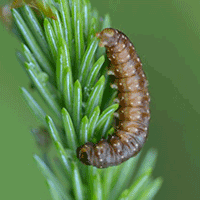
Forest health under climate change: impact of insect pests
iForest - Biogeosciences and Forestry, Volume 17, Issue 5, Pages 295-299 (2024)
doi: https://doi.org/10.3832/ifor4520-017
Published: Sep 30, 2024 - Copyright © 2024 SISEF
Review Papers
Collection/Special Issue: Project LIFE MODERn(NEC)
Workshop "Climate change and forest health monitoring in Italy" - Rome (Italy) May 5, 2023
Guest Editors: Bussotti F, Pollastrini M
Abstract
The impact of climate change on forests is difficult to predict, as it depends on multiple factors and the final effect may vary in different parts of our planet. However, these effects can be sorted in “direct”, when they act directly on plants, and “indirect”, when they act through other agents. Among the indirect ones, there are those mediated by biotic factors, particularly insect pests. The impact of climate change on insect pests varies depending on the insect species and the type of forest. Some plant-feeding insects may be disfavoured by climate change, with a consequent reduction in population density and thus in damage to forests. However, many outbreaks of forest insects have been recently recorded as related to climate change. In fact, some insect pests, in certain regions of the world, may benefit from higher temperatures, as it has been demonstrated for some devastating defoliators. In addition, more frequent drought and extreme events may favour other pests, particularly bark beetles and wood-boring insects. Bark beetles are the most dangerous ones because their aggressiveness changes with population density. They can attack only stressed trees at low population densities, while, once the populations have reached high density, they are even able to attack healthy trees in widespread areas.
Keywords
Introduction
Climate change may have positive or negative effects on forests, depending on local initial conditions ([1]). Positive effects are perceived for example by the so-called “energy-limited forests”. These are forests growing in cold climates, thus limited by a short growing season, like sub-alpine forests. In this case, a growing season that is prolonged by climate change and ensuing warmer temperatures, may positively affect tree growth ([45]). On the other hand, most forests are limited by water availability. In fact, water is the main limiting factor for plant productivity all over our planet ([34]). In this type of forests, a warmer climate, associated with increased drought, has generally a negative effect on tree health ([45]). Hereafter, in this paper we will refer in particular to the forests of the temperate and boreal regions.
Although the negative effects of climate change are numerous, variable, and interacting with each other, they can be roughly distinguished into “direct” and “indirect”. As regards direct adverse effects on forest ecosystems, the acting factors are temperature, precipitation, wildfires, and extreme weather events. For example, higher temperatures and/or water stress have been identified as the most probable causes of increased tree mortality in southern European and western North American forests ([1]). Higher mortality is observed in different forest types, independently of tree size or plant genera ([5]).
The increase in wind damage is another phenomenon caused by climate change ([30]). Windstorms represent one of the main abiotic disturbances in European forests and the main cause of forest damage ([30]). However, it is not clear if this increase in damage is due to higher storm frequency and intensity or to higher tree susceptibility. In fact, temperature and precipitation changes alter soil proprieties and it is supposed that trees in these conditions are more prone to wind damage ([30]).
Finally, an increase in wildfires is also expected because of climate change ([37]). In fact, due to warmer and drier climate conditions occurring in various regions of the globe, an increase in fire-prone areas is expected globally, particularly in temperate and boreal climates ([38]). Furthermore, warmer seasons, as well as the lengthening of the potential fire season, and thus drier vegetation, cause an increment in the frequency and duration of burning fires ([46]) and finally higher wildfire damage.
Indirect negative effects are to be added to the aforementioned direct ones. Climate stressors can have deleterious effects on tree vigour, acting through different mechanisms. For example, water stress reduces carbon availability leading to carbon starvation, which threatens plant survival ([1]). In addition, tree vigour can be reduced also through effects on their symbionts. In fact, although the impact of climate change on plant microbiota is poorly understood ([41]), it is hypothesized that climate stressors can alter the diversity and structure of microbial communities ([13]), possibly causing plant microbiota dysbiosis ([41]). In both cases the final effect is an increase in plant susceptibility to biotic factors ([1], [45], [31]), particularly pathogens and insect pests. However, since climate changes affect at the same time trees, insect pests, as well as their interactions, the combined effect is very complex and hardly predictable. As mentioned above, some warm-adapted insect pests can benefit from higher temperatures and drier climates ([7]), leading to increased damage to forests. Conversely, other pest species may be negatively affected and, as a result, their population densities may decrease, ultimately leading to reduced forest damage ([28], [33]).
