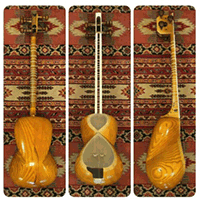
Reversible and irreversible effects of mild thermal treatment on the properties of wood used for making musical instruments: comparing mulberry to spruce
iForest - Biogeosciences and Forestry, Volume 15, Issue 4, Pages 256-264 (2022)
doi: https://doi.org/10.3832/ifor4074-015
Published: Jul 20, 2022 - Copyright © 2022 SISEF
Research Articles
Abstract
Thermal treatments can be considered as an accelerated ageing, bringing partly similar changes in properties as naturally aged wood. Thermal treatment was applied on white mulberry (Morus alba L.), a dominant species for making musical instruments from middle-East to Far-East, to investigate the effects on the vibro-mechanical and physical properties of this wood, and the results compared to previously published data on spruce (Picea abies Karst.) as a reference for the soundboard of Western string instruments. Thermal treatment (TT) at 150 °C and 0% of relative humidity was applied to five analogous groups of specimens with five different durations (2.5, 8, 24, 72, 261 hours). Humidity re-conditioning of specimens was done to explore the reversibility of TT effects. Physical and vibrational properties such as specific gravity (γ), equilibrium moisture content (EMC), CIELab colorimetric values, specific modulus of elasticity (E’/γ) and damping coefficient (tanδ) in longitudinal (L) and radial (R) directions, have been measured after stabilisation of samples in standard conditions (20 °C, 65% RH), before and after TT and then after re-conditioning. Untreated mulberry had a low EMC, very low L/R anisotropy and low E’L/γ, and relatively low tanδ. Weight loss (WL) and CIELab values evolved similarly during TT for mulberry and for previous results on spruce, however, their EMC and vibrational properties were affected differently. This could be explained in part by the low anisotropy of mulberry, and in part by its particular extractives. The parts of irreversible effects, linked to chemical modification or degradation, and of reversible effects, linked to physical configuration, were different between mulberry and spruce. The applied treatments did not bring permanent “improvements” in vibrational properties of mulberry, yet its colour appearance was enhanced.
Keywords
Anisotropy, CIELab, Morus alba, Musical Instruments, Reconditioning, Thermal Treatment, Vibrational Properties
Introduction
Wood has special mechanical and acoustical properties, and this had been traditionally used in the field of musical instruments making ([14]). As it has generally been considered in scientific studies, mainly based on Western/globalised cultures, suitable wood for making stringed musical instruments would often be defined by higher modulus of elasticity (E) per specific gravity (γ), and by lower damping by internal friction (tanδ) than other woods ([38]). In this context, the most studied wood species is spruce (Picea abies Karst.) commonly used for the soundboard of string instruments in the Western culture. However, different cultures use different wood species to make traditional musical instruments, with sometimes very different material properties ([3]). For example, white mulberry (Morus alba L.) is a dominant species used by craftsmen for making stringed musical instruments in Iran (see Fig. S1 in Supplementary material), Central Asia, and Japan ([48], [20], [44], [46]). Yet mulberry wood has nearly opposite vibro-mechanical properties to spruce when the species used for string instruments among the world are compared ([3]). Today, the variety of instruments in different geographical and cultural regions can be attributed to the geographical, historical and cultural course of each region. Besides, it can be thought that there is a relationship between access to wood species in a geographical area and the selection of that species for use in the construction and evolution of musical instruments. Therefore, it can be deduced that a musical instrument consist of two main feature regarding wood species; acoustical aspects and cultural-geographical aspects ([3]). Accordingly, the interaction between the different wood species and acoustical properties, variations of musical instrument structures, and differences in musical tastes across different cultures lead to wood selection.
