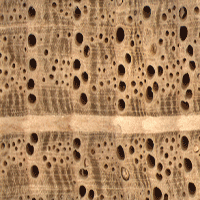
Behavior of pubescent oak (Quercus pubescens Willd.) wood to different thermal treatments
iForest - Biogeosciences and Forestry, Volume 8, Issue 6, Pages 748-755 (2015)
doi: https://doi.org/10.3832/ifor1348-007
Published: Feb 16, 2015 - Copyright © 2015 SISEF
Research Articles
Abstract
Quercus pubescens Willd. is a common oak species in the Mediterranean area, where it is currently exploited mainly for purposes such as firewood. To improve the technological properties of its wood, various steaming and/or heat treatments were applied to 160 wood samples from a southern Italian stand, cut from either sapwood or heartwood, while 20 samples served as controls. Steaming was carried out in an autoclave at 120 °C, whereas heat treatments were performed in an oven at 150 or 200 °C for 3 or 6 h. The equilibrium moisture content, swelling, mass loss, wood density, compression strength, color variation, and lignin content of the samples were measured and compared among treatments. The swelling and water adsorption of wood samples decreased due to the hydrothermal treatments. The mass loss was strictly related to the temperature and duration of the heat treatments, whereas it was not influenced by the steaming treatment. The average axial compression strength value was positively influenced by the combination of steaming and heat treatments. A significant and general darkening of color was also observed for the harshest treatments, while an increase of lignin content was detected mainly in the sapwood. Thus, pubescent oak wood subjected to steaming and heat treatments may acquire useful characteristics suitable for its industrial use.
Keywords
Steaming, Moisture Content, Swelling, Color, Strength, Ray Cells
Introduction
Pubescent or Downy oak (Quercus pubescens Willd.) is a deciduous species characterized by high morphological variability ([24]) and a widespread distribution range, from western to eastern Europe ([15]). Pubescent oak covers with monospecific stands 876 000 and 850 000 ha in France and Italy, respectively ([13], [14]). In other Mediterranean countries, e.g., Slovenia, Croatia, Montenegro, and Albania, the growing stock of mixed or pure stands of this species is rather limited, according to the national forest inventories. In general, the wood of pubescent oak is scarcely considered as industrial lumber and is mainly used as firewood. In the past, it was largely employed for rail crossbeams, while nowadays it is occasionally used for carpentry, boat-building, or packaging. It is well known that through adequate modification processes, its wood properties may be improved, creating a new and environmental-friendly material ([11]).
Thermal treatment of wood is applied since the beginning of the last century, when several studies demonstrated the reduced equilibrium moisture content and consequently reduced dimensional shrinkage of wood exposed to a high temperature ([17], [27], [18]). Lately, a great interest in thermal processing of wood had been rising, and several types of thermal treatments have been developed. Most studies aimed at analyzing the variation in the different treatment phases of several wood parameters, such as moisture content, dimensional stability, durability, mass loss, color changes, mechanical properties, and chemical modifications ([7]).
Nowadays, the industrial and scientific interest in thermally treated wood is undergoing a “new age”, promoted by the growing demand for environmental-friendly construction products. Although the scientific literature is rich of studies describing the improved technological properties of several species, scant information is available on the wood of pubescent oak. In general, based on its wood properties, the choice of the most suitable thermal treatment for pubescent oak is often troublesome.
Pubescent oak wood veining is macroscopically indistinguishable from other oak species. In fact, there are no significant differences in its strength properties as compared with other deciduous oak species, though pubescent oak wood is heavier with a large shrinkage, and consequently very prone to cracking ([9]). In addition, the presence of reaction wood, as well as cross grain or internal tensions, can cause serious warping, such as bowing, crooking, or twisting, and cross breaks develop in the areas of major shrinkage ([10]). Also, pubescent oak wood shows remarkable chromatic differences between sapwood and heartwood, being the latter usually darker. Furthermore, its high level of extractives, along with other features such as tylosis, make this wood less permeable and more difficult to be treated with chemical additives ([12], [3]). Presently, not enough information is available for a suitable industrial exploitation of pubescent oak wood.