Effect of insect pest response to climate change on forests
Climate change may alter the frequency and intensity of forest pest outbreaks ([44]), however, these effects may vary, as outbreaks can be enhanced or decreased by the changed climatic parameters ([22]). The response of insect pests, in fact, may depend on the intensity of climate stressors as well as on the insect species (Tab. 1). Effects on certain species are well studied, however, generalization is not possible, as each species reacts very differently to the changing climate ([31]). In addition, the same species may have multiple and contrasting responses to climate change, in other words, its damage may increase or decrease according to the local community and geographical region ([22], [12]). Some species increase the outbreak intensity in the northern part of their range, while reducing it in the southern parts ([22], [18]). Many insects are shifting their range poleward and to higher elevations ([12]). In addition, climate factors may act in different ways on insects, affecting their survival (directly or through the activity of their natural enemies), their life cycle, their dispersal capacity, their distribution, and their trophic interactions ([4]).
Tab. 1 - Examples of negative and positive effects on insects of various abiotic factors involved in climate change. Impact: Impact of climate change on insect populations; (*): Mechanism by which the effect on the insect population is achieved.
Impact | Feeding guild | Abiotic factor | Insect species | Acting mechanism (*) | References |
---|---|---|---|---|---|
Negative impact | Sapsucking insects | Water stress | Adelges abietis | Higher plant resistance | [32] |
Higher temperatures | Aphids | Increase in coccinellid predators | [39] | ||
Defoliators | Water stress | Operophtera brumata | Reduction in food quality | [15] | |
Higher winter temperatures | Zeiraphera diniana | Diapause disruption | [2] | ||
Warmer spring temperatures | Desynchronization with host plant | [2] | |||
Xylophagous insects | Higher temperatures (above the optimum) | Dendroctonus frontalis | Decline of population growth | [8] | |
Higher temperatures | Agrilus planipennis | Shorter oviposition period | [47] | ||
Positive impact | Sapsucking insects | Higher temperatures | Phenacoccus solenopsis | Higher growth rate and better tending by ants |
[49] |
Defoliators | Higher temperatures | Cephalcia arvensis | Higher larval survival and faster development | [2] | |
Lymantria monacha and Lymantria dispar | Higher larval survival and faster development | [16] | |||
Higher winter temperatures | Lymantria dispar | higher winter survival of eggs | [28] | ||
Neodiprion sertifer | higher winter survival of eggs | [43] | |||
Operophthera brumata | higher winter survival of eggs | [17] | |||
Thaumetopoea pityocampa | Higher overwintering survival, higher females’ fecundity, increase in distribution range and outbreak areas | [2], [3], [40] | |||
Xylophagous insects | Higher temperatures | Agrilus planipennis | Reduction in mortality by parasitoids | [47] | |
Dendroctonus ponderosae | Increase in distribution range and outbreak areas | [23] | |||
Ips typographus | Faster larval development and more generations per year | [21], [19] | |||
Water stress | Dendroctonus spp. | Higher tree susceptibility | [10], | ||
Tomicus piniperda and Tomicus minor |
Higher tree susceptibility | [20] | |||
Windstorms | Ips typographus | Higher tree susceptibility | [24], [29], [27] |
||
Wildfires | Dendroctonus spp. and Ips spp. | Higher tree susceptibility | [25], [14] |
||
Ips pini | Higher tree susceptibility | [35], [14] |
Negative impact of climate change on insect populations
Temperatures
Insects, being ectothermic organisms, are strongly affected by temperature. Each species has a specific temperature range within which finds the proper conditions to survive. Thus, summer higher temperatures linked to climate change may negatively affect insects. If the temperature increases above this range, adverse effects may occur, such as decreased individual size, fecundity, dispersal capacity, and increased mortality rates ([8], [47], [15], [32]). Mild winter temperatures may affect insects differently. Certain insect species require chilling periods to enter the obligate winter diapause, so the privation of these lowering temperatures may lead to higher mortality. In addition, higher winter temperatures lead to a snowfall decrease and an earlier snowmelt. This may negatively affect the survival of species that benefit from snow protection during overwintering in the soil ([28]).