Some instrument makers sometimes use traditional (hygro-) thermal pre-treatments ([45]). Currently, there is a growing interest on research and development studies in a more industrial scope, for general uses of wood of course, but also specifically for instrument making ([47], [40], [49], [22]). Thermal and hygrothermal treatments are relatively eco-friendly processes involving temperature and relative humidity, developed to improve biological durability and dimensional stability by reducing hygroscopicity ([18], [43], [17]). They also have an effect on darkening wood colour, that could be made to profit for imitating either endangered tropical species ([22]) as natural heritage, or ancient wood from artefacts of cultural heritage ([25], [24]).
It is often argued that musical instrument makers and artisans would consider aged wood as a material with improved physical and acoustical properties such as reduced moisture expansion and damping ([14], [35]). Two interesting studies show some “improvement” in the acoustical properties of long-term aged pine wood ([28]) or spruce wood ([37]). However, when actually asking artisan violin makers, they consider long-term (decades or centuries) ageing to be more beneficial for aesthetical properties than for physical-acoustical ones, while they consider short-term (years or few decades) ageing/seasoning to be more beneficial for physical-acoustical properties ([11], [12]).
(Hydro-) thermal treatments might be considered as a kind of accelerated ageing, since raising temperature accelerate chemical changes which occur during natural ageing ([25], [24], [52]), and accelerated aged wood can be, at least partially, similar to naturally aged wood, regarding increased stability and rigidity as well as appearance and colour ([34], [35], [14]).
Hydrothermal treatments have been studied in mild conditions and in severe ones ([15], [16]). The effect of thermal modification under saturated steam at three different temperature (120 °C, 150 °C and 180 °C) on the mechanical and physical properties of pine has been investigated and compared with the thermal modification under superheated steam condition in high pressure state ([41]). It has been concluded that thermal treatment under saturated steam condition in a lower temperature and shorter duration of treatment can give better or the same mechanical properties than superheated steam status, suggesting that in saturated conditions the temperature can be reduced to 150-180°C rather than 180-210°C which is typically used for industrial thermal treatments. Besides, Marcon et al. ([24]) studied two thermal modification processes (Thermal and Hydro-thermal modification) by applying time temperature superposition method. They presented a master curve with the possibility of shift based on the temperature of modification process. The dry mass loss during both ventilated oven treatment (T) and saturated steam one (HT) has been investigated, while the reference temperature of 150 °C has been selected for both processes. According to their studies, thermal treatments in dry conditions require higher temperature and/or longer durations to obtain similar results as in saturated conditions.
The chemical irreversible reactions such as recrystallization, degradation and cross-linkage of wood components are considered some important effects of hygrothermal treatments ([43], [17]). Chemical changes of amorphous polymers during heat treatment are presented as irreversible consequences of hygrothermal treatment ([31]). The hypothesis for the decrease in swelling is the decrease of accessible hydroxyl groups. Nonetheless, the reduction in hygroscopicity has been found to be temporary and moistening in high humidity led to recovery in hygroscopicity ([33]). The reversibility of physical and acoustical changes has been studied ([31], [37], [35]) and partial recovery is found for both naturally aged and thermally treated wood. Karami et al. ([21]) have also studied the effects of mild hygrothermal treatments on the acoustical properties of spruce wood used for making musical instruments. A significant part of the changes after mild hygrothermal treatments were reversible (recovered by reconditioning), but irreversible changes remained, with different amplitudes depending on the direction of anisotropy.
A majority of studies on thermal treatments concern softwoods, whereas their effects could be significantly different when applied on various hardwoods ([26], [22], [6]). The acoustical consequences of low-temperature (<100 °C) hygro-thermal pre-treatments traditionally applied by instrument makers have been studied on mulberry (Morus alba L.) wood by Se Golpayegani et al. ([45]), with some interactions between treatments and extractives. In untreated mulberry wood, extractives amount to approx. 12%, and they globally lower the damping coefficient of this wood, but with contrasted effects depending on the considered fraction of extractives ([44]). Chemical properties of Morus nigra are significantly dependant on the genotype, the age and the mutual interaction of both these factors ([13]).
