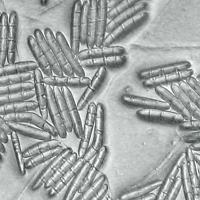
Potential spread of forest soil-borne fungi through earthworm consumption and casting
iForest - Biogeosciences and Forestry, Volume 8, Issue 3, Pages 295-301 (2015)
doi: https://doi.org/10.3832/ifor1217-008
Published: Aug 26, 2014 - Copyright © 2015 SISEF
Research Articles
Abstract
To test if forest soil-borne fungi concerned with plant health can be selectively dispersed by earthworms, 10 fungal species isolated from 5 forests were presented, at 2 concentrations, to 3 ecologically distinct earthworm species in laboratory trials. Between 5 and 13 days after introduction, casts were collected, where possible, from each earthworm species fed with a different fungus. These casts were analysed, using molecular methods, for the presence of the given fungus and its vitality verified through traditional plating techniques. The research confirmed that earthworms have an important role in dispersal of soil fungi in forests, and that such activity can depend on the taxonomical position of the fungus, ecological category of the earthworm species involved and the fungal concentration. In certain instances there is a suggestion that some fungi may be toxic to some earthworms at the given concentrations, which equated to those within and outside of the rhizosphere.
Keywords
Earthworms, Soil-borne Fungi, Fungal Inoculum, Ecology, Dispersal
Introduction
Since the late 1940s, there has been a growing interest in soil mycology and soil-borne fungal diseases of plants, motivating studies on soil fungi and their ecology ([63], [64], [9]). Such fungi are involved in many plant-soil relationships, including water and nutrient uptake and cycling, plant disease expression or suppression. From a functional standpoint, such fungi can be grouped according to energy derivation: (i) decomposers (saprotrophic), utilizing dead organic material, sometimes acting antagonistically with others; (ii) mutualists (mycorrhizal), colonizing plant roots, supplying soil nutrients and protection against root parasites in exchange for sugars and possibly other components; (iii) parasites, reducing the growth of plant structures or causing diseases by acting as pathogens.
Relationships among soil-borne fungal species involved in forest plant fitness are complex, in that expansion and spread of one population versus another linked and associated with other variables (e.g., plant susceptibility, soil pH, temperature, humidity) may lead to changes in plant health. In line with well-known biocontrol strategies ([7]), a parasitic species can seldom express its full pathogenicity against a plant when sufficient mutualistic and/or antagonistic species are present outside of, or within, the rhizosphere ([67], [36]). The rhizosphere represents a peculiar ecological niche: a common physiological stress on a healthy plant (e.g., an unusual drought period) can easily be reflected in different root exudates, such as sugars and other components, which are important signals of the plant vigor to rhizosphere inhabitants. In this way, a multifaceted dynamic of microbiological interactions, which may awaken their resting stages or chemotactically attract their mobile propagating organs, could lead to establishment of root diseases (e.g., by Phytophthora, Armillaria, Fusarium, Nectria, Verticillium species), the most dangerous in forestry ([39]). In an established forest soil, decomposer fungi (sometimes with an antagonistic behavior against other microorganisms, including parasites, such as Trichoderma) are commonly present both within and outside of the rhizosphere. By contrast, mutualistic fungi, usually in the rhizosphere (e.g., Laccaria, Pisolithus, Suillus, Xerocomus), can produce toxic metabolites, inhibiting infection by parasitic fungi or physically masking root tips ([62]). Therefore, the higher is fungal abundance, dispersal rate and positive synergistic effects useful to plants (by decomposers and mutualists), the lower the probability of root disease is likely to be.