Insect-plant interactions
Changing climate may affect interactions between insect pests and their host plant. For example, due to climate change some specialist insects, strictly synchronized with host plant, may be negatively affected by a change in plant phenology. Some defoliators may have a short timeframe within which leaves provide proper quality food ([26]). Thus, if climate change acts differently on these species’ phenology than on that of its host, a desynchronization may occur ([48], [31]). Lack of synchrony leads to a reduction in insect abundance ([2], [33]) and thus plant damage is also reduced.
Severe water stress, which is expected with climate change, can modify the content of primary and secondary metabolites in plants, reducing nitrogen and water content, and thus affecting plant-feeding insects ([12]). Based on the most accepted hypothesis, the effects on insects depend on their feeding guild and the intensity of the stressors. Sap-sucking insects, as well as defoliators and leaf-mining insects, for example, are negatively affected by severe droughts as the weather-stressed host offers them low-quality food ([28], [9], [12]).
Natural enemies
Finally, since the insect pest density also depends on natural control agents, the responses of their natural enemies to climate change should be considered. Effects are not easily predicted and authors have contrasting opinions about them. Some researchers argue that a warming climate can be more deleterious on predators or parasitoids than on their prey or hosts, with beneficial effects on pest populations ([47], [12]). Conversely, others think that reduced food quality for insect pests, with the resulting lengthening of their life cycle, exposes them for longer periods to their natural enemies ([39], [28]). Based on this last theory, pest population densities would be reduced by climate change.
In conclusion, some insect pests may be negatively impacted by climate change, either directly, with effects on their survival or performances, or indirectly, through changes in host plant phenology or their natural enemies’ performance. This kind of effect may lead to a reduction in the population density of insect pests and in some cases to their range contraction ([22], [12]), with a consequent reduction of damage to forests.
Positive impact of climate change on insect populations
Temperatures
Warmer climate may favour some insect pests, particularly in regions where their optimal temperatures are not reached. Insect development rates strictly depend on temperatures: it is faster at optimal temperatures, while it slows down at suboptimal temperatures, regardless of them being higher or lower. Each species has a different sensibility to temperatures and a different optimal level. Some insect pests may be favoured in some regions, particularly those at higher latitudes, where temperatures are lower than their optimal level ([22]). Here, an increase in temperatures would accelerate their metabolic rate, thus increasing their development and movement capability, as well as reducing their mortality, ultimately leading to an increase in population density ([6], [16], [49]).
A higher development rate leads to shorter life cycles and, for multivoltine species, to a higher number of generations per year. This is also enabled by an extended growing season, which generally occurs due to climate change ([21], [19], [16], [12]). Therefore, clearly, if an insect pest has more generations per year, the damage it causes to plants increases too ([16]).
In addition, in areas where winter low temperatures are a limiting factor for insects, an increase in temperatures may reduce mortality rates of insects without an obligate winter diapause ([6]). This was demonstrated both for species that overwinter as eggs and those overwintering as larvae. Some examples are forest defoliators like the winter moth Operophthera brumata L., the gypsy moth Lymantria dispar (L.), and the European pine sawfly Neodiprion sertifer (Geoffroy), whose egg mortality rates have been reduced by higher winter temperatures ([43], [17], [28]). In the case of defoliators which overwinter as larvae the effect is more complex. One of the most studied cases is that of a Mediterranean species, namely the pine processionary moth, Thaumetopoea pityocampa (Denis et Schiffermüller). Its larvae actively feed during winter if temperatures allow it. This winter feeding reduces overwintering mortality and increases insect performances, leading to an increase in females’ fecundity ([3], [2], [40]).