The starting hypothesis of this study was that the factors limiting the utilization of pubescent oak wood could be reduced by applying appropriate hydrothermal treatments. In particular, a reduction in the equilibrium moisture content and consequently an improvement in the dimensional stability could be possibly achieved. Therefore, the main objective of this work was to determine the influence of thermal and combined (steam and heat) treatments on some basic properties of pubescent oak wood, such as dimensional variations, equilibrium moisture content, mass loss, compression strength, lignin content, and color variation.
Material and methods
Four pubescent oak trees were sampled from a mixed Quercus spp. forest located in the Basilicata region (southern Italy). Some 30mm-thick boards were sawed in parallel from each log. From each board, samples without defects with dimensions of 20 mm (tang) × 20 mm (rad) × 40 mm (long) were randomly selected and cut. Overall, 180 wood samples were obtained, none of which included the pith section.
Samples were then assigned to nine different treatment groups, equally distributed between heartwood and sapwood. The description of treatments for each group is reported in Tab. 1. The control group (Group 1) was not subjected to any treatment. Groups 2-5 were subjected to a thermal treatment, while groups 6-9 received a combination of steaming and heat treatment. Each group was subjected to a specific treatment in terms of steaming, heating, and time (Tab. 1).
Tab. 1 - Treatment schedules and number of samples (n) analyzed. (ST): steaming.
Group | Treatment (°C, h) |
Steaming (°C) |
Heating (°C) |
Time (h) |
Heartwood (n) |
Sapwood (n) |
Total (n) |
---|---|---|---|---|---|---|---|
1 | Control | - | - | - | 10 | 10 | 20 |
2 | 150 × 3 h | - | 150 | 3 | 10 | 10 | 20 |
3 | 150 × 6 h | - | 150 | 6 | 10 | 10 | 20 |
4 | 200 × 3 h | - | 200 | 3 | 10 | 10 | 20 |
5 | 200 × 6 h | - | 200 | 6 | 10 | 10 | 20 |
6 | ST + 150 × 3 h | 120 | 150 | 3 | 10 | 10 | 20 |
7 | ST + 150 × 6 h | 120 | 150 | 6 | 10 | 10 | 20 |
8 | ST + 200 × 3 h | 120 | 200 | 3 | 10 | 10 | 20 |
9 | ST + 200 × 6 h | 120 | 200 | 6 | 10 | 10 | 20 |
Prior to any treatment, all samples were conditioned at 20 ± 2 °C and 65% relative humidity until a constant mass was reached, and then dried in an oven at 103 °C for 24 h, in order to balance the moisture content. The mass, dimensions, and color coordinates of each specimen were measured before and after treatment.
Steaming
Eighty samples were subjected to an indirect steaming inside an autoclave with a maximum capacity of 23 L (Vapormatic 770/A, Asal s.r.l., Cernusco, Italy). The cycle was completely automatic, thermoregulated, and controlled by an HMOS microprocessor that enables programming different times and temperatures (from 100 to 130 °C). The performed steaming cycles are displayed in Fig. 1. The main goal of the steaming phase was to slow the release of internal tensions, and consequently to reduce the formation of surface checks and sharpness, which are particularly present in pubescent oak wood. Afterward, the dimensions, mass, and color coordinates were also determined.
Fig. 1 - Steaming cycle. The red dashed line represents the temperature (°C), and the black line represents the pressure (bar).
Heating
The samples were treated in a small heating unit with ± 1 °C accuracy under atmospheric pressure, according to two different heating cycles (see Tab. 1). In order to reduce the formation of surface checks during the thermal treatment, a bowl containing 1 L of distilled water was placed in the oven at the beginning of each cycle. The samples were then weighed, and their tangential, radial, and axial dimensions measured before and after each treatment.
Characterization tests
After treatment, the samples were reconditioned in a climatic chamber at 20 ± 2 °C and 65% relative humidity. The differences in weight due to moisture content adsorption were measured daily during the first week, and weekly thereafter.
The equilibrium moisture content and radial-tangential-total swelling were measured on strictly radial-tangential-oriented samples with dimension of 20 × 20 × 40 mm according to UNI-ISO-3130 ([39]), UNI-ISO-4859 ([42]) and UNI-ISO-4860 ([43]) standards. The swelling was determined as follows (exemplified for tangential, tg
- eqn. 1):
Tab. 2 - Equilibrium moisture content (ECM, %) at 4 weeks after treatment. Values in parentheses are standard deviations. Different letters indicate significant differences between means after Duncan’s multiple range test (p<0.05). (ST): steaming.
