Forest
Data availability statement
The data that support the findings of this study are openly available in Open Science Framework repository at ⇒ https://osf.io/fkuds/.
Acknowledgments
I want to express my gratitude to the owners of the private areas where I conducted this research for their hospitality and logistical assistance. Financial support for this study was provided by Universidad del Rosario under the Small grant project “Dinámicas de regeneración y descomposición de hojarasca en un gradiente sucesional de bosque Altoandino” (Project ID: IV-FPD003). I am thankful to Juan M. Posada and Adriana Corrales for their invaluable discussions regarding litter decomposition and functional ecology in upper Andean tropical forests. I also want to thank to Laura Garzón-Salamanca for her help in the preparation of the map in Fig. 1, and to Brayan Polania-Camacho for his dedicated collaboration during fieldwork and in the laboratory. Lastly, I would like to express my gratitude to the two reviewers for insightful comments, which substantially improved the quality of this paper.
References
Gscholar
Gscholar
CrossRef | Gscholar
CrossRef | Gscholar
CrossRef | Gscholar
Gscholar
Gscholar
Authors’ Info
Authors’ Affiliation
Biology Department, Faculty of Natural Sciences, Universidad Del Rosario, Carrera 24 No. 63C-69. Bogotá, 111321 (Colombia)
Corresponding author
Paper Info
Citation
Castillo-Figueroa D (2024). No home-field advantage in upper Andean tropical forests despite strong differences in site environmental characteristics. iForest 17: 286-294. - doi: 10.3832/ifor4518-017
Academic Editor
Michele Carbognani
Paper history
Received: Nov 05, 2023
Accepted: Jun 28, 2024
First online: Sep 27, 2024
Publication Date: Oct 31, 2024
Publication Time: 3.03 months
Copyright Information
© SISEF - The Italian Society of Silviculture and Forest Ecology 2024
Open Access
This article is distributed under the terms of the Creative Commons Attribution-Non Commercial 4.0 International (https://creativecommons.org/licenses/by-nc/4.0/), which permits unrestricted use, distribution, and reproduction in any medium, provided you give appropriate credit to the original author(s) and the source, provide a link to the Creative Commons license, and indicate if changes were made.
Web Metrics
Breakdown by View Type
Article Usage
Total Article Views: 6113
(from publication date up to now)
Breakdown by View Type
HTML Page Views: 3906
Abstract Page Views: 924
PDF Downloads: 1063
Citation/Reference Downloads: 0
XML Downloads: 220
Web Metrics
Days since publication: 288
Overall contacts: 6113
Avg. contacts per week: 148.58
Article Citations
Article citations are based on data periodically collected from the Clarivate Web of Science web site
(last update: Mar 2025)
Total number of cites (since 2024): 1
Average cites per year: 0.50
Publication Metrics
by Dimensions ©
Articles citing this article
List of the papers citing this article based on CrossRef Cited-by.
Related Contents
iForest Similar Articles
Research Articles
Effects of stand age on litter quality, decomposition rate and nutrient release of Kazdagi fir (Abies nordmanniana subsp. equi-trojani)
vol. 13, pp. 396-403 (online: 03 September 2020)
Research Articles
Effects of arbuscular mycorrhizal fungi on microbial activity and nutrient release are sensitive to acid deposition during litter decomposition in a subtropical Cinnamomum camphora forest
vol. 16, pp. 314-324 (online: 13 November 2023)
Research Articles
The manipulation of aboveground litter input affects soil CO2 efflux in a subtropical liquidambar forest in China
vol. 12, pp. 181-186 (online: 10 April 2019)
Research Articles
Effect of plant species on P cycle-related microorganisms associated with litter decomposition and P soil availability: implications for agroforestry management
vol. 9, pp. 294-302 (online: 05 October 2015)
Review Papers
Recent insights in soil nutrient cycling: perspectives from Pinus and Eucalyptus forest studies around the world
vol. 17, pp. 394-404 (online: 20 December 2024)
Research Articles
Soil fauna communities and microbial activities response to litter and soil properties under degraded and restored forests of Hyrcania
vol. 14, pp. 490-498 (online: 11 November 2021)
Research Articles
Mid-rotation fertilization and liming of Pinus taeda: growth, litter, fine root mass, and elemental composition
vol. 14, pp. 195-202 (online: 24 April 2021)
Research Articles
Litter quality changes during decomposition investigated by thermal analysis
vol. 8, pp. 827-837 (online: 19 March 2015)
Research Articles
Soil respiration along an altitudinal gradient in a subalpine secondary forest in China
vol. 8, pp. 526-532 (online: 01 December 2014)
Research Articles
Changes in organic compounds during leaf litter leaching: laboratory experiment on eight plant species of the Sudano-guinea Savannas of Ngaoundere, Cameroon
vol. 1, pp. 27-33 (online: 28 February 2008)
iForest Database Search
Google Scholar Search
Citing Articles
Search By Author
Search By Keywords
PubMed Search
Search By Author
Search By Keyword