With the warming climate, many insect pests are expanding their distribution and outbreak areas in regions that previously exceeded their thermal tolerance. In these new environments, they may have a higher impact due to the lack of coevolution, with higher damage to plants. This has been confirmed for many species of bark beetles and defoliators, which have recently increased their performances at higher elevations ([31]). Again T. pityocampae may serve as an example, as its outbreaks have been recently reported beyond both the northern and the altitude limits of its historical occurrence ([2]).
Insect-plant interactions
The multiple negative effects of climate change make forest trees prone to pest attacks. Adverse effects of warm temperatures and drought reduce plant defences, making them susceptible to pests. Indeed, while sap-sucking insects and defoliators may be negatively affected by severe droughts, generally, xylophagous insects benefit from them ([31], [28], [9], [12], [42]). In fact, many outbreaks of bark beetles have recently been reported after drought events. The main species involved in this phenomenon belong to the Tomicus, Ips, and Dendoctronus genera ([10], [1], [20]).
Finally, outbreaks by bark beetles and wood boring insects can be triggered by increasingly frequent windstorm and wildfire disturbances. As a result of these events abundant breeding material is made available for those insect species that exploit felled and broken trees, partially burned trees, and stressed trees in general. This kind of material is suitable for pest colonization, causing the population to build up rapidly ([35], [25], [14], [37], [15]). Especially for bark beetles, this situation is even more complex because their aggressiveness changes with population density. Once the population has reached high density the pest is even able to attack healthy trees, not only stressed ones ([24]). Initially, this occurs in single “spots”, near weakened trees, then on multiple spots, and finally in widespread areas ([36]).
An example is the European spruce bark beetle, Ips typographus (L.) which has caused severe outbreaks after windstorms in Europe on various occasions ([29], [24]). The more recent one is the “Vaia” storm occurred in the Alps in 2018, which has caused a huge infestation (still in progress) in the Italian spruce forests, even without the contribution of other climatic stressors ([27]).
Although adverse effects on insect pests are possible, many outbreaks of forest insects have been related to climate change ([1], [31]). In fact, outbreaks of many insect pests, mainly bark beetles and defoliators, have been recorded in different areas of the world after extreme droughts. For example, the outbreaks of the two aggressive bark beetles Dendroctonus ponderosae Hopkins and I. typographus were associated to higher availability of trees stressed by droughts or to faster biological cycles favoured by higher temperatures ([10], [21], [19]). Similarly, favourable climate promoted the survival and speeded up the development of some main forest defoliators, like the web-spinning sawfly Cephalcia arvensis Panzer ([2]). To these, we may add the aforementioned bark beetle outbreaks recorded after large and more frequent windstorms and wildfires ([29], [24], [27]).
Conclusions
The impact of climate change on forests is difficult to predict, particularly when it is mediated by insect pests, as it can vary depending on various factors ([15]). In any case, many outbreaks of forest insects have been recently recorded as related to climate change ([2], [6], [23], [33]). Generalisation is not possible, as many studies agree that insects belonging to different feeding guilds have different reactions to climate change. While the majority of sapsuckers and defoliators may be negatively affected by severe drought ([31], [11], [12]), they may benefit from higher temperatures, if these do not exceed their optimal threshold ([28], [15]). On the contrary, bark beetles and wood-boring insects generally benefit from both types of climate modifications ([10], [21], [23], [20]); furthermore, they may be favoured by the occurrence of wildfires and extreme windthrows ([24], [37], [15]). Anyway, the impact of insect pests varies depending on the insect species and the type of forest, in addition, the same insect species may decrease or increase both outbreak frequency and intensity in the different regions of its distribution range ([22], [12], [18]).
Acknowledgments
The research has been carried out within the project “LIFE MODERn(NEC) - new MOnitoring system to Detect the Effects of Reduced pollutants emissions resulting from NEC Directive adoption” - LIFE20 GIE/IT/000091.
FB contributed to bibliography curation, as well as writing, reviewing and editing of the manuscript. MB and TP contributed equally to the manuscript preparation.