Group | Treatment (°C, h) | EMC at the 4th week (%) | Decrease (%) |
---|---|---|---|
1 | Control | 7.6 (0.4) d,e | - |
2 | 150 × 3 h | 7.8 (0.3) e | 1.8 |
3 | 150 × 6 h | 7.7 (0.4) d,e | 0.2 |
4 | 200 × 3 h | 6.2 (0.4) b | -19.2 |
5 | 200 × 6 h | 5.8 (0.4) a | -23.8 |
6 | ST + 150 × 3 h | 7.2 (0.4) c | -6.0 |
7 | ST + 150 × 6 h | 7.5 (0.3) d | -2.2 |
8 | ST + 200 × 3 h | 6.2 (0.3) b | -18.5 |
9 | ST + 200 × 6 h | 5.7 (0.3) a | -25.9 |
The mass loss was determined by weighing the boards of the stack prior to the thermal treatment (at a moisture content of 0%) and immediately after, and it was expressed as percentage. The wood density (kg m-3) was determined according to the UNI-ISO-3131 ([40]) standard and was expressed as mass per volume, where mass (in g) was calculated by an electronic scale with an accuracy of ± 0.001 g; and the volume (V
, in cm3) was calculated by measuring the three dimensions with a caliper having an accuracy of ± 0.001 cm3. The Klason lignin content was assessed by a modified TAPPI ([31]) method, resulting from the attack of sulfuric acid on the extractive-free material and quantifying the lining as a precipitate. To determine the compression strength parallel to the grain, the samples were tested according to UNI-ISO-3787 ([41]) after 4 weeks in a climatic chamber (see Tab. 2 for the average moisture content values). According to the CIELAB system, color parameters were used to verify the color modifications. L
*, a
*, and b
* were measured on each specimen on the radial and tangential sections of untreated and treated wood, and their average values were calculated for each treatment. Measures were taken using a Minolta CM-2002 spectrophotometer (Minolta Corp., Osaka, Japan) with a pulsed Xenon arc light source on measurement areas of 8 mm in diameter. Color variations (exemplified below for L
*) were determined according to the following formula (eqn. 2):
The color changes of ΔL
*, Δa
*, and Δb
* of each sample before and after treatments were also used to calculate the total color change (ΔE
) according to the following formula (eqn. 3):
The difference after treatments between heartwood and sapwood was determined as follows (exemplified below for L
* - eqn. 4):
Analysis of variance was performed by applying the general factor model procedure implemented in the software package SPSS ver. 10.0. Post-hoc Duncan’s multiple range test (α = 0.05) was used to test for differences among means of each measured parameter in relation to the treatment applied.
Results and discussion
Moisture content variation
The moisture adsorption was mainly related to the temperature used in the process (Fig. 2 and Tab. 2). After 4 weeks in the climatic chamber, moisture content of the control samples was 7.6%, which was not statistically different from treatments 2, 3, or 7. In contrast, the reduction in water adsorption was statistically significant when the wood was heated at 200 °C (Tab. 2).
Treatments 5 and 9, which were subjected to higher temperature for a longer duration, resulted in a low water adsorption after 4 weeks in a climatic chamber (5.8% and 5.7%, respectively). In contrast, the samples heated at 150 °C for 3 h and steamed showed a low reduction (7.2%) of water adsorption, as compared with the controls after 4 weeks. These results indicated that a higher temperature is required to obtain a significant improvement in the moisture content. Furthermore, similar results were obtained using the same temperature during the heating cycle, regardless of whether steaming treatment was applied. Nevertheless, the heating duration significantly affected the moisture content (treatments 4, 5, 8, and 9).
Sundqvist ([28]) reported that noticeable changes in the lignin structure occur at a temperature of approximately 120 °C. For wet conditioned wood, lignin can plasticize and change in structure even at 100 °C. However, the samples in our study were not steamed under wet conditions. On the contrary, we previously reported about significant variations in moisture content at 120 °C both for steaming and heating when wet wood was used ([36]). These facts confirmed the hypothesis that the steaming process assumes a greater importance under wet conditions as compared with dry conditions. Thus, in our study, the positive effect of steaming on moisture content reduction was weakly depicted (Tab. 2), compared with other similar studies ([35], [36]).