Soil fungi represent a large biomass in the soil ([30]), providing a rich and abundant resource for fungivorous soil invertebrates ([27], [65], [70]). Among the latter, earthworms have an active role in soil ecology, altering soil structure, water movement, nutrient dynamics, and plant growth ([37]). However, different earthworm species inhabit different parts of the soil, have distinct feeding strategies and can be separated into three major ecological groups ([4]). These are based primarily on feeding and burrowing habits: (i) epigeic species, living within or close to surface plant litter; (ii) endogeic species, moving and living in the upper soil strata and feeding primarily on soil and associated organic matter; (iii) anecic species, feeding on organic matter and inhabiting semi-permanent burrow systems that may extend vertically down several meters into the soil.
Therefore, earthworms and soil fungi are closely intertwined by direct and indirect grazing, altering spore viability during passage through the gut of the earthworm, and altering the dispersal patterns of fungal propagules by transport ([14], [19]). Edwards ([21]) demonstrated that earthworms may be capable of selectively digesting some fungal species. However, little is known on the spread of fungi in relation to fungal species and their involvement in forest plant health.
The aim of the current research was to determine whether 10 forest soil-borne fungal species (decomposers, mutualists and parasites), could be dispersed through the earthworms’ casts by selected forest dwelling earthworms. To simulate what occurs within and outside of the rhizosphere, where direct plant-soil system interactions take place, the fungal species were offered to earthworms at two different concentrations.
Material and Methods
Fungal selection and isolation
Ten soil-borne fungal species belonging to 3 phyla collected from 5 forests located in the Veneto region (north-eastern Italy - Tab. 1) were selected according to the parasitic, mutualistic or hyperparasitic groups with forest plants. Soils from these and similar forests are known to support earthworm communities which include the 3 species selected for this trial ([71], [22]).
Tab. 1 - Major forest features from sites where experimental fungal species were isolated.
Site | Forest type, elevation (m a.s.l.), coordinates |
Dominant tree species |
Additional tree species |
---|---|---|---|
1 | Pure coniferous (1572 m) 46° 23′ N / 12° 10′ E |
European larch Larix decidua Mill. |
Picea abies (L.) Karst. |
2 | Mixed forest (1117 m) 45° 53′ N / 11° 28′ E |
Norway spruce Picea abies (L.) Karst. |
Abies alba Mill., Fagus sylvatica L. |
3 | Broadleaved (54 m) 45° 38′ N / 11° 31′ E |
European alder Alnus glutinosa L. |
Acer pseudoplatanus L., Fraxinus excelsior L. |
4 | Broadleaved (9 m) 45° 49′ N / 12° 31′ E |
Common oak Quercus robur L. |
Carpinus betulus L. Fraxinus oxycarpa Bieb., Ulmus minor Mill., Prunus avium L. |
5 | Broadleaved (2 m) 45° 07′ N / 12° 15′ E |
Holm oak Quercus ilex L. |
Ostrya carpinifolia Scop., Fraxinus ornus L. |
The Oomycete Phytophthora cactorum (Lebert & Cohn) J. Schröt. (plant parasite), the Ascomycete Neonectria radicicola (Gerlach & L. Nilsson) Mantiri & Samuels [anam. Cylindrocarpon destructans (Zinssm.) Scholten], Fusarium reticulatum Mont., Verticillium dahliae Kleb. (plant parasites) and Trichoderma harzianum Rifai (fungal hyperparasite), and the Basidiomycote Armillaria ostoyae (Romagn.) Herink. (plant parasite), Laccaria laccata (Scop. ex Fr.) Bk. & Br., Pisolithus arhizus (Scop.) Rauschert, Suillus grevillei (Klotzsch) Singer and Xerocomus chrysenteron (Bull.) Quél. (plant mutualists) were isolated and purified in 2008 (Tab. 2).
Tab. 2 - Species (strain and isolation site according to Tab. 1), main behaviour, substrate of isolation and growing media. (PDA): Potato Dextrose Agar (BD, Becton, Dickinson and Company, NJ, USA); (PDB): Potato Dextrose Broth (BD, NJ, USA); (MMN): modified Melin-Norkrans liquid medium; (MMNA): MMN added with 1.5 % agar; (TME): Trichoderma medium E.
