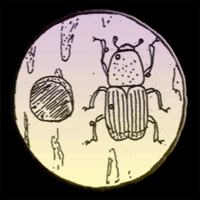
Dutch elm disease and elm bark beetles: a century of association
iForest - Biogeosciences and Forestry, Volume 8, Issue 2, Pages 126-134 (2015)
doi: https://doi.org/10.3832/ifor1231-008
Published: Aug 07, 2014 - Copyright © 2015 SISEF
Review Papers
Collection/Special Issue: 3rd International Elm Conference, Florence (Italy - 2013)
The elms after 100 years of Dutch Elm disease
Guest Editors: A. Santini, L. Ghelardini, E. Collin, A. Solla, J. Brunet, M. Faccoli, A. Scala, S. De Vries, J. Buiteveld
Abstract
Bark beetles of the genus Scolytus Geoffroy are the main vectors of the fungus Ophiostoma ulmi s.l., which causes the Dutch elm disease. The large and small elm bark beetles - S. scolytus (F.) and S. multistriatus (Marsham), respectively - are the most common and important species spreading the pathogen worldwide. The success of the pathogen-insect interactions is mainly due to the characteristic reproductive behavior of the elm bark beetles, which, however, largely depends on the occurrence of infected trees. During feeding activity on elm twigs, callow adults carrying pathogen conidia on their bodies contaminate healthy trees and facilitate pathogen development and movement within the wood vessels. Infected trees become then suitable for insect breeding in the stem bark. This well-known mutualistic association has devastating consequences for elm survival. Although much is known about insect-pathogen interactions and transmission mechanisms, many topics still deserve additional attention, as, for example, beetle systematic based on new molecular tools and morphological characters; selection of European elm clones based on disease avoidance; consequences of global warming on life-history of the three organisms (fungus-insect-tree) involved in the pathosystem; new problems resulting from the rapid increase of international trade among continents, leading to the accidental introduction of new vector species or new pathogen species or races, or to the introduction of new highly susceptible elm species in gardens and public parks. A holistic approach to tackle the problem is highly recommended, taking into account how these organisms interact with each other and the environment, and how their interactions could be modified in order to face one of the most destructive diseases ever known in plant pathology.
Keywords
Dutch Elm Disease, Elm Barck Beetles, Scolytus-Ophiostoma Interactions, DED Cycle, Avoidance Mechanisms, Disease Escape, Resistance
Introduction
Dutch elm disease (DED), caused by some Ascomycete fungi of the genus Ophiostoma (Ophiostoma ulmi s.l.), is one of the most destructive diseases of woody trees ever known in plant pathology. The severity and devastation of its pandemics stirred up the interest of public opinion and researchers ([106]). Since prehistory, elms (Ulmus spp.) are strictly linked to human activities, providing a number of different fo-rest, agricultural and cultural services. DED spreading and infection of suitable hosts is mainly due to a synchrony between life cycles of host-tree, pathogen and elm bark beetles (Coleoptera: Curculionidae, Scolytinae), the main fungus vectors. Such a synchrony allows the insect vectors to spread the pathogen when host plants are more prone to be infected and temperatures are favorable to the fungus growth, enhancing the pathogen aggressiveness.
After a century since the beginning of the first pandemic of DED in Europe ([127]), and the introduction in the USA of one of its most effective vector, the small elm bark beetle Scolytus multistriatus ([27]), the amount of available scientific data is indeed vast and covers many aspects. The aim of the present paper is to provide a thorough review of the knowledge concerning the association between DED pathogens and vectors. Possible challenges for future research topics are also explored.