Sapwood and heartwood
We found that sapwood of pubescent oak adsorbed water more easily than heartwood when exposed to severe treatments (200 °C - Fig. 3). After the adsorption phase, the relative difference of moisture content between sapwood and heartwood increased with temperature and duration, both in the steamed and not steamed samples. In fact, hygroscopic inertia seemed to increase, except for treatments 2 and 7, in which the differences between sapwood and heartwood moisture content were similar to those of the control.
Fig. 3 - Moisture content in sapwood and heartwood after 4 weeks in the climatic chamber. Gray and white boxes are heartwood and sapwood, respectively. The dotted line represents the relative difference (%) between sapwood and heartwood. The shaded area refers to samples submitted to the steaming treatment.
Considering the general and significant reduction of moisture content previously observed (except for treatments 2, 3, and 7 - Tab. 2), a higher increase in water adsorption was detected in sapwood than in heartwood. Treatment with both heat and steam seemed to increase the hygroscopic inertia during the adsorption process, mainly in the heartwood where the presence of tylosis (Fig. 4) may decrease the permeability ([6]). Tylosis is the result of enzymatic hydrolysis breaking down the pit membrane between a parenchyma cell and an adjacent vessel. This process allows a portion of the parenchmya protoplast to extrude into the vessel lumen ([30]). Sohar et al. ([25]) have reported that in oak species, sapwood is distinguished from heartwood by its lighter color and the absence of tylosis in earlywood vessels. Thus, the degree of tylosis in the Quercus spp. ([12], [20]) may affect the fluid flow, which limits the moisture uptake, hampers the physical movement of pathogens through the wood, and increases its durability ([30]). Moreover, in the treatment at 200 °C with steaming, groups 8 and 9 showed a reduced moisture content difference between sapwood and heartwood, compared to groups 4 and 5. Therefore, the presence of tylosis is crucial to improve the characteristics of pubescent oak subjected to hydrothermal treatment.
Fig. 4 - Tylosis (T) and color differences between the heartwood (left) and the sapwood (right) of pubescent oak.
Swelling
Wood swelling was measured after 4 weeks in a climatic chamber (Tab. 3). In the tangential direction, the groups heated at 200 °C (treatments 4, 5, 8, and 9) adsorbed a lower amount of water. The treated material also showed a high dimensional stability: this is fundamental considering that pubescent oak wood is particularly prone to volume and shape variations.
Tab. 3 - Tangential and radial swelling after 4 weeks in a climatic chamber at 20 °C and 65% relative humidity. Values in parentheses are standard deviations. Different letters indicate significant differences between means after Duncan’s multiple range test (p<0.05). (ST): steaming.
Group | Treatment (°C, h) |
Tangential Swelling (%) |
Decrease (%) |
Radial swelling (%) |
Decrease (%) |
---|---|---|---|---|---|
1 | Control | 2.1 (0.6) c | - | 1.4 (0.4) d | - |
2 | 150 × 3 h | 1.9 (0.5) c | -12.8 | 1.3 (0.3) b, c | -6.3 |
3 | 150 × 6 h | 1.9 (0.3) c | -8.3 | 1.2 (0.3) b, c | -11.1 |
4 | 200 × 3 h | 1.5 (0.2) a | -31.0 | 1.2 (0.4) b | -14.8 |
5 | 200 × 6 h | 1.4 (0.5) a | -35.7 | 0.9 (0.3) a | -31.4 |
6 | ST + 150 × 3 h | 1.8 (0.6) b,c | -15.5 | 1.3 (0.3) b, c | -2.7 |
7 | ST + 150 × 6 h | 1.9 (0.6) c | -9.4 | 1.3 (0.5) b, c | -5.8 |
8 | ST + 200 × 3 h | 1.4 (0.4) a | -32.0 | 1.4 (0.3) c | -1.3 |
9 | ST + 200 × 6 h | 1.5 (0.4) a,b | -29.1 | 1.4 (0.4) d | 5.0 |
Contrasting results were found regarding wood swelling in the radial direction. Our data indicated that the influence of moisture adsorption on swelling was limited to the tangential direction of pubescent oak wood, at least in the treatment combining steaming and heating. In fact, in the radial direction, the treatment effect was remarkable only in samples not treated with steaming, likely because during steaming permanent changes occurred in samples due to swelling. This assumption is in accordance with Babicki et al. ([2]), who found an increase of beech wood volume during the first period of hydrothermal treatment (1-5 h). On the other hand, Rybarczyk & Ganowicz ([22]) described detailed information regarding the swelling pressure of wood, indicating permanent deformation in both the radial and tangential directions.
