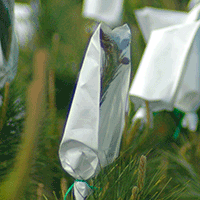
Prospects for evolution in European tree breeding
iForest - Biogeosciences and Forestry, Volume 17, Issue 2, Pages 45-58 (2024)
doi: https://doi.org/10.3832/ifor4544-017
Published: Mar 06, 2024 - Copyright © 2024 SISEF
Review Papers
Abstract
Genetically improved forest reproductive materials are now widely accessible in many European countries due to decades of continuous breeding efforts. Tree breeding does not only contribute to higher-value end products but allows an increase in the rate of carbon capture and sequestration, helping to mitigate the effects of climate change. The usefulness of breeding programmes depends on (i) the relevance of the set of selected traits and their relative weights (growth, drought tolerance, phenology, etc.); (ii) the explicit management of targeted and “neutral” diversity; (iii) the genetic gain achieved; and (iv) the efficiency of transferring diversity and gain to the plantation. Several biological factors limit both operational breeding and mass reproduction. To fully realise the potential of tree breeding, the introduction of new technologies and concepts is pivotal for overcoming these constraints. We reviewed several European breeding programmes, examining their current status and factors that are likely to influence tree breeding in the coming decades. The synthesis was based on case studies developed for the European Union-funded B4EST project, which focused on eight economically important tree species with breeding histories and intensities ranging from low-input breeding (stone pine, Douglas-fir and ash) to more complex programmes (eucalyptus, maritime pine, Norway spruce, poplar, and Scots pine). Tree breeding for these species is managed in a variety of ways due to differences in species’ biology, breeding objectives, and economic value. Most programmes are managed by governmental institutes with full or partial public support because of the relatively late return on investment. Eucalyptus is the only tree species whose breeding is entirely sponsored and managed by a private company. Several new technologies have emerged for both phenotyping and genotyping. They have the potential to speed up breeding processes and make genetic evaluations more accurate, thereby reducing costs and increasing genetic gains per unit of time. In addition, genotyping has allowed the explicit control of genetic diversity in selected populations with great precision. The continuing advances in tree genomics are expected to revolutionise tree breeding by moving it towards genomic-based selection, a perspective that requires new types of skills that are not always available in the institutions hosting the programmes. We therefore recognise the importance of promoting coordination and collaboration between the many groups involved in breeding. Climate change is expected to bring in new pests and diseases and increase the frequency of extreme weather events such as late frosts and prolonged droughts. Such stresses will cause slow growth and mortality, reducing forest productivity and resilience. Most of these threats are difficult to predict, and the time-consuming nature of conventional breeding does not allow for an adequate and timely reaction. We anticipate that most breeding programmes will need to revise their selection criteria and objectives to place greater emphasis on adaptive performance, tolerance to multiple environmental stresses, stability in different environments, and conservation of genetic diversity. Testing breeding materials in a variety of environments, including potentially contrasting climates, will become increasingly important. Climate change may also force the incorporation of new genetic resources that provide new useful adaptations, which may involve the use of new, previously unexplored gene pools or hybridisation, with the enormous challenge of incorporating useful alleles without adding along an unfavourable genetic background. Decision-support tools to help landowners and foresters select the best-performing forest reproductive material in each specific environment could also help reduce the impact of climate change.
Keywords
Tree Breeding, Breeding Programmes, Breeding Strategies, Climate Change, Seed Orchards, Genomic Selection
Introduction
Forests provide a range of ecosystem services to society, most importantly ensuring the renewable raw materials for a wide range of manufactured products, including pulp, paper, cork, resins, fuel, and construction timber, which replace fossil-based carbon products. In addition, forests are efficient carbon sinks that mitigate the effects of climate change ([111]). Between 1990 and 2020, the global forest area decreased by 420 million hectares ([47]). Despite a slight increase in European forest area, the global conversion of forests to agriculture and infrastructure is increasing pressure on production in cultivated forests and on the conservation of wild forests. It is expected that plantation forests, which can incorporate the benefits of genetic improvement and conservation, can play an important role in minimising the demand for wild resources. Both European forest owners and forest managers agree that one of the strategies to be favoured in the face of climate change is the use of improved Forest Reproductive Material (FRM) in plantations and for the enrichment of natural regeneration zones ([120]). Forest tree breeding aims to improve the genetic qualities of FRM to increase the quantity and quality of harvested forest products and make forests more resilient to global change. In Europe, breeding programmes have been carried out for decades in several economically important tree species, resulting in significant genetic gains ([76]), even maintaining the genetic variability of improved FRM close to the level of the original natural stands ([106], [110]).
Many forest trees have biological characteristics such as a long lifespan, late fertility, and difficult sexual or vegetative propagation that are not conducive to smooth breeding progress. As a result, tree breeding is a slow process compared to crop and livestock breeding, but the gain in each generation can still be relatively high. Tree breeders have already experimented with various tools and technologies to alleviate the major biological constraints in the hope of reducing the cost of breeding activities, increasing the pace of breeding, and increasing genetic gains. Some of these, such as flowering promotion by plant hormones or vegetative propagation by top-grafting ([38]), rooted cuttings, or somatic embryogenesis ([91]), have proved to be operationally feasible, while others, such as genetic engineering leading to genetically modified plants, have all but disappeared from the discussion. It has now become clear that most technologies require a long period of development before they are ready for large-scale breeding and that their application also depends on the context of the breeding programme. For example, the first DNA markers appeared in the 1980s, but it took decades for them to find their way into mainstream breeding programmes. More recently, advancements in high-throughput DNA sequencing and the introduction of Single Nucleotide Polymorphisms (SNPs) have greatly expanded the range of potential applications in tree breeding ([71]).
This article first presents the current status of forest tree breeding programmes in Europe through their organisation, the breeding objectives and the strategies considered for selection and deployment of improved FRM. Then a transversal analysis of potential developments that could soon influence the way tree breeding is practised in Europe and worldwide is detailed. The technological innovations examined cover those that reduce breeding costs by facilitating the collection and analysis of large amounts of phenotypic data; and those that take advantage of genomic knowledge and can be used to replace or supplement phenotyping data with information from SNP markers to improve the accuracy of genetic evaluation and speed up the breeding process. We also examine new challenges for tree breeding in the context of climate change, the potential barriers to the production of different FRM types, the breeding objectives and their future evolution, and the key players in the current tree breeding efforts taking place in Europe.
Our synthesis owes a great deal to the species-specific case studies acquired as part of the “B4EST” H2020 research project ([15]), funded by the European Union and involving 21 partners from universities, research organizations, and companies. These provide a more in-depth analysis for the breeding of eucalyptus ([89]), maritime pine ([3], [20]), Mediterranean stone pine ([108]), Norway spruce ([140]), poplar ([17]), and Scots pine ([58]).