Species strain code (site code) | Main behaviour | Substrate of isolation |
Isolation and purification media / liquid culture media |
---|---|---|---|
A. ostoyae (AM-13 2) |
parasitic (root rot - [53]) | Sporocarp below Norway spruce | PDA / PDB |
N. radicicola (CD-2 3) |
parasitic (root rot, wilt - [43]) | Rootlets from European alder | PDA / PDB |
F.reticulatum (FR-1 4) |
parasitic (root rot, wilt - [42]) | Rootlets from Common oak | PDA / PDB |
P. cactorum (PC-6 5) |
parasitic (root rot, wilt - [69]) | Rootlets from Holm oak | PDA / PDB |
V. dahliae (VD-4 4) |
parasitic (root rot, wilt - [47]) | Rootlets from Prunus avium | PDA / PDB |
L. laccata (LL-4 2) |
mutualistic (ectomycorrhizal - [17]) | Sporocarp below Norway spruce | MMNA / MMN |
P. arhizus (PT-7 4) |
mutualistic (ectomycorrhizal - [20]) | Sporocarp below Common oak | MMN / MMN |
S. grevillei (SE-1 1) |
mutualistic (ectomycorrhizal - [35]) | Sporocarp below European larch | MMN / MMN |
X.chrysenteron (XD-3 5) |
mutualistic (ectomycorrhizal - [18]) | Sporocarp below Holm oak | MMN / MMN |
T. harzianum (TH-24 3) |
hyperparasite (root parasites’ antagonist - [50]) | Organic soil layer | TME / PDB |
P. cactorum, N. radicicola, F. reticulatum and V. dahliae were isolated from fragments (1-2 mm long) of infected rootlets surface-sterilized with 0.5 % sodium hypochlorite, thoroughly rinsed with sterile water and plated on PDA ([41]). T. harzianum was isolated from 4 cm deep soil cores using 10-fold serial dilutions of soil on Trichoderma medium E (TME - [8]). A. ostoyae, L. laccata, P. arhizus, S. grevillei and X.chrysenteron pure cultures were obtained from internal tissues of young, undamaged sporocarps; A. ostoyae was plated on Potato dextrose agar (PDA), while those remaining were plated on MMNA (“modified Melin-Norkrans liquid medium” with 1.5 % agar; [23] - Tab. 2).
P. cactorum, N. radicicola, F. reticulatum, T. harzianum and V. dahliae were morphologically identified ([55], [48], [33], [56]). A. ostoyae, L. laccata, P. arhizus, S. grevillei and X. chrysenteron sporocarps were identified according to Nilson & Persson ([49]) and Bérubé & Dessureault ([2]).
Also for subsequent investigations and comparisons (see below), the identity of the fungal species was confirmed by DNA extraction, ITS PCR and sequencing. In detail, P. cactorum was identified according to Causin et al. ([10]), P. arhizus upon Henrion et al. ([29]), and all the remaining fungi according to Morris et al. ([46]). The sequences obtained were compared to those available in the NCBI (⇒ http://www.ncbi.nih.gov) and UNITE (⇒ http://unite.ut.ee) databases.
Fungal inoculum production
Each purified fungal strain was cultured in liquid medium (PDB or MMN - Tab. 2). For every strain a 1 liter flask containing 500 ml of medium was inoculated, with a blended 10-ml slurry of one 6 cm diam pure colony, previously grown on its agarized medium and maintained in an orbital shaker (50 r.p.m., 20 ± 1 °C in the dark) up to the concentration of at least 103 propagules (spores, mycelial fragments) cm-3, checked every 72 h by a Thoma hematocytometer. The mycelium from each flask was then blended for 10 s, diluted at 102 propagules in sterile water and used to inoculate six 2-liter sterile containers for each fungal species, containing 500 ml of sterile Kettering loam soil mixture (GSB loams, Kettering, Northants, UK; pH 7.7, 5.5% organic matter), moistened to 85-90% RU with sterile liquid medium, previously stored for 2 days at room temperature to allow the stabilization of the medium.