Ophiostoma ulmi s.l. and Dutch elm disease
As a result of DED, during the last century elms suffered major losses worldwide, with the near-total disappearance of adult trees in many European, Asian and North American areas. Two pandemics occurred. The first, caused by O. ulmi (Buisman) Nannfield, began in Europe in the 1910s ([127]) and rapidly devastated elm populations in Europe and, 20 years later, in North America ([18], [62]). Around 1940, the disease declined in Europe ([14], [86]). A few years later, in the mid-1900s, a second and more destructive pandemic caused the widespread destruction of mature elms in Europe, Western Asia and North America ([58], [19]). This second still active pandemic is due to a different species, the highly virulent O. novo-ulmi Brasier ([17]), which has almost totally replaced O. ulmi. Moreover, two subspecies of O. novo-ulmi are known: O. novo-ulmi ssp. novo-ulmi, previously known as the Euro-Asian race (EAN), and O. novo-ulmi ssp. americana, previously known as the North American race (NAN - [14], [19]). Since the 1980s, the presence of hybrids has been detected between these two subspecies. The hybrids, whose pathogenicity does not differ from that of their parent subspecies ([111]), are now expanding across the continents ([20]). A third species, O. himal-ulmi Brasier & Mehrotra, which also causes DED, was identified in the Himalayas ([21]), but its presence has still not been reported in Europe or North America.
The destructiveness and severity of this disease is mainly due to the rapidity and efficiency of its spread, which is particularly quick and effective because O. ulmi s.l. has exploited an ancient association between the native saprotrophic species O. quercus and the elm bark beetles active in Europe prior to the arrival of O. ulmi ([16]). Various species of elm bark beetles are involved in the disease transmission. Callow beetles (i.e., sexually immature) emerge in spring from the bark of dying infected elms and fly to the crown of healthy elms to feed at the crotches of young twigs. Infected beetles contaminate healthy elms by carrying the pathogen spores into feeding wounds, in direct contact with the host’s vascular tissues. Spores germinate into a growing mycelium and reach the xylem, where the pathogen moves into the vessels through a yeast multiplication phase ([139]). Later on, the beetles move to dying elms, i.e., infected by DED, to lay eggs in the inner bark of the stem and main branches, which provide an ideal environment for both larval development ([107]) and pathogen fructification ([139]). The new contaminated beetles emerge from the bark and move toward the crown of new healthy elms, completing the cycle. The infection may also occur through root anastomoses between infected and healthy trees ([139]).
Initial external symptoms of DED include crown discoloration and leaf wilting. Symptoms spread along branches centrally, affecting the entire crown, and the tree dies rapidly. A typical internal symptom of the disease is the formation of a brown ring in the infected sapwood due to the formation of tyloses and gels in the xylem vessels ([130], [105], [93], [94], [37]), resulting in their obstruction ([147], [91]). A systemic infection drastically reduces the hydraulic conductivity in the functional xylem, resulting in a severe wilt syndrome which kills the tree rapidly ([77]).
Life history of the elm bark beetles
Bark beetles belonging to the genus Scolytus Geoffroy are the main vectors of Ophiostoma ulmi s.l. ([139]). Although about 10 species of Scolytus live on elms ([144], [99]), the large and small elm bark beetles - S. scolytus (F.) and S. multistriatus (Marsham), respectively - are the most common and important species spreading the pathogen worldwide ([141], [139], [140], [137], [138], [40], [41]).
Many species of elms have been recorded as potential elm bark beetle hosts, both in the insects’ native distribution range and in countries of new introduction ([3], [128], [85], [144], [99]). Elm bark beetles attack trees dying, stressed or weakened by different factors (e.g., drought, diseases, pruning, defoliations). Mature beetles identify potentially suitable hosts by a blend of volatiles released by damaged or diseased elms ([84], [97]). Following initial attacks by the pioneer beetles, most of the insect populations find the suitable hosts in response to a blend of aggregation pheromones released by conspecific insects ([97], [143], [41]), assuring the whole bark colonization ([96]).