Current status of European tree breeding
Breeding programmes and their organisation
The breeding programmes included in the current analysis are listed in Tab. 1. Most of them were initiated in the second half of the twentieth century. They represent different levels of complexity and progress, from basic breeding programmes with a limited number of trials (stone pine and ash) to more advanced ones (eucalyptus, maritime pine, Douglas-fir, Norway spruce, poplar, and Scots pine).
Tab. 1 - Main features of the European forest tree breeding programmes reviewed. SYEAR: starting year; GEN: the most advanced generation with selected trees; TOTHA: total accumulated trial area (ha); AVGHA: Average trial area established per year (ha).
Tree species | Country | SYEAR | GEN | TOTHA | AVGHA |
---|---|---|---|---|---|
Maritime pine | France | 1960 | 3 | 400-600 | 6-10 |
Spain-Galicia | 1998 | 1 | 10 | 1.5 | |
Spain-Central | 1990 | 1 | 100 | 3 | |
Stone pine | Spain | 1989 | 1 | 20 | <1 |
Scots pine | Finland | 1947 | 2 | 2250 | 3 |
Sweden | 1940-1950 | 3 | 900 | 10-12 | |
Norway | 1947, 2020 | 1 | 10 | 5 | |
Poplar | Italy | 1980 | 2 | 280-330 | 8-10 |
Norway spruce | Finland | 1947 | 2 | 420 | 8 |
Sweden | 1940-1950 | 2 | 800 | 10 | |
Norway | 1947 | 2 | 225 | 8 | |
Eucalyptus | Portugal | 1964 | 3 | 250 | 2 |
Ash | Netherlands | 1960-1970 | 1 | 9 | 0 |
France | 1985 | 1 | 45 | 1 | |
Douglas-fir | France | 1985 | 2 | 200 | 4 |
The organisation of the tree breeding programmes considered here varies; some are run entirely by for-profit companies, while others are fully linked to the public sector. However, the general trend is that tree breeding in Europe is largely supported by public funds.
Maritime pine breeding activities in Spain are publicly funded and run by regional institutions in the regions where maritime pine grows. The French maritime pine breeding programme also relies mainly on public funding. It is managed by two institutions (INRAE, the National Research Institute for Agriculture, Food, and Environment, and FCBA, the technological institute for wood and forest sector) and coordinated by a common structure (GIS “Groupe Pin Maritime du Futur”). A similar scheme exists for Douglas-fir in France, involving three main players: INRAE, FCBA, ONF, and also coordinated through a GIS-like board, which is regularly funded by the Ministry. In the Netherlands, Wageningen Research, a non-profit research organisation, is the main actor engaged in ash tree improvement, with the Ministry of Agriculture, Nature, and Food Quality providing the majority of financing. Same in France with INRAE, and the Ministry of Agriculture. The Scots pine and Norway spruce breeding programmes in Finland are funded by the government and managed by a public research institute (Luke). The Norwegian breeding programmes of these two species are managed by the non-profit foundation Skogfrøverket (Norwegian Forest Seed Center), which is supported by 60 percent public money and 40 percent seed sales revenue. Stone pine breeding in Spain has been the result of isolated initiatives by forest administrations in different autonomous regions of the country and by the Ministry for Ecological Transition, supported by collaborations with several universities and the national research institute ICIFOR-INIA (CSIC).
The two eucalyptus breeding programmes in Portugal, run by pulp and paper companies Altri and The Navigator Company, are the only examples of private-sector breeding, among the examples cited here. Cases of Norway spruce in Sweden, stone pine in Portugal, and poplar in Italy fall into the middle ground. In Sweden, Skogforsk, which is a private foundation with a mix of public and private support, runs the breeding programmes of Norway spruce and Scots pine and provides improved breeding material for private enterprises that manage seed orchards of these species. In Portugal, the national research institute INIAV oversees stone pine breeding and receives funding from associations of large private landowners, whereas regional private forest owners’ associations own and manage mother-tree orchards (clonal gardens used to collect scions for grafting). In Italy, poplar breeding projects are independently run by the public organisation CREA and private enterprises, with no stable collaboration between the public and private research communities.
The profitability of breeding activities heavily influences the mode of organisation ([49]). There are many factors that contribute to a generally low rate of return on investment, such as the long time between the start of breeding and the first releases of improved FRM and the resources required (time, money, land, and personnel). The market size (annual regeneration area) for some tree species may be modest. Finally, landowners’ reluctance to pay more for better materials may further discourage private investments in breeding. A larger investment in upstream activities includes the sizable investments made by the forest industry for conifer breeding in Sweden and eucalyptus in Portugal. Mills are substantial investments that require a steady supply of wood in sufficient quantities. Breeding, as well as seed and seedling production, will increase the supply of raw materials for this purpose, encouraging superior varieties to be planted in both company-owned and privately held forest areas.
The operative deployment and production of improved FRM consist of the establishment, maintenance, and harvesting of seed orchards, as well as the production of seedlings and clonal plants in nurseries. Even in situations where breeding operations receive only public funding, private businesses typically manage these commercial activities. More details on the type of FRM deployed for each tree species are reported below.
Breeding objectives and selected traits
As most forest trees have long commercial rotations, breeding objectives are usually rather general to ensure that they are valid in a range of future scenarios, including those involving changing climate and evolving industrial processes and demands. Most breeding programmes aim to increase the quantity and quality of harvested wood, but for a few species, there are other objectives such as resin supply (maritime pine in Spain) and cone production (Mediterranean stone pine).
The main goal of breeding Scots pine and Norway spruce is to increase wood production and the economic value of end-products ([125], [126], [76], [133]). As these species are commonly used for sawn timber, it is important to achieve good stem quality (few knots, straight stems and no defects). For Norway spruce in particular, the economic value of wood is strongly linked to structural uses. In addition to eliminating trees with stem defects, more attention is being paid to eliminating early flushing families that are susceptible to spring frost, as well as to increasing stiffness, reducing grain angle, and maintaining wood density ([140]).
Eucalyptus breeding goals have been aligned with the demands of pulp and paper companies, with trees selected for growth, wood density, and wood cellulose content ([89]). In case of poplar, breeding mainly aims at improving vegetative propagation capacity, growth vigour, stem form, and wood quality ([17]). Stone pine, traditionally used for land reclamation and forest restoration, has gained attention for producing highly prized edible pine nut kernels, and therefore the main objective of breeding is now improved cone and kernel production ([110]). With respect to ash, breeding was primarily aimed at improving the growth and wood quality features, such as stem straightness, lack of forks, and fine branching ([113]), but recently the focus has shifted to tolerance to the devastating ash dieback disease ([153]). Douglas-fir is appreciated mostly for structural timber. First selections across European breeding programs were made with growth and general tree architecture being the most important traits, to obtain important volumes with as little defaults as possible in order to keep its good baseline mechanical properties ([10]).