Differences in behavior between the radial and tangential directions are clearly related to the anisotropy of wood ([34]), with particular reference to the radial ray cells, which are large and abundant in pubescent oak, thus affecting the physical and mechanical properties of wood ([5]). It has been hypothesized that during the absorption of water, the radial ray cells may act as a longitudinal barrier to the movement of wood ([36]). Reiterer et al. ([21]) also confirmed that the volume fraction of ray cells affects wood mechanical properties in the radial direction, while has negligible effect in the tangential direction. This is likely a consequence of the contrasting anisotropy and geometry of the ray cells as compared with other wood tissue cells ([5]).
Mass loss and density variation
Our analysis revealed that the oven-dry mass loss was not related to the steaming treatment, but it was only associated with the heat treatment temperature and its duration (Tab. 4). Such results indicated that the mass loss was significantly higher in material treated at 200 °C for 6 h with or without steaming, while heat treatment at 150 °C led to slight mass changes.
Tab. 4 - Mean density (kg m-3) at the origin (wet state), after treatments, and after 4 weeks in a climatic chamber. Oven dry mass loss (%) and standard deviation (in parentheses) after thermal treatments. Different letters indicate significant differences between means after Duncan’s multiple range test (p<0.05). (ST): steaming; (ns): not significant.
Group | Treatment (°C, h) |
Origin (kg m-3) |
After steaming (kg m-3) |
After heat treatment (kg/m-3) |
Mass loss (%) |
4th week (kg m-3) |
---|---|---|---|---|---|---|
1 | Control | 857 a | - | - | 0 | 842 a,b,c |
2 | 150 × 3 h | 874 a,b | - | 826 c,d | 0.3 (0.1) a | 863 c |
3 | 150 × 6 h | 880 a,b | - | 830 c,d | 0.6 (0.1) b,c | 865 c |
4 | 200 × 3 h | 903 b | - | 846 d | 2.5 (0.3) g | 874 c |
5 | 200 × 6 h | 881 a,b | - | 819 b,c,d | 3.5 (0.4) f | 846 b,c |
6 | ST + 150 × 3 h | 871 a,b | 822 ns | 783 a | 0.4 (0.1) a,b | 814 a,b |
7 | ST + 150 × 6 h | 871 a,b | 820 ns | 780 a | 0.6 (0.2) c | 811 a |
8 | ST + 200 × 3 h | 880 a,b | 834 ns | 786 a,b | 2.1 (0.3) d | 810 a |
9 | ST + 200 × 6 h | 900 b | 851 ns | 805 a,b,c | 3.7 (0.4) f | 826 a,b |
Modifications of hemicellulose, cellulose, and lignin occur at high temperatures, influencing the wood properties such as mass loss, hygroscopicity, mechanical resistance, swelling, natural durability, etc. Therefore, the extent of the above modifications depends mainly on the steaming and heating temperatures, as well as the treatment duration ([7]).
Concerning the wood density variation (Tab. 4), a significant difference only was observed in the samples that had first been subjected to steaming, suggesting that the application of this treatment may be useful for uniformly preparing the wood for further processing. In fact, hydrothermal treatment significantly influenced the density of samples previously steamed. Groups 6 to 9 all showed a lower wood density after the combination of steaming and heat treatment, as compared with both the control sample (group 1) and samples subjected to thermal treatment only (groups 2-5). These results paralleled those found by Kartal et al. ([16]), in a study on beech wood submitted to steaming treatment. Assor et al. ([1]) hypothesized that steaming may affect the degradation of wood components by promoting hydrolysis of some chemical compounds and a partial removal of extractives ([16]), with a consequent effect on the wood density.