Elm bark beetles usually lay eggs in the phloem of weakened trees (Fig. 1). After finding and acceptance of the host, elm bark beetle females bore an entrance hole through the tree bark, communicating with a small mating (or nuptial) chamber excavated in the phloem, where mating occurs ([51], [132]). Each mated female excavates a maternal tunnel (or egg gallery) in the phloem where eggs are laid along both sides. The maternal galleries run parallel to the wood fibers, without ramifications ([116], [24], [1]). Construction of the maternal tunnels and egg-laying take about three weeks ([12]). Larvae hatch about one week after oviposition, and immediately begin to bore characteristic larval galleries developing in an orthogonal direction from the maternal ones ([3], [24], [1]). Larval galleries of Scolytus spp. are 60-150 mm long on average and they cross each other only rarely ([12], [23], [78], [70]). Larval tunnels become wider as the larvae develop and move away from the maternal gallery ([12]). Larvae feed in the phloem for about 30 days, passing through 5 developing instars before becoming fully grown ([51]). Shortly before pupation, mature larvae bore a pupal chamber in the external part of the sap-wood, where they metamorphose first into pupae ([139], [137]) and after about two weeks into adults ([146]). The new callow adults then emerge from the bark of the host tree through a hole excavated directly from the pupal chamber ([70]). After emergence, adults fly and disperse looking for healthy trees on which they carry out the sexual maturation feeding. Elm bark beetles may be mono- or bivoltine. In favorable climatic conditions there are usually two generations per year ([30]), the first starting in late spring (May-June) and emerging in late summer (August-September), the second beginning in autumn (September) overwintering as larvae, and emerging in the following spring ([12], [23], [51], [71], [146]).
Fig. 1 - Dutch elm disease cycle (modified from [53]).
In all monogamous bark beetle species, before reproducing in the bark of dying trees, the newly emerged callow adults need a period of sexual maturation reached by feeding in crotches of 2-3 years old twigs of healthy and vigorous elms. The maturation feeding lasts a few days, during which the insects excavate short tunnels (2-4 cm long) in the twig phloem and sapwood ([51], [139]). Twig feeding, which is a prerequisite for sexual maturation of callow adults, is associated also with restoration of the beetle reserves (food and water - [51], [132], [71], [64], [75]). Although Scolytus beetles prefer twigs occurring on the upper part of the crown ([132], [141]), feeding tunnels can be made in almost any young and sappy bark ([51], [71], [141]). Scolytus beetles can stay in the feeding tunnels for up to 13 days ([51]). When sexual maturity is reached, adults fly away looking for weakened trees in which they lay eggs and start a new generation.
Interactions among elms, DED fungi and elm bark beetles
The mechanisms of pathogen transmission were deeply investigated in the past ([139]). Some authors considered the wind to be important in spreading fungal spores from infected plants to tissues of healthy trees exposed by wounds or pruning ([142], [122]). This hypothesis, however, was soon disproved, as anemochoral dispersion of the spores would be too casual and generic, and could not ensure ideal growing conditions to the pathogen for a rapid development ([61]).
Other authors suggested the rain as the main responsible of the conidial spread, allowing them to reach the leaf stomata ([112]). However, in 1927 Marchal proposed that bark beetles could be vectors of O. ulmi s.l., as they migrate between infected and healthy elms ([80]). Although the pathogen may be transmitted effectively in several ways ([112], [142], [122]), elm bark beetles belonging to the genus Scolytus were found to be the most effective pathogen vectors ([80], [50], [66], [67], [28], [139], [137], [6], [44], [8], [42], [43], [41]). The definitive demonstration of Marchal’s theory was provided by Fransen ([50]) and Fransen & Buisman ([52]), who showed pathogen presence and proliferation in the short galleries bored by elm bark beetles in healthy plants during maturation feeding. Many other vectors were later identified, mainly mites and insects living on elms, but none as effective as bark beetles ([66], [67], [28]).
Elm bark beetles originally had a mutualistic ectosymbiosis with the indigenous saprotrophic fungus O. quercus, previously known as the hardwood biological species group, or OPH group, of O. piceae ([16]). Later, the arrival in Europe of the congeneric O. ulmi s.l., a fungus having niche requirements similar to O. quercus but far more aggressive, caused the complete replacement of the endemic fungus. The new association became an effective DED transmission pathway, with devastating consequences for elm survival.