Breeding goals have frequently evolved over time. Early in the breeding programme of maritime pine, plus trees were selected for growth and stem straightness. While these traits remain essential selection criteria, wood density, branch quality, and twisting-rust resistance were later added to the list of selection criteria. A similar trend has occurred in Douglas-fir breeding in France, which currently prioritises phenology, architecture, branching patterns, and wood density over growth ([9]). In Nordic breeding programmes of Norway spruce and Scots pine, increased adaptive performance and phenotypic plasticity across environments ([36], [135]) are now given more attention.
Resistance and tolerance to biotic and abiotic stresses are frequently associated with potential breeding goals related to wood production. Resistance to pathogens and pests includes root rot (Heterobasidion spp.) in Norway spruce ([145], [139], [33]), Fusarium circinatum and nematode in maritime pine ([3]), Phoracantha semipunctata, Gonipterus platensis and fungi from genus Mycosphaerella in eucalyptus ([89]), ash dieback in ash ([104]), Phloeomyzus passerinni, Melampsora spp., and Marsonnina brunnea in poplar ([40], [29], [52], [34]).
Drought tolerance is a major breeding target in eucalyptus to increase its resilience ([89]), but there is growing interest in breeding for this trait in other species such as maritime pine ([112]), poplar ([124]) and Norway spruce ([63]). The lack of practical and effective methods for phenotyping is currently considered as a major bottleneck for the integration of abiotic stress resilience traits in breeding programmes.
Breeding strategies
Tree breeding customarily starts with the selection of phenotypically superior trees in natural stands as candidates. Following that, breeding advances to (i) the genetic evaluation by means of progeny testing; (ii) the selection of superior candidates for their breeding value; and (iii) the production of the next-generation recruitment population using the selected candidates as parents. At this stage, the top candidates are also deployed as seed orchard parents or in vegetative mass propagation. Beyond these general features, the precise format in which any breeding programme is carried out varies greatly ([113]). The structuring of breeding materials, for instance, may vary from a single to multiple populations. Such population division could be motivated by the need to cover diversifying breeding goals, for conservation purposes, or the ability to provide unrelated parents to production populations ([59]). In regions such as northern Europe, where there is a wide range of climatic conditions, breeding materials are organised into smaller subpopulations, each of which is bred for a specific climate. Sometimes these subpopulations may have different testing and selection strategies, as in the case of Scots pine breeding in Sweden ([58]).
Phenotypic data gathered from progeny tests and pedigree information forms the basis of selection among candidates. Mixed-model methodology is now commonly used to produce robust BLUP (Best Linear Unbiased Prediction) calculations that take into account the information from a large number of trials connected through tree relatedness ([24]) or accommodate spatial adjustments of environmental heterogeneity ([28]) for the general improvement of selection accuracy. Sometimes, selection happens at subsequent steps for operational reasons. This is the case of Norway spruce, where preliminary selection for the most promising candidates in the field may come at an age of around 7 years before the genetic testing in the field is finalised at ages higher than 12 ([140]). Considering poplar, selection is also done in multiple stages, with distinct emphasis placed on different sets of traits at each stage. The candidates selected at each stage are cloned and evaluated more precisely based on data from many genetically identical copies in the subsequent steps ([138], [114]). Vegetative propagation (rooted cuttings) is also employed for field testing of Norway spruce on multiple sites in Sweden, Norway, and Finland to improve the accuracy of genetic evaluation ([82], [141]) and to get more information about the phenotypic plasticity of candidates ([83], [140]).
Breeding programmes use different methods to develop new recruitment populations depending on their biological and financial constraints. Most programmes rely on bi-parental crosses, but polycrosses ([23]) and open pollination, followed by paternity recovery or relationship analysis from genomic data can also be used. The time required for trees to reach reproductive maturity as well as uneven cone setting are considered major impediments to making fast breeding progress in species like Norway spruce and Scots pine ([58], [140]).
In the breeding programmes under consideration, the use of DNA markers has been confined to quality control (recognition of the real genotypic identity of trees - [6]). Several pilot studies are underway, however, to use DNA markers to enhance selection within a marker assisted selection or genomic selection scheme for some of the species considered here. These are pilot studies because the schemes are implemented on a reduced scale, involving a relatively small part of the whole breeding population and testing networks. Some of the most advanced cases are those in Norway spruce ([32], [35]), maritime pine ([72]), poplar ([114], [17]) and eucalyptus ([62]). In other species, like in ash, ongoing research aims at developing DNA markers for species and genotype recognition (Dowkiw A., pers. comm.) and ash dieback resistance ([142], [31]), or in Pinus pinea to test the application of genomic prediction ([110]).
Deployment of improved FRM
The impact of tree breeding is determined by the scale at which improved FRM is used in forest regeneration. Tab. 2 summarises the types of FRM used as well as the magnitude of the current deployment by species and countries, showing that genetically improved FRM represents a significant portion of the materials accessible in Europe today.
Tab. 2 - FRM and their current deployment European countries.
Tree species | Country | Type of FRM | Seedling/clonal plants produced per year |
Share of improved FRM |
---|---|---|---|---|
Maritime pine | France | Seedlings | 37M | >95% |
Spain - Galicia | Seedlings | 20K | <5% | |
Spain - Central | Seedlings | 0.5M | <15% | |
Stone pine | Spain | Clonal plants | <1K | <1% |
Portugal | Clonal plants | >10K | <5% | |
Scots pine | Finland | Seedlings / seeds | 40M (seedlings) | 96% |
Sweden | Seedlings | 236M | 98% | |
Norway | Seedlings | 2M | 99% | |
Poplar | Italy | Clonal plants | 140K-180K | 10-15% |
France | Clonal plants | 900K | 40-50% | |
Norway spruce | Finland | Seedlings | 75M | 70% |
Sweden | Seedlings | 197M | 71% | |
Norway | Seedlings | 50M | 95% | |
Ash | Netherlands | Seedlings | < 4K | 73% |
Eucalyptus | Portugal | Clonal plants / seedlings | 16 M | 58% |
Douglas-fir | France | Seedlings/seeds | 12M (seedlings) | 100% |
Improved FRM is produced by means of vegetative and sexual propagation. Seedlings produced from open-pollinated seed orchards are the dominant type of FRM since they are cheaper to produce than vegetatively propagated plants. Nurseries use seed from seed orchards to grow seedlings, but in some countries, orchard-reproduced seeds are also directly used for regeneration (for the direct seeding of Scots pine, see [58]). Seed orchards fall into two categories: clonal seed orchards, which consist of grafted propagules of multiple (often more than 20) selected trees, and seedling seed orchards, which consist of offspring (families) of selected parents. Seedling seed orchards have been established in Norway spruce, Scots pine, maritime pine, and eucalyptus.
Clonal plants are currently produced by means of rooted shoot cuttings (eucalyptus and poplar), rooted cuttings (Norway spruce), grafting (stone pine, Douglas-fir), or somatic tissues multiplied and manipulated in vitro (eucalyptus). In eucalyptus, the main limitation of vegetative propagation is the rooting ability, which greatly varies among eucalyptus species and genotypes, with reproduction by means of seed orchards being the only option for propagating rooting recalcitrant genotypes ([89]). Production facilities for somatic embryogenesis plants of Norway spruce are under implementation in Sweden and Finland.