Compression strength
The compression strengths of the samples heated at 200 °C with no steaming were only slightly greater than those of the control: the mechanical resistance of group 4 increased by 7.4% and that of group 5 increased by 8.3%. Meanwhile, no significant increases were observed in groups 2 and 3 (Fig. 5). In contrast, the compression strength parallel to the grain was clearly increased after steaming and heat treatment (Fig. 5) at both 150 and 200 °C (groups 6-9). Compared to the control, an increase of strength from 15.7% (group 7) to 17.4% (group 9) was observed. Similar findings were reported by Boonstra et al. ([4]), who ascribed such results to an increased cross-linking of the lignin polymer network, which improves the strength of the middle lamella, consequently affecting the strength properties of the cell wall parallel to the grain.
Fig. 5 - Compression strengths of samples with the moisture content as reported in Tab. 2. The shaded area refers to samples submitted to the steaming treatment. Different letters indicate significant differences between means after Duncan’s multiple range test (p<0.05).
The lower moisture content (Tab. 2) is likely a key factor accounting for the observed improvement of some mechanical properties after the thermal treatment. However, higher temperatures and a longer process duration may enhance the risk of wood fiber modifications, making wood less resistant to compression ([7], [19]). Therefore, an appropriate balance among temperature, moisture content, pressure, and treatment duration must be maintained in order to improve wood properties.
Color variation
The color measurements of the wood samples showed a general darkening of the surface (Fig. 6). Darkening was more evident for the stronger treatments (ST + 200 °C × 3 h and 6 h), while the weakest treatments (150 °C × 3 h) showed the lowest reduction (-2.9). Little variation of the red index in relation to treatment was observed, whilst the strongest treatments produced significant variation of the yellow index. As reported by Terziev ([32]), these results were probably due to the enrichment of sugar and nitrogenous compounds at the wood surface during treatment. In addition, statistical analysis confirmed that hydrothermal treatments led to significant total color modifications (ΔE
), with a great difference between treated and untreated wood when samples were submitted to the highest temperature. Indeed, our results indicate that temperature and (to a less extent) time of treatment significantly affected the color variation (ΔE
- Fig. 6), as already reported by Tolvaj & Molnar ([38]). Changes in ΔE
were mainly due to L
* and less affected by a
* and b
*.
Fig. 6 - Influence of treatments on the average color parameters. The shaded area refers to samples submitted to the steaming treatment.
Comparison between sapwood and heartwood submitted to the same treatment indicated that the hydrothermal treatment reduced color differences inside the wood (Tab. 5). According to Sundqvist & Morén ([29]), a prevalent role in wood color changes after hydrothermal treatments is played by extractives, which are more abundant in heartwood than in sapwood and are the first compounds degraded during that process. In addition, hydrolysis is an important reaction occurring when wood is heat-treated and moisture is present in wood cells or under steaming conditions. The temperature and duration of heat treatment during steaming has been found as the most important factor affecting darkening in hardwoods ([28]). Discoloration of oak also might occur at lower temperatures, though only when the wood is wet and the drying treatment is prolonged ([26], [23]).
Tab. 5 - The color coordinate differences between sapwood and heartwood. (ST): steaming.
Group | Treatment (°C, h) |
Lightness ( L *) |
Green-red axis ( a *) |
Blue-yellow axis ( b *) |
---|---|---|---|---|
1 | Control | -13.4 | 2.7 | -9.5 |
2 | 150 × 3 h | -5.1 | -0.7 | -8.1 |
3 | 150 × 6 h | -10.3 | -11.2 | -13.9 |
4 | 200 × 3 h | -9.8 | -3.8 | -18.2 |
5 | 200 × 6 h | -8.6 | -5.9 | -24.8 |
6 | ST+ 150 × 3 h | -12.6 | -7.9 | -21.2 |
7 | ST + 150 × 6 h | -7.8 | -10.5 | -12.1 |
8 | ST + 200 × 3 h | -10.8 | -14.3 | -21.9 |
9 | ST + 200 × 6 h | -5.0 | -8.4 | -12.0 |
Lignin content
The proportion of lignin content in the wood remained almost unchanged at 150 °C, but increased in the samples heated at 200 °C (Tab. 6). However, such results should be considered with caution, as some of the thermally degraded carbohydrates may have been incorporated within the lignin during its determination ([33]), leading to the overestimation of lignin content ([44], [45]). It is important to note that the lignin percentage observed in the heartwood remained almost unchanged or decreased, leading to the assumption that a fixed percentage of lignin remained ([44]). The underlying cause is unknown and deserves to be further investigated. In fact, the lignin content increased in the sapwood samples as compared with the control, but not in the heartwood samples, indicating the importance of steaming when wet wood is used. In a recent study, Todaro et al. ([37]) found contrasting results for Turkey oak wood, showing that an increase of lignin content in the heartwood after a high temperature treatment was associated with a previous steaming treatment on wet wood. However, Dashti et al. ([6]) highlighted the difference of lignin content between sapwood and heartwood in terms of their different permeability and diffusion coefficients in Quercus infectoria Olivier. The authors stated that the presence of tylosis in the heartwood, which lowers the diffusion rate of water vapor through wood, has a significant effect on the final impact of steaming treatment on the lignin content. In our study, the low presence of water during steaming and consequently the reduced destruction of the cellular wall structure caused a slower degradation of the heartwood compounds and reduced the lignin content. Funaoka et al. ([8]) also suggested that the water contained in the wood enhances the condensation of lignin.