The success of the pathogen-insect interactions is mainly due to the characteristic reproductive behavior of the elm bark beetles, and largely depends on the occurrence of O. ulmi infected trees. The feeding activity of callow adults emerged from infected trees, and carrying pathogen conidia on their bodies, contaminates healthy trees and facilitates consequent development and movement of the pathogen within their wood vessels ([52], [60], [61], [56], [24], [141], [139]). Basset et al. ([6]) showed that a contact for at least 72 hours between infected beetles and xylem can be sufficient for the pathogen transmission. This is known as the pathogenic phase of the disease ([72], [59]). The canopy of every tree may host several dozen callow adults, and the process can be repeated for several years; thus, healthy trees growing close to elms infected by O. ulmi s.l. and infested by elm bark beetles are exposed to a very high risk of infection. This transient phase is very important for pathogen spread, as it is the only way for the fungus to reach and infect isolated trees. Maturation feeding and pathogen infection weaken trees that will become attractive for mature reproductive adults of the following insect generations, which will be looking for dying (i.e., diseased) elms where to lay eggs in the bark of trunk and main branches. During bark infestation and excavation of the mating galleries, the mature adults again infect the hosts with the conidia carried on their tegument, fulfilling their role as vectors for a second time. Bark colonization by insects and pathogen on already infected elms (from the twigs) is known as the saprophytic phase of the disease ([72], [59]). In this respect, maternal galleries and pupal chambers are an ideal micro-environment for both fungal growth and sporulation ([139]). Interestingly, in this phase two fungus isolates having different origins, one from previous maturation feeding and the other from the more recent bark colonization, may meet in the same tree. This gives to the pathogen more chances of sexual reproduction, being O. ulmi s.l. an obliged outcrossing fungus. The emerging beetle offspring developed in the phloem of infected trees will become new vector of fungal conidia and the dispersal cycle will start again ([139]). Not every wound due to maturation feeding results, however, in pathogen transmission ([51]). Parker et al. ([95]) reported that 13% of all cases resulted in tree infection, whereas Webber & Brasier ([139]) found that about 30% of feeding wounds were infected by O. ulmi s.l. Xylem infection may be a result either of a primary and direct spore transfer from the beetle into the xylem vessels or, more likely, of a secondary infection due to earlier pathogen colonization of the feeding wound followed by subsequent growth into the xylem tissues ([23]).
Host resistance
There is considerable variation among elm species in host resistance to DED. Asian elms are usually the most resistant ([120], [136], [119]), while most North American elms are highly susceptible and European species are moderately to very susceptible ([57], [32]).
Compared with susceptible elms, both at species and individual level, naturally resistant elms show some peculiar anatomical features such as smaller ([83], [117], [123], [124], [126]) and shorter vessels ([33], [38], [135]), smaller pit membrane diameter and pit aperture area, lower pit membrane abundance per vessel- wall, smaller ray width and ray tangential area ([81]). Anatomical features, however, show much variability also between and within species. For instance, the mean maximum vessel length and diameter are significantly higher in U. minor than in U. minor x pumila, and these differences increase with age ([82]).
Induced resistance to DED is associated strictly with the host’s capacity to quickly localize the infection, preventing the pathogen from spreading in the vascular system ([117]) and reaching the cambium ([115], [13]). Reactions taking place as a consequence of the infection may include vessel closing by tyloses, embolisms, accumulation of pectin and hemicelluloses ([35], [114], [92], [105]), synthesis of chemicals such as phytoalexin-like sesquiterpenes ([68], [31], [129]), and formation of histological barriers typically containing phenols and suberin ([103], [104], [93], [94], [37]).