Being generally based on more intensively selected material, clonal plants have the potential to be more productive than orchard-reproduced seedlings. The vegetatively propagated materials are usually progenies from intra-specific crosses or, as is the case for poplar (P. deltoides × P. nigra), interspecific crosses ([18]). Controlled-crossed materials are free of the high quantities of pollen contamination that can cause significant genetic losses in open-pollinated seed orchards ([66]). As a further advantage, vegetative propagation avoids the delay in the onset of seed production after selection that we experience with seed orchards.
The constraints in FRM production are often related to production capacity, and adjusting them takes some time, especially in the case of conifer seed orchards, which show at least an 8- to 10-year lag from the establishment to the first harvest. Although occasional seed shortages could be avoided by increasing production capacity with regular turnover, this involves substantial investment that is rarely compensated by moderately priced seed sales.
Drivers of evolution in tree breeding
Technological progress
Phenotyping tools
The collection of large quantities of phenotypic data on an increasing range of traits and environments for genetic evaluation is both expensive and time-consuming. In addition, the reality and prospects of climate change have also given rise to the need to set up assessments in contrasting environments or complete gradients, in order to measure the plasticity and resilience of FRM, which in turn generates new phenotyping needs. Given the financial constraints of breeding, there is a need for more effective phenotyping methods that can increase the number of trees assessed, enabling more intense selection and higher genetic gain. It is difficult to summarise the diverse technological advancements impacting phenotyping, but most of them fall into two categories: (i) robotic vehicles, which are the platforms for automated sensing assessments; (ii) technologies providing faster proxies for traditional assessments.
Unmanned aerial vehicles (UAVs) are the most prominent examples of the first category and are among the most promising pieces of equipment for phenotyping since they may be used for high-resolution aerial photography (3D photogrammetry) or light detection and ranging scanning (LiDAR). These techniques may be used to assess cone and pollen production in seed orchards or clonal archives. In field trials, they are now replacing telescopic poles or Vertex for measuring tree heights in many situations, particularly after canopy closure in stands taller than 10-12 metres. This approach is now being evaluated in maritime pine and is being evaluated and implemented in Norway spruce ([137]) and Scots pine ([134]). In poplar, UAV-based thermal imaging was used to assess genotype variability under drought stress conditions, with promising results ([97]). Currently, an UAV has been used, in conjunction with a UAV-born sawing system, to gather twigs (for grafting), needles (for DNA extraction), and cone samples from Norway spruce and Scots pine trees in Norway ([134]). LIDAR has rapidly become a way to gather information on tree attributes that are challenging to assess using conventional methods, like stem form, tree crown volume, and leaf area index, although the efforts needed for data processing are still limiting its application in breeding. The use of image-based phenotyping could have been even more disruptive, as reviewed by Bian et al. ([16]): it provides a means to precisely quantify complex traits that were only scored rudimentarily or even not considered until now.
The technological advancements providing faster proxies can be illustrated by two examples revealing the many technical revolutions to come: the resistograph tool and the use of Near-InfraRed Spectroscopy (NIRS). The resistograph is a device initially designed for structural assessments on wooden constructions, which can easily replace in the field the X-ray measurements based on increment cores in most species. It evaluates wood density precisely and quickly, while simultaneously providing information on annual radial growth ([70], [21], [50], [73]). Although many other non-destructive evaluation methodologies for wood properties have been reviewed by Schimleck et al. ([128]), the use of resistograph appears to be the most appropriate for quickly evaluating a large number of trees and is now largely used by forest tree breeders. The second example is based on the use of NIRS which is widely used for phenotyping in plants and animals ([1], [25]). NIRS has been applied in forest trees for evaluation of wood lignin properties, wood density, as well as other wood properties such as extractives ([5], [127], [131]) or even for forecasting the susceptibility of ash to biotic stressors like chalara ([157]). The term “phenomic selection” was coined by Rincent et al. ([118]) to describe the use of NIRS as a high-throughput, inexpensive, and non-destructive technology to indirectly record endophenotypic variations and calculate relationship matrices in Populus nigra. This method provides intriguing new insights into characterising trees in various environments, enhancing selection and exploiting greater genetic diversity. Phenomic selection proof-of-concepts must be developed to better assess its potential for breeding in other forest tree species besides poplar.
Genotyping tools
SNP arrays are generally preferred over genotyping-by-sequencing techniques for high-throughput genotyping in breeding because of their excellent repeatability and simpler raw data processing, but the investments required for their design limit their development. Furthermore, they can be biased towards the specific germplasm used in the SNP discovery step ([8]). However, they have been widely used in plant genetic applications, even for forest trees (Tab. 3).
Tab. 3 - High-throughput genotyping tools available per tree species.
Tree species | Genotyping tool | Markers | Reference |
---|---|---|---|
Scots pine | Thermo Fisher Axiom PiSy50k array | 50K SNPs | [84] |
Norway spruce | Thermo Fisher Axiom Pcab50K array | 50K SNPs | [14] |
Poplar | Illumina ISelect Infinium | 34K SNPs | [53] |
Infinium | 12K SNPs | [46] | |
Thermo Fisher Axiom 4TREE array | 12K SNP | [57] | |
Maritime pine | Illumina Infinium | 12K SNPs | [30] |
Illumina Infinium | 9K SNPs | [115] | |
Thermo Fisher Axiom 4TREE array | 12.5K SNPs | [57] | |
Stone pine | Thermo Fisher Axiom 4TREE array | 5.7K SNPs | [110] |
Eucalyptus | Thermo Fisher Axiom Euc72K | 14.7K SNPs | [62] |
Ash | Thermo Fisher Axiom 4TREE array | 13.4K SNPs | [57] |
Douglas-fir | Thermo Fisher Axiom PN550607 | 50K SNPs | [67] |
In particular, as a result of the B4EST initiative, a commercial multi-species 4TREE array was designed with 50K SNPs for Populus sp., Fraxinus sp., Pinus pinaster, and Pinus pinea ([6], [57]). Two other arrays were also generated within the same project: one for Scots pine ([84]) and one for Norway spruce ([14]). All these genotyping tools must be associated with genotyping platforms that ensure an efficient and robust protocol for sample collection, DNA extraction, genotyping, and biobank storage, as developed in Nordic countries for Scots pine, for example.
Molecular markers are particularly useful for genotypic identification and pedigree correction. This means eliminating labelling and grafting errors, and guaranteeing that the correct genotypes are utilised for controlled crossings, clonal archives, and seed orchards. In France, for instance, a low-density SNP array (62 markers - [155]) was optimised for identity and parentage analyses in maritime pine. The results showed that about 10% of the grafted plants in clonal archives were affected by pedigree errors. Each new selected tree is now genotyped for identity control before grafting to clonal archives. Furthermore, pedigree corrections enable a more precise BLUP evaluation and higher genetic gains, although the widespread adoption of molecular markers for this purpose is still in its early stages ([155], [85]).