Inoculated soils were then cultured at 20 ± 1 °C in the dark. Every 72 h, the fungal viability was verified by plating fragments onto agarized medium (PDA, MMNA, TME), observing the mycelial growth. Furthermore, the fungal concentration was assessed by means of a Thoma’s hematocytometer on a mixture of five 1 cm³ sub-samples randomly collected from different portions of the container content.
For every strain, the incubation was considered complete when 3 containers reached the concentration of 1-3·103 propagules cm-3 and the remaining three 1-3·105 propagules cc-1, allowing the set up of 20 treatments (10 fungi × 2 concentrations) of 3 replicates each. The two fungal concentrations were chosen as likely average values in forest soils outside and inside the rhizosphere, according both to preliminary investigation by the authors in forest sites 1, 2 and 4 (Tab. 1), and to the scientific literature ([25], [61], [51], [57]).
Earthworm maintenance and cast collection
After incubation, whose length varied depending on the planned concentrations of fungal species (7-35 days), the content of each container was transferred to three 750 ml sterile plastic vessels suitable for earthworm culture ([38]). In each vessel a single, healthy adult of Lumbricus terrestris L., L. rubellus (Hoffmeister) or Aporrectodea caliginosa (Savigny), laboratory-bred and randomly obtained from groups producing casts lacking fungal propagules (plating casts in the 3 agarized media) was transferred and stored at 15 ± 1 °C in the dark for 13 days. These earthworm species were chosen as representatives of the 3 above-mentioned major ecological categories ([4]).
After 5, 7, 9, 11 and 13 days, each earthworm was removed from the soil, assessed, and classified as: (i) active; (ii) coiled in a resting stage (producing casts or not); or (iii) dead. Each earthworm was rinsed with distilled water, blotted dry (to remove surface soil) and then gently manipulated, such that casts were produced and the latter deposited directly into two sterile tubes: one for plating and the other one for DNA analysis. The earthworm was then returned to the given pot. When casts were not available from active or coiled earthworms, casts were defined as absent. Collected casts were stored at 5 ± 2 °C in darkness for no more than 10 days.
Fungal presence and vitality in casts
Casts from the tube for plating were used to verify the presence of the fungus previously inoculated into the soil by means of the molecular methods reported above. When the fungus was detected in at least one cast among those obtained across the whole collection period, the fungus was classified as present, otherwise it was considered absent.
Casts from the tube for DNA analysis were used to verify the vitality of the fungus present. To this purpose, all casts were singly plated in 9 cm diam Petri dishes (PDA, MMNA, TME, all treated with streptomycin sulphate 80 mg l-1 after autoclaving, to limit bacterial proliferations) and incubated at 20 ± 1 °C in the dark. Growing colonies were inspected every 2 days over a 15-day period using a compound microscope for the morphological features of the inoculated fungal species. All colonies were then isolated, purified and classified on molecular bases as previously reported, comparing the obtained sequences. When the fungal species was confirmed from at least one cast, the fungus was considered as vital; otherwise it was considered as non vital.
Results
Results showed that earthworm behavior was greatly influenced by the fungal phylum and mycelium’s concentration (Tab. 3).
Tab. 3 - Earthworm status at two fungal concentrations (103, 105 propagules cc-1 of soil) and fungus status in casts. Earthworm: (•): active; (◊): coiled in a resting stage but producing casts; (-): coiled but not producing casts; (†): dead. Fungus: (•): present and vital; (†): present and non vital; (-): absent.