Elm susceptibility to DED shows strong seasonal variation in both resistant and susceptible species. The period of highest susceptibility and its duration, i.e., the period during which trees can easily become infected varies greatly among elm species and even among elm populations and experimental conditions ([4], [5], [100], [101], [89], [90], [121], [134], [120], [109], [125]). According to studies correlating susceptibility to O. ulmi s.l. with seasonal host phenology ([100], [89], [90], [133], [125], Santini & Ghelardini, unpublished data), the period of highest susceptibility coincides with the seasonal maximum growth rate, recorded at the beginning of leaf expansion and formation of large spring xylem vessels, all genotype-dependent characters. The decrease in susceptibility observed from spring to late summer is also correlated to the seasonal changes in wood anatomy, i.e., the transition from early wood, characterized by large vessel elements, to late wood, made up of smaller cells with thicker cell walls ([102], [125]). Pathogen development is, hence, highly dependent on the host’s seasonal morphogenesis and cambial activity, as well as on the pattern of longitudinal and radial growth of the host plant. Because efficient wood compartmentalization forces the plant to keep building new barriers, which limit the accessibility of its own reserves in the stem ([13]), the highest susceptibility occurs with low energy reserves, high growth rate, not fully efficient photosynthesis and large vessels ([53]). Since plant physiological processes are seasonally regulated, and elms are exposed to fungus inoculation only in a transitory and short phase of the vector life history (twig-crotch feeding phase), the infection will occur only if insect feeding phase and time of host susceptibility overlap. Thus, an asynchrony between these phenological phases may allow trees to avoid infection, representing a particular form of disease-escape resistance. For instance, although inoculated in the same day in central Italy, southern European clones of U. minor showed fewer disease symptoms than northern ones, with a significant direct relationship between disease severity and bud burst date ([109]). As southern clones cultivated in central Italy flushed significantly earlier than northern ones ([54]), this suggests that, at the inoculation date, the southern clones had already passed the period of maximum susceptibility, which for European elms begins 40-50 days after bud burst and lasts for a number of days related to environmental conditions and genotype ([134], [120], Santini & Ghelardini, unpublished data). Southern clones completed the formation of the large spring vessels and the production of latewood early, reducing their risk of infection ([102], [125], Santini & Ghelardini, unpublished data). These studies provided new information on susceptibility to DED, suggesting that early flushing is a mechanism of DED avoidance based on asynchrony between maximum susceptibility period and inoculation time ([109]).
Variations in tree susceptibility related to different growth rhythms, caused by either seasons or differences in elm populations or clones ([131]), fit well with the growth-differentiation balance hypothesis ([63]). This hypothesis provides a framework for predicting how plants balance resource allocation between differentiation-related processes and growth-related processes over a range of environmental conditions, as it is known that resources cannot be allocated to both functions simultaneously ([74]). Resource availability is high in spring, when favorable temperatures are combined with plentiful water supply, a condition lasting for a short time in the Mediterranean climate until the early, hot and dry summer occurs. At this time of the year, elm energy reserves are already exhausted by the flowering process that occurs in late winter, and by the construction of the new porous ring, which has to provide nutrients for the expanding photosynthetic surfaces. Growing meristems behave as strong photosynthetic sinks, provisioned by carbon sources that include neighboring mature leaves ([79]) and also newly formed leaves. No resources are dedicated to defense, which make elms especially susceptible to DED during spring, exactly in the period of the vector’s inoculative phase. As time passes, resource availability and growth rate decrease so that photosynthates can be allocated to differentiation. This process could explain why inoculations by the second beetle generation in summer - which furthermore accounts for a lower percentage of vectors ([42]) - is less effective or totally ineffective in pathogen transmission compared to the first generation in spring. Because in early-flushing elms these phenological and physiological events occur earlier in spring, the emerging beetles will feed on trees having already mature leaves able to allocate carbohydrates to secondary metabolism, and therefore better defend against pathogen infections. In this period early-flushing elms are also already producing latewood that, being constituted of small and scattered vessels with a greater proportion of fibers, is less suitable for spore diffusion.
Challenges for the nearby future
Although we are learning much about insect-pathogen interactions and transmission mechanisms, many topics still deserve additional attention.