References
CrossRef | Gscholar
CrossRef | Gscholar
CrossRef | Gscholar
CrossRef | Gscholar
CrossRef | Gscholar
Gscholar
Gscholar
Online | Gscholar
CrossRef | Gscholar
Authors’ Info
Authors’ Affiliation
Tiziana Panzavolta 0000-0002-8985-3071
Department of Agriculture, Food, Environment and Forestry - DAGRI, Plant Pathology and Entomology section, University of Florence (Italy)
National Institute for Research and Development in Forestry “Marin Dracea” (Romania)
Corresponding author
Paper Info
Citation
Bracalini M, Balacenoiu F, Panzavolta T (2024). Forest health under climate change: impact of insect pests. iForest 17: 295-299. - doi: 10.3832/ifor4520-017
Academic Editor
Martina Pollastrini
Paper history
Received: Nov 07, 2023
Accepted: Jun 27, 2024
First online: Sep 30, 2024
Publication Date: Oct 31, 2024
Publication Time: 3.17 months
Copyright Information
© SISEF - The Italian Society of Silviculture and Forest Ecology 2024
Open Access
This article is distributed under the terms of the Creative Commons Attribution-Non Commercial 4.0 International (https://creativecommons.org/licenses/by-nc/4.0/), which permits unrestricted use, distribution, and reproduction in any medium, provided you give appropriate credit to the original author(s) and the source, provide a link to the Creative Commons license, and indicate if changes were made.
Web Metrics
Breakdown by View Type
Article Usage
Total Article Views: 8114
(from publication date up to now)
Breakdown by View Type
HTML Page Views: 5268
Abstract Page Views: 1086
PDF Downloads: 1541
Citation/Reference Downloads: 27
XML Downloads: 192
Web Metrics
Days since publication: 285
Overall contacts: 8114
Avg. contacts per week: 199.29
Article Citations
Article citations are based on data periodically collected from the Clarivate Web of Science web site
(last update: Mar 2025)
(No citations were found up to date. Please come back later)
Publication Metrics
by Dimensions ©
Articles citing this article
List of the papers citing this article based on CrossRef Cited-by.
Related Contents
iForest Similar Articles
Research Articles
Distribution and abundance of the alien Xylosandrus germanus and other ambrosia beetles (Coleoptera: Curculionidae, Scolytinae) in different forest stands in central Slovenia
vol. 12, pp. 451-458 (online: 29 September 2019)
Review Papers
Dutch elm disease and elm bark beetles: a century of association
vol. 8, pp. 126-134 (online: 07 August 2014)
Research Articles
A bark beetle infestation predictive model based on satellite data in the frame of decision support system TANABBO
vol. 13, pp. 215-223 (online: 06 June 2020)
Research Articles
Hygroscopicity of the bark of selected forest tree species
vol. 10, pp. 220-226 (online: 06 November 2016)
Research Articles
Determination of differences in temperature regimes on healthy and bark-beetle colonised spruce trees using a handheld thermal camera
vol. 14, pp. 203-211 (online: 02 May 2021)
Research Articles
Estimating the potential threat of increasing temperature to the forests of Turkey: a focus on two invasive alien insect pests
vol. 15, pp. 444-450 (online: 03 November 2022)
Short Communications
Effective monitoring as a basis for adaptive management: a case history of mountain pine beetle in Greater Yellowstone Ecosystem whitebark pine
vol. 2, pp. 19-22 (online: 21 January 2009)
Research Articles
Tree-ring-based reconstruction of larch budmoth outbreaks in the Central Italian Alps since 1774 CE
vol. 12, pp. 289-296 (online: 27 May 2019)
Research Articles
Effect of stand density on longitudinal variation of wood and bark growth in fast-growing Eucalyptus plantations
vol. 12, pp. 527-532 (online: 12 December 2019)
Research Articles
Exposure elevation and forest structure predict the abundance of saproxylic beetles’ communities in mountain managed beech forests
vol. 16, pp. 155-164 (online: 08 June 2023)
iForest Database Search
Search By Author
Search By Keyword
Google Scholar Search
Citing Articles
Search By Author
Search By Keywords
PubMed Search
Search By Author
Search By Keyword