The use of DNA markers to reconstruct pedigrees allows for less costly breeding strategies. One such potential strategy is to replace bi-parental controlled crosses with polymix breeding followed by pedigree reconstruction ([71], [88]). The main advantage of this strategy is its ability to generate a high number of families with a low number of pollination operations, making it particularly attractive for breeding programmes with limited resources to implement conventional breeding schemes. It has been evaluated in the context of the French maritime pine breeding programme ([23]) and more recently with attention to the cost of different breeding operations (B4EST deliverable D5.2). Two analogous strategies, known as “Breeding without Breeding” (BwB - [43]) and “quasi-field trial” have been successfully tested in the breeding of European larch (Larix decidua - [95]) and Nordmann fir (Abies Nordmanniana - [60]), respectively. In these cases, the concept was proven when planted stands were treated as ad-hoc progeny trials using DNA markers to fingerprint and reconstruct the pedigree of a population and candidates for phenotypic forward selection. The basic concept is to skip the initial steps of plus-tree selection in wild stands and establishment of progeny trials, and instead conduct the initial selections in commercial stands that have been created using bulked seedlots from breeding arboretums, plus-tree selections, or seed orchards. BwB is now being considered in breeding of Scots pine and Norway spruce in Norway, where stands are phenotyped using LiDAR scanning from drones, and a sample of top candidates and randomly selected trees is genotyped to ascertain their pedigree. A genomic relationship matrix is estimated from genetic markers and then used for breeding value prediction ([42], [92], [93], [94], [140]).
High-throughput genotyping enables the quantification of genomic relatedness between trees in a continuous and quantitative manner. This advancement allows for the substitution of pedigree-based relatedness matrices with their genomic counterparts in solving mixed model equations used for genetic evaluation, leading to superior accuracy of BLUP for breeding values ([64]), a method referred to as G-BLUP. Considering that many breeding programmes have generations or cohorts of individuals in the pedigree that cannot be genotyped, a hybrid matrix called H-BLUP has been developed ([90]). H-BLUP combines genomic and pedigree relatedness information, offering significant benefits in evaluation accuracy by integrating maximum genetic information without incurring additional genotyping costs. In the near future, both G-BLUP and H-BLUP versions are expected to be widely adopted across various species as straightforward and effective approaches to implementing genomic selection (Scots pine, maritime pine, and Norway spruce).
Genomic selection stands out as one of the most revolutionary applications of molecular markers in breeding, particularly in perennial species faced with the significant expenses and time delays associated with traditional evaluation methods ([56]). Although its routine implementation is not widespread across most species at present, it is highly plausible that it will emerge as a viable and valuable option for numerous forest breeding programmes in the near future. The most promising applications of genomic selection lie in its potential for evaluation of costly traits, such as those concerning wood quality or drought tolerance, and for traits that are challenging to assess directly, such as resistance to emerging diseases in the context of climate change. Genomic selection has the potential to increase selection intensity, minimise phenotyping costs, and include novel traits in breeding. It may also be used for early selection to speed up the breeding cycle, although this appears difficult in many conifers, which reach sexual maturity rather late ([99], [163]).
Data analytics
An important issue in long-term tree breeding is the need to maintain high levels of genetic variability for future breeding while maximising the short-term response to selection ([6]). Such levels of genetic variability over the long term may prove essential in the current context of substantial environmental change to ensure a minimum evolutionary potential in the face of unplanned pressures other than those generated by genetic gain. Finding the optimal balance of gain and diversity becomes difficult when the pool of selection candidates consists of related individuals from two or more generations, as is usual in all advanced-generation breeding programmes. Effective new tools, for example, based on the theory of genetic contributions and their optimization over one generation, such as OPSEL ([102]) and XDESIGN ([103]), have been developed and made available to breeders to carry out what is known as optimum contribution selection (OCS) or to optimise mating regimes. Other recent developments have gone further by proposing derivations of OCS with improved long-term performance ([147]).
High-throughput phenotyping, dense genotyping, and characterising the diverse experimental environments generate an immense volume of sometimes highly heterogeneous data. Dealing with such volumes and heterogeneity requires novel analytical approaches that prioritise integrating different layers of information to extract meaningful signals from noise. Platforms like R ([146]) have played a crucial role in enabling the development and accessibility of numerous innovative and continuously evolving tools, which have undergone testing and improvement by a rapidly growing user community. Numerous examples exist, although not all can be cited here. One noteworthy tool tailored to the needs of forestry field experiments is breedR ([105]) which leverages mixed models and incorporates modules for spatial statistics, interaction between trees, and genomic selection ([27], [148], [164]).
The characterization of the environment in field experiments is becoming increasingly important in forestry studies. We have moved from a situation where environmental heterogeneity was absorbed analytically in order to work with average yields, to placing the environmental gradient at the centre of genetic evaluation, which is undoubtedly necessary in the context of climate change ([37]). The aforementioned spatial statistical analyses, included in breedR, are widely used now in genetic trials to account for stand-level environmental variation ([11], [26]). The environment is a major explanatory factor when constructing and explaining the plastic reaction functions to the changes that the tree undergoes, in what is known as reaction norm. Random regression has been widely used in animal genetics, for example, in dairy cattle to model the evolution of lactation with age. However, this now classical but promising methodology has been less used in perennial plants, even though these are the organisms that present the greatest advantages in terms of characterization of the environment given their immobility. Random regression modelling has been recently investigated for predicting tree growth norms of reaction over environmental gradients in a pedigreed population ([98]). It is now being used in maritime pine breeding to assess genomic norms of reaction over a water balance index gradient using annual growth data obtained from annual rings ([112]). However, despite the promise of these norms of reaction construction techniques, there are still many aspects to be clarified regarding their routine use in breeding programs, given that their use represents a real paradigm shift between classical traits and the newer plasticity functions.
Tools for vegetative propagation and seed production
Mass vegetative propagation techniques greatly facilitate the dissemination of improved material through the use of large-scale clonal varieties and the setting up of clonal experiments that can greatly improve the accuracy of genetic evaluation. Somatic embryogenesis (SE) has emerged as a viable option in some species (stone pine, Norway spruce, eucalyptus, and Scots pine - see review [91], [41]). However, to make embryogenic plants competitive with seedlings, the SE operations must be further automated in order to reduce costs. An alternative is the development of vegetative propagation through cuttings, as it is being considered for maritime pine in Spain for deployment in the most productive areas or for Eucalyptus globulus in Portugal, but the fundamental issue is to develop a process that overcomes the limitations of poor rooting and high costs.
Seed production can be optimised through the development of techniques to accelerate flowering. It is under consideration in Scots pine and Norway spruce, as performing controlled crosses on selected individuals is typically the most time-consuming step of the breeding cycle. Various methods have been tested to promote flowering in these species, but the most promising ones include top-grafting onto sexually mature inter-stocks, gibberellin, heat and light treatments in greenhouses, and various damage-causing treatments applied to grafts ([44], [77]).