The systematic of species belonging to the genus Scolytus is one of the most complicated amongst bark beetles ([85], [99]). The systematic position of elm bark beetle species must be better defined by sequencing specific regions of the DNA in phylogenetic studies, to provide a correct identification of the Scolytus species, as already done with other scolytid genera ([26]). Genetic improvement programs are also required on the host trees aiming to select new European elm clones with higher levels of DED resistance, based, for instance, on the bud-burst precocity ([109]). This resistance could be exploited by specific studies exploring how different climatic conditions affect budburst date and growth rhythm, and the natural history (i.e., phenology and voltinism) of Scolytus spp. ([53]). Early flushing European elm clones could be selected to obtain elms avoiding natural infection through an asynchrony between host and insect phenology, both of which are regulated by temperature ([116], [113], [55]). Although many studies were conducted to select elm species and clones resistant to O. ulmi s.l. ([119], [87]), no effort was made to identify mechanisms of combined resistance to both DED and elm bark beetles. Concerning pathogens, considerable progress has been made in genetic and systematic knowledge of the Ophiostoma species associated with elm bark beetles ([69]). The significance of the association, however, is still matter of discussion, especially regarding the role of fungi - and spore load carried by the beetles - in exhausting tree defenses, the relations between fungus pathogenicity and beetle aggressiveness, and their role in the tree killing process.
There have been very few morphological studies on the Ulmus-Ophiostoma-Scolytus system. Concerning insects, the structure and ultra-structure of the mycangia or mycetangia on the bark beetle tegument was deeply investigated in the past ([45], [46], [47], [48], [49], [7], [10], [11], [73]), but not specifically in the Scolytus species except for a few preliminary observations ([39]). The host-pathogen interactions leading to DED symptoms were recently analyzed in vitro by histo- and cyto-chemical tests. Callus cultures of susceptible U. americana were inoculated with the highly aggressive pathogen O. novo-ulmi. Inoculated callus tissues were then compared with water-treated callus tissues using TEM and SEM light microscopy. New aspects of these interactions were described, including histological observations - for the first time in plant callus cultures - on suberin, with its typical lamellar structure, and the intracellular presence of O. novo-ulmi ([2]). Other SEM applications were the description of the typical tyloses induced by the pathogen within the spring vessels of infected elms, or the tissue invasion process operated by blastoconidia ([34], [36]). However, the studies were limited to a simple description of the host reactions to pathogen attacks, and did not investigate the structure of the reaction tissues.
Chemical communication is of crucial importance to manage insect populations, DED epidemics and their damage to elms. Gaps in our knowledge concern, for instance, the selection mechanisms driving callow adult beetles in the plant choice for maturation feeding. In this phase, tree attractiveness to elm bark beetles is of primary importance for a susceptible plant to be infected by O. ulmi s.l. Therefore, once the chemical attractant has been identified a specific marker assisted tree-breeding program may be developed for both native European and American elm species. Such a tree-breeding strategy would avoid the use of Asian species as source of resistance, as they could become invasive, posing a threat to the native biodiversity ([145], [22]).
Because the number of papers published on different Scolytus species is directly related to the economic importance of the organisms, only biology and ecology of the most harmful species were investigated, while very little is known about the less common species - such as S. laevis, S. sulcifrons and S. triarmatus - which, however, are recently showing an increasing importance as DED vectors. There is hence a dramatic lack of detailed information on less damaging species, although it would be very useful for comparison and generalization, and would certainly also lead to a better understanding of the reasons for the aggressiveness of the dangerous species. There is, therefore, a wide and regrettable gap in our knowledge concerning secondary, or less common, Scolytus species.
Research is also needed about the environmental factors affecting elm bark beetles and pathogen performance and their adaptation to new and changing environments, especially in relation to global change. New problems can emerge from climate change that modify the geographic distributions of pests, pathogen and hosts. Although the northward and upward spread of both DED epidemics and some Scolytus species (e.g., S. multistriatus in southern Sweden (Faccoli 2003, pers. obs., - or at higher altitudes on Apennines, [98]) was recently associated to global warming, considerable knowledge gaps exist regarding the effects of these factors on insect aggressiveness and fungus pathogenicity in both Europe and North America. Very little information is also available regarding the relations between global change and tree resistance, although this topic represents a considerable component of modern researches. Characterizing the physiological status of trees in relation to their susceptibility is also needed prior to building risk prediction models in non-epidemic areas, such as Northern Europe and America. What will the responses be of elms, DED and elm bark beetles to increasing temperatures? In U. minor a large intra-specific variation in timing of bud burst was found to be mainly dependent on geographic origin. The bud burst date was directly related to latitude and altitude, i.e., temperature, with a greater chilling requirement for dormancy in northern areas or highlands than in southern or lowlands ([54]). It can be hypothesized, for instance, that under climate warming European elms would start to flush earlier in most parts of their natural range, maybe escaping the pathogen infection ([110], [55]), but how the insect phenology will change in front to a warmer climate? The few models describing the population dynamics of pathogen and insects consider climatic conditions only marginally. They are generally never focused on changing environments, nor on the prediction of future impacts in new areas or on new elm species, as recently observed in Colorado following the introduction of the banded elm bark beetle, S. schevyrewi Semenov from Siberia ([65]). Studied carried out at larger geographic scale should hence lead to more dynamic models.