Earthworm species |
Fungus Order |
Fungus species |
Earthworm status | Fungus status | ||
---|---|---|---|---|---|---|
103 | 105 | 103 | 105 | |||
L. terrestris | Oomycota | P. cactorum | • | • | • | • |
Ascomycota | N. radicicola | • | • | • | • | |
F. reticulatum | • | • | • | • | ||
T. harzianum | • | • | • | • | ||
V. dahliae | • | • | • | • | ||
Basidiomycota | A. ostoyae | • | • | † | - | |
L. laccata | • (- from day 11) | • | - | - | ||
P. arhizus | • | • (◊ from day 5) | - | - | ||
S. grevillei | • | • (- from day 11) | - | - | ||
X. chrysenteron | • | • (- from day 7) | - | - | ||
L. rubellus | Oomycota | P. cactorum | • | • († from day 9) | • | † |
Ascomycota | N. radicicola | • | • | • | • | |
F. reticulatum | • | • | • | • | ||
T. harzianum | • | • | • | • | ||
V. dahliae | • | • | • | • | ||
Basidiomycota | A. ostoyae | • | • | † | † | |
L. laccata | • | • († from day 5) | † | - | ||
P. arhizus | • | • († from day 9) | † | - | ||
S. grevillei | • | • | † | - | ||
X. chrysenteron | • | • († from day 11) | - | - | ||
A. caliginosa | Oomycota | P. cactorum | • (- from day 13) | • ( ◊ from day 5) | • | - |
Ascomycota | N. radicicola | • | • († from day 5) | • | - | |
F. reticulatum | • | • († from day 11) | • | • | ||
T. harzianum | • | - († from day 11) | • | - | ||
V. dahliae | • (◊ from day 7) | - († from day 9) | • | - | ||
Basidiomycota | A. ostoyae | • (◊ from day 11) | - | † | - | |
L. laccata | • (◊ from day 13) | - († from day 11) | † | - | ||
P. arhizus | • | • († from day 9) | † | - | ||
S. grevillei | • (◊ from day 13) | - (◊ from day 9) | • | - | ||
X. chrysenteron | • (◊ from day 13) | ◊ († from day 11) | • | - |
Lumbricus terrestris demonstrated a sharply different behavior when fed with Oomycota and Ascomycota compared with Basidiomycota. For the first group (P. cactorum, N. radicicola, F. reticulatum and V. dahliae, plant parasites and T. harzianum, their hyperparasite) at both fungal concentration and for the whole experiment period, L. terrestris maintained full fungal vitality, producing casts where the fungal species were present and vital. In contrast, when fed with fungi belonging to the Basidiomycota, only the root parasite A. ostoyae caused no adverse effects at both concentrations, even if detected in casts, also if not vital, only at the lower concentration. By comparison, when fed with all of the mutualistic Basidiomycota (L. laccata, P. arhizus, S. grevillei and X. chrysenteron), stress symptoms appeared, such as inactivity and no cast production after 5-11 days, and the fungi were never detected in casts.
Lumbricus rubellus behaved as L. terrestris for the Ascomycota, at both concentrations, and for P. cactorum at the lower concentration, but produced casts with non-vital fungus and died after 9 days when cultured with P. cactorum at the higher concentration. Furthermore, when grown with A. ostoyae and S. grevillei, stress symptoms did not appear, whilst death was detected from day 5, 9 and 11 (L. laccata, P. arhizus, X. chrysenteron, respectively) in the other treatments. Besides, none of the Basidiomycetes was found living in casts: A. ostoyae at both fungal concentrations; L. laccata, P. arhizus and S. grevillei at the lower concentration were present, but not alive; at higher concentrations the fungi were absent, as for X. chrysenteron at both concentrations.
Quite different results arose from A. caliginosa treatments, where stress symptoms never appeared when grown with N. radicicola, F. reticulatum, T. harzianum and P. arhizus at the lower concentration. In all other treatments, stress symptoms appeared (resting stages, dead, or no cast production). Regardless of the fungal presence in casts, from the lower concentration trials, P. cactorum, all the Ascomycota, S. grevillei and X. chrysenteron were present and living, while A. ostoyae, L. laccata and P. arhizus were detected but not vital. In the casts collected at the higher concentration trials, only F. reticulatum was living, while all others were not present.