Optimal seed production in orchards faces several difficulties for most forest tree species. The first is the heterogeneous parental contribution and pollen contamination from unimproved surrounding stands when seed orchards are managed through open pollination. It can induce heavy losses in the genetic value of the crop ([22]). The second is the increasing damage due to biotic and abiotic factors, which contribute to a drastically lower seed yield. For example, maritime pine seed orchard productivity has plummeted since 2009-2010 ([19]), probably due to a combination of seed bug attacks (Leptoglossus occidentalis) and climatic factors (spring frost, summer drought). Similarly, in Norway spruce seed orchards, cones and seed infections cause significant losses in cone harvests ([121]). Various solutions are being examined, including chemical treatments and more intense management for seed production in a controlled greenhouse environment with mass pollination. In Norway spruce, the treatments proposed include the removal of Prunus species (known to be the primary hosts of cherry-spruce rust) in the proximity of orchards ([80]), and the removal of redundant cones ([4]). In Eucalyptus globulus, evolution of controlled pollination techniques has enabled the development of cost-efficient mass controlled pollination programmes to produce improved eucalyptus full-sibs ([61]). In Portugal, at Altri Florestal, controlled pollination has been carried out since 1984-1985. Initially, ten thousand flowers were pollinated, but since 2012, more than half a million flowers are pollinated every year through a simplified pollination technique where only two visits are needed (one for the controlled pollination and the other to collect the fruits that have been hand pollinated).
Transgenesis and new genomic techniques
Transgenic technologies in forest trees have been studied for decades ([150], [165]), but they have not entered operational breeding. The primary concerns revolve around the environmental and conservation risks linked to the spread of the transgene into natural gene pools ([143]). This dissemination might be more likely to occur within forest tree species, given the typically minimal genetic distinction between improved tree varieties and wild populations. Transgenic trees are specifically banned in PEFC and FSC certifications.
On the other hand, applications of biotechnology to non-food crops such as forest trees may be seen as more acceptable by the public than applications to food crops. The risk of transgene introduction into wild gene pools can be limited by containment strategies based on modified expression of floral regulatory genes leading to sterility or to the development of flowers impaired for the production of pollen or viable seeds ([86], [96]). In addition, in particular cultivation practises such as, for instance, poplar short-rotation coppices for energy use, the risk of gene flow is reduced as plants are harvested before reaching the flowering stage.
In the last ten years, the introduction of the New Genomic Techniques (NGT), comprising genome editing and cisgenesis, has opened new possibilities for the genetic improvement of forest trees. These techniques allow targeted mutagenesis, or the introduction of genes from sexually compatible donors to selected genotypes with minimal unwanted (off-target) modifications of the receiving genetic background. Although, NGTs are currently classified as GMOs following a ruling by the European Court of Justice in 2018, the European Commission ([45]) has come up with a proposal to treat plants obtained by targeted mutagenesis or cisgenesis that could also occur naturally or be produced by conventional breeding, similarly to conventional plants. Genome editing has been successfully applied in poplar for the modification of traits related to wood quality and resistance to biotic and abiotic stresses ([100]). However, the realisation of “clean” genome editing in trees is still challenging because the molecular components necessary for mutagenesis cannot be eliminated by crossing as easily as from annual crops. Several strategies have been proposed to overcome the problem, but since all of them have drawbacks or display low efficiency, further research on this topic is needed ([54]). The pattern of inheritance of traits of economic importance is another explanation for the low interest in these technologies. Most of these traits have a quantitative genetic basis, which means they are regulated by numerous genes, each having a minor impact on the trait, whereas genetic engineering methods are mostly suited to traits with relatively simple inheritance ([152]). However, genome editing technologies targeting multiple genes are under development ([159]). Furthermore, functional genomics studies have led to the characterization of several genes that have a profound impact on useful traits, such as drought tolerance ([124]). A limitation of these studies is that, in most cases, they were confined to the lab and greenhouse, which was partially due to legislative constraints in Europe. These constraints, however, may be removed in the near future with the evolution of European legislation. A step in this direction was taken in Italy in summer 2023, when the national Parliament pronounced favourably on the possibility of field trials for plants obtained by NGTs.
Climate change
Climate change is expected to reduce forest production for most tree species due to drought and the expansion of insects and disease ranges. Trees will also be stressed by the increased occurrence of extreme weather events, such as cold and heat waves, windstorms, and floods ([74], [75], [144], [2]). However, in the boreal region, where tree species are mainly constrained by temperature, the expected lengthening of the growing season may increase productivity ([12]). The increase in growth could be enhanced by FRM that is optimally adapted to the new conditions. A decision-support tool has been developed to help landowners in Sweden and Finland select the most productive FRMs for any site ([13]). In the temperate and Mediterranean regions, drought stress reduces tree growth and increases mortality, reducing wood production ([119]). Central Europe and the southern Nordic regions experienced extreme droughts in the early 2000s, when Norway spruce trees, especially those older than 40-50 years, showed poor drought tolerance ([122], [123], [65]). More recently, the extremely hot and dry conditions in the summer of 2018 in Central Europe, followed by bark-beetle attacks, led to major forest diebacks, specifically in Norway spruce plantations in lowlands, a forest crisis that is considered a turning point for the forest sector in Germany ([129], [120]). Even the growth of young trees was affected by drought, resulting in a significant genotype-by-environment interaction ([63]).
Douglas-fir has often been cited as a replacement alternative to other conifer species that are showing early symptoms of maladaptation in Europe, such as the forecited Norway spruce ([158], [120]). Nevertheless, some authors indicate that Douglas-fir is also showing symptoms of stress under extreme water scarcity conditions in some regions of Europe ([154]). In eucalyptus, drought and temperature increases are expected to be major causes of productivity decline in Portugal ([89]). In maritime pine and stone pine, drought stress also affects seed productivity, decreasing the yield in seed orchards ([107]). In southern environments, drought is increasingly a problem for Scots and maritime pine ([109], [51]). In poplar, unfavourable changes in wood composition following a drought stress treatment have been reported ([162]). Furthermore, it has been demonstrated recently that heat and drought stresses aggravate each other in poplar, leading to increased water loss by transpiration ([149]).
Although the initial eucalyptus plantings in Portugal had essentially no pests, the number of harmful fungi and insects has risen exponentially over time. The pine wood nematode (Bursaphelenchus xylophilus), now prevalent in Portugal, infects maritime pine forests and plantations, causing pine wilt disease and ultimately the mortality of infected trees in a matter of weeks or months. Because there is no phytosanitary management for this pest, the nematode has become a major threat to maritime and radiata pine plantations in Spain and France. For ash, the ash dieback disease, caused by the invasive fungus Hymenoscyphus fraxineus, is a relatively new disease, first observed in Poland in the 1990s. Since then, the fungus has spread rapidly over almost the entire natural range of ash in Europe, with a devastating impact on ash forests ([130]). New pests and diseases are likely to become new challenges for ash, such as the Emerald ash borer (Agrilus planipennis), which will require adapting the current breeding approaches to include more resistance traits. The difficulty of predicting the emergence and diffusion of pests is a major constraint ([55], [116], [132]). For example, in the case of poplar hybrids, there is a risk of releasing improved clones that might not perform as expected because of insufficient resistance.