Some factors suspected to be of seconda- ry importance in the epidemic dynamics of DED were in the past largely underestimated. The case of natural enemies of the elm bark beetles should be, for instance, valuable for further research. Similarly, apart a few studies concerning the unattractiveness of U. laevis toward the Scolytus beetles ([108]), neither the effects of intra- and inter-specific variations in the nutritional quality of elms nor the tree constitutive defenses against elm bark beetles were considered. Research on genetic variations in beetle/pathogen populations, especially by comparing endemic and epidemic areas, should also provide very useful information on their role in epidemic dynamics. Moreover, the possible occurrence and importance of transmission mechanisms different than those due to elm bark beetle maturation feeding or the occurrence of other possible vectors were incompletely explored ([29]). For instance, the high efficiency of S. scolytus in spreading DED was recently suggested to be partly due to its association with two mites, Proctolaelaps scolyti Evans and Tarsonemus crassus (Schaarschmidt), and the hyperphoretic spores of O. novo-ulmi they carry ([88]). Another challenge concerns the emergence of new problems resulting from the rapid increase of international trade among continents, leading to the accidental introduction of new vector species, or new highly pathogenic strains, or new highly susceptible elm species in gardens and public parks. Introductions of new pathogens or vectors, for instance, may lead to new and unexpected associations, able to increase the fitness of both organisms with tremendous consequences for the host populations, as recorded in other insect-fungus associations ([9], [76]).
Conclusion
The processes that regulate the current beetle-fungus symbiosis remain poorly understood. The relationships between elm bark beetles and O. ulmi s.l. depend on several factors, such as the climatic and environment characteristics, and the interactions between the components of the biotic community, including, for example, the d-factor, a citoplasmically transmitted virus disease of Ophiostoma ulmi s.l. ([15]). Commonly to other bark beetle-fungus symbiosis, all these factors play important roles in determining composition, fidelity and longevity of the association between beetles and DED fungi ([118]).
Insects play a role of primary concern in transmitting diseases to elms, like for instance several species of leaf-hoppers identified as vectors of the elm yellow phytoplasma ([25]).
There are thus several aspects that still deserve to be studied for a better understanding of the pathogen-insect interactions. In addressing these issues, a holistic approach is highly recommended, taking into account all the many factors affecting pathogen transmission and disease spread.