Discussion and onclusion
The dispersal strategy of forest soil fungi, independent of their relationships with plants, is a key factor from a phytopathological point of view. Soil-borne fungi rarely disperse over great distances ([5]) spreading themselves by means of slow hyphal growth towards a nutritional source ([24]), but their interactions with a wide range of micro- and macro-organisms ([54], [31]) can assist passive coverage of larger distances and areas. Among these, earthworms probably more than others interact substantially with forest soil, with a feeding behavior influenced mainly by their soil exploration strategies (epigeic, endogeic, anecic). Their ability to select and feed on samples infected by a given fungal species, digesting or depositing as vital within casts is well documented ([52], [13], [12], [45], [3]), but further information is needed on earthworm involvement in the propagation of fungi involved in forest plant health, with increasing distance from the plant.
The experiment performed confirmed ([45], [60]) that different earthworm species can feed on different fungi, with total digestion, or their release with at least partial vitality in casts, with differences in this ability mainly due to the fungal phylum and fungal concentration. Results obtained here were partially comparable with those of Bonkowski et al. ([3]), where pathogenic fungi were preferred over Trichoderma, but in our experiment the species used were different. By comparison, when fed with Basidiomycota the earthworms showed a species-specific behavior. Lumbricus terrestris produced casts containing dead hyphae of A. ostoyae, typically a root rotter fungus, while no DNA traces of all other Basidiomycota (all ectomycorrhizal) were found in casts. Lumbricus rubellus demonstrated an ability to feed on all of the Basidiomycota given, excluding the mycorrhizal X. chrysenteron, but these were never found as vital in casts. A. caliginosa fed on all of the Basidiomycota, producing casts with dead propagules of A. ostoyae, L. laccata and P. arhizus, and living propagules of S. grevillei and X. chrysenteron.
The destiny of ingested microorganisms depends on their adaptation to the intestinal conditions of the earthworm ([15], [44], [45], [6]). Two opposing processes act during digestion. Favorable pH-value, increased nutrient and water supply in the gut increases the microbial population during gut passage ([1]), whilst intestinal transit and fluids can reduce numbers of species by digestion ([16]). According to these results, our study showed that all earthworms allowed a safe transit of all the non-Basidiomycetes and that the parasitic Basidiomycete A. ostoyae was fed upon and totally digested. Moreover, the remaining Basidiomycetes, all mycorrhizal, ranged from rejection (L. terrestris) to ingestion (A. caliginosa). Moreover, in the latter a total digestion of some mycorrhizal Basidiomycete (L. laccata, P. arhizus) and a lack or incomplete digestion of others (S. grevillei and X. chrysenteron) was observed.
As earthworms prefer the habitats in which they forage ([54], [19]), considering 7 days is enough both to feed and, for L. terrestris, to potentially move several meters away from the point of grazing ([40]), we might suppose that all the earthworms tested can spread the given Oomycota and the Ascomycetes (1 antagonist, 4 root parasites). Furthermore, A. caliginosa can act as a vector of 2 mutualistic fungi. Observed stress symptoms of this earthworm species, likely attributable to mycotoxic effects ([62]), need further investigation.
In contrast with Moody et al. ([44]), under our experimental conditions no differences in earthworm preference were observed among Ascomycetes, apart from A. caliginosa that, at the highest concentration, exclusively preferred F. reticulatum.