Climate change clearly mandates adjustments to conventional approaches to field-based tree breeding. The first is the requirement to evaluate breeding materials in a variety of conditions that can be warmer and drier than those prevailing in the current target climate. In maritime pine breeding, for example, some candidates selected in France are already being tested in genetic trials in Spain, and the number of Spanish trial locations is expected to increase. However, as maritime pine is predicted to spread to Northern France, several experiments with genetic material selected in the current breeding zone are being conducted in these potential future production areas. The Norway spruce breeding programmes in Sweden, Finland, and Norway use clonally propagated candidates, which are evaluated in field experiments established in multiple different environments. Such field experiments facilitate the evaluation of phenotypic plasticity and the stability of the candidates ([81]). In poplar, some studies suggest that clones characterised by high productivity are generally less tolerant to abiotic stresses ([101], [7], [156]). These studies also highlight that the high degree of genetic variability among poplar clones should be further explored by re-assessing, in different environments, clones that are already established for their productivity and are grown in specific areas.
In several studies of Norway spruce, it has been shown that adaptive performance is influenced by the reproductive environment (summarised in [79]). Higher temperatures during zygotic embryogenesis and seed maturation ([78]) modify the phenology of future plants, resulting in progenies that are suited to a longer growth season ([136]), without known genetic selection on the way ([87]). Similar regulation has been found in other conifers, e.g, Scots pine ([39]), lodgepole pine ([161]), and the Picea glauca × P.engelmannii complex ([160]), as well as in poplar for agamic (cuttings) propagation material ([117]). This plasticity, which is often linked to epigenetic regulation of gene expression, can be utilised to speed up adaptation by moving seed orchards closer to the future climate or changing the temperature during zygotic embryogenesis.
The second predicted change in breeding activities of all the tree species is the introduction of new selection criteria connected to emerging biotic and abiotic stressors. Because breeding cannot respond quickly to environmental changes, it is vital to foresee which new stresses will be most severe and estimate the new selection criterion thresholds, such as minimum temperatures. Breeding programmes must also consider their adaptability to various scenarios. Some of the novel selection criteria may necessitate considerable investments, such as breeding for nematode tolerance in maritime pine, which has required the construction of a quarantine greenhouse in France. Investments in new traits may limit the resources available for work on existing breeding goals. On the other hand, delivering plant material with a sufficient level of tolerance to relevant pests, or abiotic stresses will ensure the long-term viability of profitable plantation forestry. For example, maritime pine silviculture in northwestern Iberia has ceased due to pine wood nematode predominance, and private spruce silviculture in the Central European lowlands has collapsed following large-scale diebacks.
Breeding programmes must seek out new sources of genetic variation to better meet new breeding objectives ([69], [18]). This might include increasing the size of current breeding populations, establishing new ones, for instance, those required for calibrating genomic evaluation. This variation must also be managed in an explicit and objective manner, using genomic information, to ensure not only long-term genetic gain, but also the adaptive capacity of the system ([147]). In maritime pine, the introduction of new genetic variation from non-local provenances is being examined, because, e.g., the Corsican provenance may be more tolerant to nematodes, but the Spanish, Portuguese, and Moroccan provenances appear to be more drought resilient. In eucalyptus, E. globulus can be crossed with other eucalyptus species that have a higher tolerance to drought and cold, or with a hybrid of E. rudis × E. saligna that is tolerant to the pest Gonipterus platensis. Also, when Populus deltoides and P. nigra were hybridised, new clones (P. ×canadensis) were produced that were more resistant to spring leaf and shoot blight ([52]).
Conclusions
Tree breeding and related research initiatives have demonstrated their ability to provide improvements in long-term wood production and associated services. However, owing to the length of time required for tree development, the expense of genetic testing, and issues with FRM manufacturing capacity, private investment in tree breeding has been limited. New technological opportunities related to genomics, phenotyping, and mass propagation help to alleviate these issues by reducing breeding costs, speeding the breeding process, and increasing the accuracy of genetic evaluations. They also allow for more accurate monitoring of genetic diversity and conservation for advanced breeding, which can benefit long-term genetic gains.
A disruptive effect on forest tree breeding cannot be achieved with a single technology but rather requires a combination of technologies. In addition to the desire for new methods, it is crucial to stress the significance of conventional breeding (e.g., field testing, crossing) and associated activities (in situ and ex situ conservation). Additionally, the large-scale diffusion of improved varieties will always be limited by the mass production of FRM. Therefore, investments in novel breeding techniques should be coordinated with those intended to enhance the efficiency of deployment.
Evolution in social needs and expectations is likely to influence tree breeding in various ways. Concerns have been expressed that planted stands and intense silvicultural practises make forests more vulnerable to diseases and extreme weather events. For instance, in Portugal, forest fires are commonly associated with the presence of large eucalyptus plantations. Although the issue is controversial ([48]), eucalyptus plantations on new lands have been banned since 2018. Conversely, other factors, such as the increasing public awareness of forest trees as carbon sinks and the creation of a carbon credit market ([151]), are likely to raise the profile of managed forests and related activities, such as forest tree breeding. Finally, we may expect a rising demand for bioeconomy raw materials, including, e.g., food additives, building materials, and various pulp-derived goods ([68]). The need to respond to both market and environmental changes will require a re-assessment of breeding strategies and, possibly, new tree species or hybrids that are more tolerant to predicted climate change and better suited to delivering new types of end-products.
From an organisational point of view, tree breeding is becoming more complex, requiring new knowledge and advanced technologies, for which traditional breeders are not necessarily trained. This will demand new types of training and competence, as well as changes that promote greater mutualism among breeding parties, which is likely to gradually eradicate the traditional paradigm of one tree species, one breeder.
Strengthening collaboration among countries could also be a useful tool for tackling breeding challenges in the context of climatic change and ensuring seed supply stability. Special attention must be paid to minor species with high ecological value but low economic importance, which rely on low-input breeding and limited funding. If new diseases and pests emerge due to climate change, these species will be even more vulnerable. European-wide collaboration, particularly during the pre-breeding phase, can increase the effectiveness of genetic improvement work in minor species by screening a wide range of germplasm for resistance and exchanging the best-performing genotypes for further breeding or seed orchard establishment.
Declarations
This work was supported by the European Union’s Horizon 2020 Research and Innovation Programme 813 Project under grant agreement no 773383 (B4EST).