In the present study, a mild thermal treatment in oven dry condition (0% relative humidity) has been investigated for white mulberry (Morus alba L.). This treatment being considered as an accelerated ageing or pre-treatment process. The effects were investigated on the physical, colorimetric and acoustical properties of this species. As well, the reversibility or irreversibility of changes occurred by thermal treatments have been studied after applying a reconditioning. Although the wood used for musical instrument is not supposed to be subject to such conditions as applied in experimental studies, yet the repetitive application of moist conditions could provoke a partial reconditioning, so that any beneficial modification for musical application would suffer from it. This work compared the effects of mild thermal treatment (TT) on physical and vibrational properties of mulberry (Morus alba L.) to those of spruce from previous studies, both being two emblematic wood species for making musical instruments but in different cultures. Besides the cultural aspects, the goal was also to compare the effect of TT on a well-studied softwood, and on a lesser-studied hardwood. A hypothesis was that the peculiar native properties and extractives’ content of mulberry could result in different impacts of TT and recovery.
Material and methods
Studied species and preparation of specimens
Experiments of the current study focused on white mulberry (Morus alba L.) species, which is a major material for making Iranian stringed musical instruments (Tar, Setar - Fig. S1 in Supplementary material). This species is a semi porous hardwood with the density ranging between 450 -800 kg m-3. This wood has a yellowish-brown heartwood and pale yellow sapwood, and usually straight grain. Wood specimens were brought from Karaj, a city near Tehran, Iran. A professional musical instrument maker (Mr. Samad Zare) selected the wood and pre-cut the specimens. All the specimens were from the same tree according to typical quality-grading criteria. Some 162 specimens were cut in two directions, 81 longitudinal (12×2×150 mm3, R×T×L) and 81 radial (120×2×12 mm3, R×T×L) specimens. The quality of the specimen’s preparation is essential when studying vibrational properties ([1]).
Then, results on Mulberry from the current study were compared to previously obtained results on Spruce (Picea abies Karst.) following a comparable protocol ([21]).
Spruce specimens from a former study ([21]) were selected for comparison, as this species is the material for soundboards of European musical instruments. There were specimens in the longitudinal (L) direction and in the radial (R) direction. A group was intended for the given modalities of treatment (150 °C and 0% relative humidity) and 1 reference group remained as a control.
Sampling: creation of similar groups
The effect of thermal treatment duration was studied by applying thermal treatments to different groups of wood specimens. To assess the effect of treatment duration, it is important that the groups of specimens have similar distribution of initial properties, so that the observed differences are not due to sampling effects. The values of EMC (Equilibrium moisture content), density, E’/γ (specific dynamic elastic modulus) and tanδ (damping coefficient) were measured before any thermal treatment on 81 wood specimens in each direction (L and R). An ad-hoc algorithm (written with Excel and Visual Basic macros) was used to make six groups of six specimens, having means and standard deviations as similar as possible for each measured parameter. The algorithm is stochastic, based on the minimisation of a score combining the between-group variance of means and standard deviations for the different parameters. Starting from a manual initial sampling, the algorithm tries random exchanges between groups, and validates them if the score is improved, until no more improvement is obtained. Fig. 1 illustrates the quality of the sampling, on L specimens, showing that groups have a similar mean and standard deviation for the 4 matching parameters considered.
Fig. 1 - Sampling into groups (6 specimens each: small coloured circles) of comparable average and variance (error bars = standard deviation) over several properties simultaneously, from a batch of untreated specimens with variable properties. (a) Equilibrium Moisture Content and specific gravity; (b) longitudinal damping coefficient and specific modulus of elasticity. The different colours indicate the 6 matched groups used in this study. The remaining open circles correspond to the whole original batch of specimens, but that were not included in the 6 groups created for further steps of the study.