In summary, the non-Basidiomycetes, frequently quickly colonizing the superficial soil layers when present in low concentrations (as typically happens in bulk soil), are easily fed upon and transported alive to other ecologically similar sites by earthworms. This resulted, moreover, independently from the behavior (relationship with plants) of the latter. Increasing the fungal concentration, as usually happens moving from bulk soil to rhizosphere, this ability characterizes mainly epigeic and anecic species ([3]). As the Oomycetes studied were restricted to just one species, such wide considerations can be only speculative, but earthworms may act as with the Ascomycetes. The Basidiomycetes (including the root parasite A. ostoyae and 4 ectomycorrhizal fungi) were not fed upon, or were eaten and totally digested, probably due to their low food quality for earthworms ([3]). Among them, as a general trend, ectomycorrhizal fungi were detected alive in casts only in the pairs A. caliginosa + S. grevillei and X. chrysenteron: often they were not eaten (L. terrestris in all concentrations, L. rubellus and A. caliginosa in the highest concentration), sometimes they were eaten and totally digested. Unfortunately, little is known to explain these results as, unlike endomycorrhizae ([26]), zootrophic dispersal of ectomycorrhizae has been widely documented only with small mammals ([68], [34], [54]). Generally, the reported results confirmed that earthworms have an important role in spreading soil fungi in forests, and that such activity can depend on both the ecological grouping of the three species involved, and the fungal concentration, widening the knowledge on the ecologic dynamics related to forest plants’ health within the soil. Fast-growing species were preferred to Basidiomycetes, generally refused, with a general food preference irrespective of earthworm ecological group, visible only when associated to the rhizospheric fungal concentrations. This allowed us to suggest that fungi characteristic of early successional stages of decomposition can be used by earthworms as cues to detect fresh and nutrient rich organic resources in soil. In accordance with previous papers ([58], [59], [66], [3]), considering the ecological role of fungi as part of the plant, decomposer community may provide a deeper insight into the underlying mechanisms, than simply referring to food preferences of earthworms. This hypothesis is supported by the observed food preferences of earthworms: the most preferred fungi of earthworms include many plant tissue parasites which commonly attack either plantlets and adult plants (Phytophthora, Fusarium, Verticillium, Nectria). The selectivity for fungal species differed considerably among earthworm species in our experiment, indicating differential use of fungi as food or food indicators by earthworms. Detritivore epigeic and anecic earthworm species, L. rubellus and L. terrestris, respectively, are important consumers of litter material which is generally densely colonized by fungi. These species have been shown to be more selective in their food choices ([28]), and the distinctive preferences for certain fungal species by the epigeic L. rubellus and anecic L. terrestris are in accordance with our expectations. In contrast, the geophagous endogeic species A. caliginosa was highly selective (only Fusarium reticulatum at rhizospheric concentration) and consumed less material. Endogeic species consume high amounts of mineral soil ([32]) and rely less on fresh litter resources.
A general trend can be proposed: outside of the rhizosphere or during secondary successions (e.g., after fires), propagules from surviving vegetation can be more readily moved into new areas, being recolonized by plants than could be achieved via physical dispersal alone. Where low fungal concentration is common, non-Basidiomycetes have greater opportunities than other fungi to be spread by earthworms. This could explain the capillary presence of Fusarium and Verticillium infections, also in seedlings, along an ecotonal forest border, probably also passively vectored by earthworms or other soil fauna.
As the effects of any competition in species assembly is difficult to demonstrate ([11]), a combined use of experimentation and mathematical modeling could be useful. Further investigation and results are needed to analyze the numerous abiotic and biotic variables dynamically interacting in the rhizosphere and having a role in plant diseases epidemiology.
Acknowledgements
The authors wish to thank Dr. M. Stefenatti and Mr. S. Zanella for their help in fungal isolations and molecular analyses.