References
CrossRef | Gscholar
Gscholar
CrossRef | Gscholar
Gscholar
CrossRef | Gscholar
Online | Gscholar
CrossRef | Gscholar
CrossRef | Gscholar
CrossRef | Gscholar
CrossRef | Gscholar
Gscholar
CrossRef | Gscholar
CrossRef | Gscholar
CrossRef | Gscholar
CrossRef | Gscholar
Online | Gscholar
Gscholar
Gscholar
Gscholar
CrossRef | Gscholar
CrossRef | Gscholar
Gscholar
Gscholar
Gscholar
CrossRef | Gscholar
CrossRef | Gscholar
CrossRef | Gscholar
CrossRef | Gscholar
Gscholar
Gscholar
CrossRef | Gscholar
CrossRef | Gscholar
Gscholar
CrossRef | Gscholar
Gscholar
CrossRef | Gscholar
CrossRef | Gscholar
Authors’ Info
Authors’ Affiliation
Stéphane Lemarié 0000-0003-1530-3787
Univ. Grenoble Alpes, INRAE, CNRS, Grenoble INP, GAEL, 38000 Grenoble (France)
Sven Mutke 0000-0002-6365-7128
Institute of Forest Science - ICIFOR-INIA, CSIC, carretera de La Coruña km 7.5, 28040, Madrid (Spain)
Research Centre for Forestry and Wood, Council for Agricultural Research and Economics - CREA-FL, v.le Santa Margherita 80, I-52100 Arezzo (Italy)
Marinus JM Smulders 0000-0002-8549-6046
Wageningen University and Research, Wageningen (Netherlands)
Giuseppe Nervo 0000-0002-8282-7110
Laura Rosso 0000-0002-2469-7602
Lorenzo Vietto 0000-0003-4489-6227
Council for Agricultural Research and Economics, Research Centre for Forestry and Wood, str. Frassineto 35, 15033 Casale Monferrato (Italy)
Agostino Fricano 0000-0003-3715-5834
Council for Agricultural Research and Economics, Research Centre for Genomics and Bioinformatics, v. San Protaso 302, 29017 Fiorenzuola d’Arda (Italy)
INRAE, UMR 0588 BioForA, 2163 avenue de la Pomme de Pin, CS 40001 Ardon, 45075 Orléans Cedex 2 (France)
Laboratoire de Recherche en Sciences Végétales, Université Toulouse, CNRS, INP, Castanet-Tolosan (France)
The Forestry Research Institute of Sweden - Skogforsk, Ekebo 2250, 26890 Svalöv (Sweden)
TRAGSA, Vivero Maceda, Carretera Maceda - Baldrei, km 2, 32708 Maceda, Galicia (Spain)
The Forestry Research Institute of Sweden - Skogforsk, Sävar SE-918 21 (Sweden)
Norwegian Institute of Bioeconomy Research - NIBIO, Skolegata 22, 7713 Steinkjer (Norway)
Corresponding author
Paper Info
Citation
Fugeray-Scarbel A, Bouffier L, Lemarié S, Sánchez L, Alia R, Biselli C, Buiteveld J, Carra A, Cattivelli L, Dowkiw A, Fontes L, Fricano A, Gion J-M, Grima-Pettenati J, Helmersson A, Lario F, Leal L, Mutke S, Nervo G, Persson T, Rosso L, Smulders MJM, Steffenrem A, Vietto L, Haapanen M (2024). Prospects for evolution in European tree breeding. iForest 17: 45-58. - doi: 10.3832/ifor4544-017
Academic Editor
Marco Borghetti
Paper history
Received: Dec 16, 2023
Accepted: Feb 27, 2024
First online: Mar 06, 2024
Publication Date: Apr 30, 2024
Publication Time: 0.27 months
Copyright Information
© SISEF - The Italian Society of Silviculture and Forest Ecology 2024
Open Access
This article is distributed under the terms of the Creative Commons Attribution-Non Commercial 4.0 International (https://creativecommons.org/licenses/by-nc/4.0/), which permits unrestricted use, distribution, and reproduction in any medium, provided you give appropriate credit to the original author(s) and the source, provide a link to the Creative Commons license, and indicate if changes were made.
Web Metrics
Breakdown by View Type
Article Usage
Total Article Views: 2423
(from publication date up to now)
Breakdown by View Type
HTML Page Views: 1484
Abstract Page Views: 389
PDF Downloads: 518
Citation/Reference Downloads: 4
XML Downloads: 28
Web Metrics
Days since publication: 52
Overall contacts: 2423
Avg. contacts per week: 326.17
Article Citations
Article citations are based on data periodically collected from the Clarivate Web of Science web site
(last update: Feb 2023)
(No citations were found up to date. Please come back later)
Publication Metrics
by Dimensions ©
Articles citing this article
List of the papers citing this article based on CrossRef Cited-by.
Related Contents
iForest Similar Articles
Research Articles
Adaptive response of Pinus monticola driven by positive selection upon resistance gene analogs (RGAs) of the TIR-NBS-LRR subfamily
vol. 10, pp. 237-241 (online: 02 February 2017)
Research Articles
Early flowering and genetic containment studies in transgenic poplar
vol. 5, pp. 138-146 (online: 13 June 2012)
Review Papers
Genetic diversity and forest reproductive material - from seed source selection to planting
vol. 9, pp. 801-812 (online: 13 June 2016)
Research Articles
Genetic variation and heritability estimates of Ulmus minor and Ulmus pumila hybrids for budburst, growth and tolerance to Ophiostoma novo-ulmi
vol. 8, pp. 422-430 (online: 15 December 2014)
Research Articles
Testing Hungarian oak (Quercus frainetto Ten.) provenances in Romania
vol. 13, pp. 9-15 (online: 08 January 2020)
Review Papers
Breeding and improvement of black locust (Robinia pseudoacacia L.) with a special focus on Hungary: a review
vol. 16, pp. 290-298 (online: 28 October 2023)
Research Articles
Age trends in genetic parameters for growth and quality traits in Abies alba
vol. 9, pp. 954-959 (online: 07 July 2016)
Research Articles
Preliminary study on genetic variation of growth traits and wood properties and superior clones selection of Populus ussuriensis Kom.
vol. 12, pp. 459-466 (online: 29 September 2019)
Research Articles
Pollen contamination and mating patterns in a Prosopis alba clonal orchard: impact on seed orchards establishment
vol. 12, pp. 330-337 (online: 14 June 2019)
Technical Advances
Gene flow in poplar - experiments, analysis and modeling to prevent transgene outcrossing
vol. 5, pp. 147-152 (online: 13 June 2012)
iForest Database Search
Google Scholar Search
Citing Articles
Search By Author
- A Fugeray-Scarbel
- L Bouffier
- S Lemarié
- L Sánchez
- R Alia
- C Biselli
- J Buiteveld
- A Carra
- L Cattivelli
- A Dowkiw
- L Fontes
- A Fricano
- J-M Gion
- J Grima-Pettenati
- A Helmersson
- F Lario
- L Leal
- S Mutke
- G Nervo
- T Persson
- L Rosso
- MJM Smulders
- A Steffenrem
- L Vietto
- M Haapanen
Search By Keywords