Tab. 6 - Average lignin content in the wood (ALW), sapwood, and heartwood. Sapwood and heartwood variation (%) are compared with the control. (SD): Standard deviation; (ST): steaming.
Group | Treatment (°C, h) |
ALW (%) |
SD | Sapwood (%) |
Sapwood variation (%) |
Heartwood (%) |
Heartwood variation (%) |
---|---|---|---|---|---|---|---|
1 | Control | 26.5 | 2.7 | 24.6 | 0 | 28.4 | 0 |
2 | 150 × 3 h | 27.5 | 1.6 | 26.4 | 7.3 | 28.7 | 0.7 |
3 | 150 × 6 h | 25.8 | 0.7 | 25.3 | 2.8 | 26.3 | -7.4 |
4 | 200 × 3 h | 27.2 | 0.8 | 27.8 | 8.1 | 28.6 | -2.6 |
5 | 200 × 6 h | 28.2 | 0.5 | 26.6 | 13.0 | 27.7 | 0.5 |
6 | ST+ 150 × 3 h | 25.6 | 0.1 | 25.6 | 3.8 | 25.7 | -9.6 |
7 | ST + 150 × 6 h | 25.1 | 0.7 | 25.6 | 4.0 | 24.6 | -13.3 |
8 | ST + 200 × 3 h | 26.5 | 1.8 | 25.2 | 2.4 | 27.7 | -2.6 |
9 | ST + 200 × 6 h | 28.0 | 0.9 | 27.3 | 10.9 | 28.6 | 0.5 |
Conclusions
The results of this study confirmed that hydrothermal treatments reduce the hygroscopic and dimensional variations of pubescent oak wood, which is currently underused due to its poor dimensional stability. Steaming and heating temperature were found to significantly affect water adsorption, strength, darkening, and lignin content after treatment. Interesting results about moisture content and relative swelling were found by applying the combined treatment of steaming and heating at 200 °C, while the treatments at 150 °C gave poorer results regardless of steaming. Furthermore, the combined effect of steaming and heating caused an increase in the axial compression strength.
Considering that pubescent oak has a high-density wood characterized by wide rays and internal stresses, the usage of high temperatures could determine undesired collapses. Hydrothermal treatments led to significant modifications of the wood color. The great color difference between the sapwood and heartwood of pubescent oak could be weakened by steaming. Concerning the lignin content, a similar trend was found for sapwood and heartwood. Moreover, more lignin was detected in samples subjected to high temperature treatments, while its content in sapwood was higher than in heartwood.
Since pubescent oak is widespread in several Mediterranean countries, the improvement of the technological properties of its wood may be of high interest, possibly reducing the amount of imported timber with positive economic effects. Though the availability of high-quality forest stands of this species is currently limited, it could be advisable the use of this wood to replace other oak species used in product manufacturing.
The present work has focused on several parameters of pubescent oak wood that could be interesting for industry. However, due to the relatively small sample size adopted, further investigations are needed to confirm the modifications and technological improvements observed in this study.
Acknowledgments
We thank Giuseppe Maltempo and Pasquale Di Chicco, undergraduate students of the University of Basilicata, for providing assistance during the laboratory measurements.