References
Gscholar
Gscholar
Gscholar
Gscholar
Gscholar
Gscholar
Gscholar
Gscholar
Gscholar
Gscholar
Gscholar
Gscholar
Gscholar
Gscholar
Gscholar
Gscholar
Authors’ Info
Authors’ Affiliation
Linda Scattolin
TeSAF Department, University of Padova, v.le dell’Università 16, I-35020 Legnaro (PD, Italy)
DAFNAE Department, University of Padova, v.le dell’Università 16, I-35020 Legnaro (PD, Italy)
School of Built and Natural Environment, University of Central Lancashire, Preston PR1 2HE (United Kingdom)
Corresponding author
Paper Info
Citation
Montecchio L, Scattolin L, Squartini A, Butt KR (2015). Potential spread of forest soil-borne fungi through earthworm consumption and casting. iForest 8: 295-301. - doi: 10.3832/ifor1217-008
Academic Editor
Alberto Santini
Paper history
Received: Dec 26, 2013
Accepted: May 24, 2014
First online: Aug 26, 2014
Publication Date: Jun 01, 2015
Publication Time: 3.13 months
Copyright Information
© SISEF - The Italian Society of Silviculture and Forest Ecology 2015
Open Access
This article is distributed under the terms of the Creative Commons Attribution-Non Commercial 4.0 International (https://creativecommons.org/licenses/by-nc/4.0/), which permits unrestricted use, distribution, and reproduction in any medium, provided you give appropriate credit to the original author(s) and the source, provide a link to the Creative Commons license, and indicate if changes were made.
Web Metrics
Breakdown by View Type
Article Usage
Total Article Views: 46850
(from publication date up to now)
Breakdown by View Type
HTML Page Views: 38945
Abstract Page Views: 2828
PDF Downloads: 3795
Citation/Reference Downloads: 57
XML Downloads: 1225
Web Metrics
Days since publication: 3623
Overall contacts: 46850
Avg. contacts per week: 90.52
Article Citations
Article citations are based on data periodically collected from the Clarivate Web of Science web site
(last update: Feb 2023)
Total number of cites (since 2015): 7
Average cites per year: 0.78
Publication Metrics
by Dimensions ©
Articles citing this article
List of the papers citing this article based on CrossRef Cited-by.
Related Contents
iForest Similar Articles
Review Papers
Soil fungal communities across land use types
vol. 13, pp. 548-558 (online: 23 November 2020)
Research Articles
Soil fauna communities and microbial activities response to litter and soil properties under degraded and restored forests of Hyrcania
vol. 14, pp. 490-498 (online: 11 November 2021)
Research Articles
Fungal and bacterial communities in a forest relict of Pinus pseudostrobus var. coatepecensis
vol. 16, pp. 299-306 (online: 09 November 2023)
Research Articles
Soil stoichiometry modulates effects of shrub encroachment on soil carbon concentration and stock in a subalpine grassland
vol. 13, pp. 65-72 (online: 07 February 2020)
Research Articles
Effect of plant species on P cycle-related microorganisms associated with litter decomposition and P soil availability: implications for agroforestry management
vol. 9, pp. 294-302 (online: 05 October 2015)
Research Articles
Seasonal dynamics of soil respiration and nitrification in three subtropical plantations in southern China
vol. 9, pp. 813-821 (online: 29 May 2016)
Short Communications
Culturable fungi associated with wood decay of Picea abies in subalpine forest soils: a field-mesocosm case study
vol. 11, pp. 781-785 (online: 28 November 2018)
Research Articles
Wood-soil interactions in soil bioengineering slope stabilization works
vol. 2, pp. 187-191 (online: 15 October 2009)
Research Articles
Soil respiration along an altitudinal gradient in a subalpine secondary forest in China
vol. 8, pp. 526-532 (online: 01 December 2014)
Research Articles
Effects of arbuscular mycorrhizal fungi on microbial activity and nutrient release are sensitive to acid deposition during litter decomposition in a subtropical Cinnamomum camphora forest
vol. 16, pp. 314-324 (online: 13 November 2023)
iForest Database Search
Search By Author
Search By Keyword
Google Scholar Search
Citing Articles
Search By Author
Search By Keywords
PubMed Search
Search By Author
Search By Keyword