References
Gscholar
Gscholar
Gscholar
Gscholar
Gscholar
Gscholar
Gscholar
Gscholar
Gscholar
Gscholar
Gscholar
Gscholar
Gscholar
Gscholar
Gscholar
Gscholar
Gscholar
Gscholar
Gscholar
Gscholar
Gscholar
Gscholar
Gscholar
Gscholar
Gscholar
Gscholar
Gscholar
Gscholar
Gscholar
Gscholar
Gscholar
Gscholar
Gscholar
Gscholar
Gscholar
Gscholar
Gscholar
Gscholar
Gscholar
Gscholar
Gscholar
Gscholar
Online | Gscholar
Gscholar
Online | Gscholar
Gscholar
Gscholar
Gscholar
Gscholar
Online | Gscholar
Gscholar
Gscholar
Gscholar
Gscholar
Gscholar
Gscholar
Gscholar
Gscholar
Gscholar
Gscholar
Gscholar
Gscholar
Gscholar
Gscholar
Gscholar
Gscholar
Gscholar
Gscholar
Gscholar
Gscholar
Authors’ Info
Authors’ Affiliation
Institute of Sustainable Plant Protection, CNR, v. Madonna del Piano 10, I-50019 Sesto Fiorentino (FI - Italy)
Department of Agronomy, Food, Natural Resources, Animals and Environment (DAFNAE), Agripolis, v.le dell’Università 16, I-35020 Legnaro (PD - Italy)
Corresponding author
Paper Info
Citation
Santini A, Faccoli M (2015). Dutch elm disease and elm bark beetles: a century of association. iForest 8: 126-134. - doi: 10.3832/ifor1231-008
Academic Editor
Marco Borghetti
Paper history
Received: Jan 02, 2014
Accepted: May 18, 2014
First online: Aug 07, 2014
Publication Date: Apr 01, 2015
Publication Time: 2.70 months
Copyright Information
© SISEF - The Italian Society of Silviculture and Forest Ecology 2015
Open Access
This article is distributed under the terms of the Creative Commons Attribution-Non Commercial 4.0 International (https://creativecommons.org/licenses/by-nc/4.0/), which permits unrestricted use, distribution, and reproduction in any medium, provided you give appropriate credit to the original author(s) and the source, provide a link to the Creative Commons license, and indicate if changes were made.
Web Metrics
Breakdown by View Type
Article Usage
Total Article Views: 75221
(from publication date up to now)
Breakdown by View Type
HTML Page Views: 56008
Abstract Page Views: 6201
PDF Downloads: 11257
Citation/Reference Downloads: 100
XML Downloads: 1655
Web Metrics
Days since publication: 3999
Overall contacts: 75221
Avg. contacts per week: 131.67
Article Citations
Article citations are based on data periodically collected from the Clarivate Web of Science web site
(last update: Mar 2025)
Total number of cites (since 2015): 89
Average cites per year: 8.09
Publication Metrics
by Dimensions ©
Articles citing this article
List of the papers citing this article based on CrossRef Cited-by.
Related Contents
iForest Similar Articles
Review Papers
Avoidance by early flushing: a new perspective on Dutch elm disease research
vol. 2, pp. 143-153 (online: 30 July 2009)
Review Papers
Genomics of the Dutch elm disease pathosystem: are we there yet?
vol. 8, pp. 149-157 (online: 07 August 2014)
Research Articles
Comparison of commercial elm cultivars and promising unreleased Dutch clones for resistance to Ophiostoma novo-ulmi
vol. 8, pp. 158-164 (online: 07 August 2014)
Research Articles
Monitoring of the incidence of Dutch Elm Disease and mortality in experimental plantations of French Ulmus minor clones
vol. 15, pp. 289-298 (online: 29 July 2022)
Research Articles
Seven Ulmus minor clones tolerant to Ophiostoma novo-ulmi registered as forest reproductive material in Spain
vol. 8, pp. 172-180 (online: 13 August 2014)
Research Articles
Genetic variation of Fraxinus excelsior half-sib families in response to ash dieback disease following simulated spring frost and summer drought treatments
vol. 9, pp. 12-22 (online: 08 September 2015)
Research Articles
Temporal development of collar necroses and butt rot in association with ash dieback
vol. 10, pp. 529-536 (online: 05 May 2017)
Research Articles
Genetic variation and heritability estimates of Ulmus minor and Ulmus pumila hybrids for budburst, growth and tolerance to Ophiostoma novo-ulmi
vol. 8, pp. 422-430 (online: 15 December 2014)
Research Articles
Seasonal dynamics of Cryptostroma corticale conidial spread
vol. 18, pp. 79-86 (online: 17 April 2025)
Research Articles
Outcome of Ceratocystis platani inoculations in Platanus × acerifolia in relation to season and inoculum dose
vol. 9, pp. 608-617 (online: 17 March 2016)
iForest Database Search
Search By Author
Search By Keyword
Google Scholar Search
Citing Articles
Search By Author
Search By Keywords
PubMed Search
Search By Author
Search By Keyword