References
Gscholar
Gscholar
Gscholar
Gscholar
Gscholar
Online | Gscholar
Gscholar
Gscholar
Gscholar
Gscholar
Gscholar
Gscholar
Gscholar
Gscholar
Gscholar
Gscholar
Gscholar
Gscholar
Authors’ Info
Authors’ Affiliation
Angelo Rita
Nicola Moretti
School of Agricoltural, Forest, Food and Environmental Science, University of Basilicata, Potenza (Italy)
Roberto Zanuttini
Department of Agricultural, Forest and Food Sciences, University of Torino (Italy)
Department of Agriculture, Division of Biology and Protection of Agricultural and Forest Systems (BIPAF), University of Naples “Federico II” (Italy)
Corresponding author
Paper Info
Citation
Todaro L, Rita A, Negro F, Moretti N, Saracino A, Zanuttini R (2015). Behavior of pubescent oak (Quercus pubescens Willd.) wood to different thermal treatments. iForest 8: 748-755. - doi: 10.3832/ifor1348-007
Academic Editor
Giacomo Goli
Paper history
Received: May 12, 2014
Accepted: Oct 01, 2014
First online: Feb 16, 2015
Publication Date: Dec 01, 2015
Publication Time: 4.60 months
Copyright Information
© SISEF - The Italian Society of Silviculture and Forest Ecology 2015
Open Access
This article is distributed under the terms of the Creative Commons Attribution-Non Commercial 4.0 International (https://creativecommons.org/licenses/by-nc/4.0/), which permits unrestricted use, distribution, and reproduction in any medium, provided you give appropriate credit to the original author(s) and the source, provide a link to the Creative Commons license, and indicate if changes were made.
Web Metrics
Breakdown by View Type
Article Usage
Total Article Views: 50148
(from publication date up to now)
Breakdown by View Type
HTML Page Views: 43284
Abstract Page Views: 2522
PDF Downloads: 3132
Citation/Reference Downloads: 20
XML Downloads: 1190
Web Metrics
Days since publication: 3566
Overall contacts: 50148
Avg. contacts per week: 98.44
Article Citations
Article citations are based on data periodically collected from the Clarivate Web of Science web site
(last update: Feb 2023)
Total number of cites (since 2015): 8
Average cites per year: 0.89
Publication Metrics
by Dimensions ©
Articles citing this article
List of the papers citing this article based on CrossRef Cited-by.
Related Contents
iForest Similar Articles
Research Articles
Physical and mechanical characteristics of poor-quality wood after heat treatment
vol. 8, pp. 884-891 (online: 22 May 2015)
Research Articles
Characterization of VOC emission profile of different wood species during moisture cycles
vol. 10, pp. 576-584 (online: 08 May 2017)
Research Articles
The physicomechanical and thermal properties of Algerian Aleppo pine (Pinus halepensis) wood as a component of sandwich panels
vol. 15, pp. 106-111 (online: 21 March 2022)
Research Articles
Changes in moisture exclusion efficiency and crystallinity of thermally modified wood with aging
vol. 12, pp. 92-97 (online: 24 January 2019)
Review Papers
Moisture in modified wood and its relevance for fungal decay
vol. 11, pp. 418-422 (online: 05 June 2018)
Research Articles
Mechanical and physical properties of Cunninghamia lanceolata wood decayed by brown rot
vol. 12, pp. 317-322 (online: 06 June 2019)
Research Articles
Testing common hornbeam (Carpinus betulus L.) acetylated with the Accoya method under industrial conditions
vol. 10, pp. 948-954 (online: 19 December 2017)
Research Articles
NIR-based models for estimating selected physical and chemical wood properties from fast-growing plantations
vol. 15, pp. 372-380 (online: 05 October 2022)
Research Articles
Estimation of total extractive content of wood from planted and native forests by near infrared spectroscopy
vol. 14, pp. 18-25 (online: 09 January 2021)
Research Articles
Kinetic analysis of poplar wood properties by thermal modification in conventional oven
vol. 11, pp. 131-139 (online: 07 February 2018)
iForest Database Search
Search By Author
Search By Keyword
Google Scholar Search
Citing Articles
Search By Author
Search By Keywords
PubMed Search
Search By Author
Search By Keyword