Steps of measurements
After being pre-cut in Iran, wood samples were brought to France and finely machined to final dimensions. Specimens were then oven dried, first at 60 °C for two days then at 103 °C for 3 hours, to prevent the damage that could result from drying too roughly. After oven drying, mass and dimensions of specimens were measured and they were conditioned in a climatic room in standard conditions to be stabilised and reach to equilibrium moisture content (EMC). After at least 3 weeks of stabilisation in climatic room (at 20 °C and 65% RH), physical and vibrational properties were measured in the same room, as vibrational properties are highly dependent upon moisture content and its variations ([30], [7], [8]). Specimens were placed in a dry oven for mild thermal treatment and intermediate data were measured every day for oven-dry dimension and weight. Final values of these properties were also measured at the end of each treatment. Then, specimens were stabilised again (at least 3 weeks) at 20 °C and 65% RH to compare untreated and treated physical, colorimetric and vibrational properties. Finally, a cycle of humidity re-conditioning was applied before measuring the last values. Control groups underwent the same conditioning and measurement steps as treated groups, except that they were not submitted to any thermal treatment.
Thermal treatment
After primary measurements, a thermal treatment (TT) was applied on 6 groups of specimens (including control groups), with 6 repetitions in each group (6L + 6R specimens). A mild thermal treatment at 150 °C and 0% RH was applied in a dry oven for 5 groups with different durations, evenly distributed in a logarithm scale: 2.5, 8, 24, 72 and 261 hours (2.5h, 8h, 1d, 3d, 9d) following the procedure proposed by Matsuo et al. ([25]), well suited to the study of thermally activated processes. Such conditions, also applied to spruce by Karami et al. ([21]), are expected to modify material properties without provoking too much degradation. For each step, one group of specimens was taken out and mass and dimensions were measured. Then the specimens were placed in a climatic room for 3 weeks to be stabilised in standard conditions (65% RH and 20 °C). After stabilisation, mass, dimension, vibrational and colorimetric properties were measured.
Physical measurements
Weight and dimensions of specimens were measured, at “standard air-dry” and at oven-dry states, before and after treatments to calculate oven-dry weight loss (WL0), specific gravity (γ), equilibrium moisture content (EMC) and partial shrinkage (pS). WL0 was calculated as (eqn. 1):
where m0u and m0t are oven-dry mass of untreated and treated wood, respectively. After stabilisation of specimens in standard condition (at 20 °C and 65% RH), weight and dimensions of specimens were measured and specific gravity (γ) was calculated as (eqn. 2):
where m and V are the mass and volume of the wood specimen and ρH2O is the density of water. The equilibrium moisture content (EMC), at 20 °C and 65% RH, was calculated as (eqn. 3):
where m65% is the mass of the stabilised wood specimens (at 20 °C and 65% RH) and mo the oven-dry mass.
Colorimetric measurements
Colour properties were measured with a spectrophotometer “spectra-guide sphere gloss” (model 6834, BYK, Wesel, Germany), with a diameter of aperture of 10mm. Standard illuminant D65 and standard observer curve of 10° were used. Data were collected in the CIELab system, where L* (lightness) is 0 for black, 100 for white, axis a* ranges from green (-a*) to magenta (+a*) and axis b* ranges from blue (-b*) to yellow (+b*). Data are also expressed in the more intuitive CIELCh system (eqn. 4, eqn. 5):
where C* is the chromaticity (intensity of colour) and h° the hue angle.
Vibrational properties measurement
The principle of non-contact forced-released vibration of free-free bars ([32]) was used for vibration test. The experimental methodology, device and system settings are described in Brémaud et al. ([4]). Damping coefficient, which is expressed as tanδ, was calculated as the inverse of the “quality factor” Q, or half-power bandwidth determined by a frequency sweep centred around the resonance frequency of the 1st bending mode. According to Bernoulli equation (considering length and thickness of specimens), the specific dynamic modulus of elasticity (E’/γ) was obtained from the first resonance frequency. Vibrational properties were defined for the L (E’L/γ and tanδL) and R (E’R/γ and tanδR) directions. Frequency range was 100-500Hz over all R and L specimens. Each specimen was measured with 3 repetitions.
Reconditioning
After performing all measurements, samples were reconditioned to determine the reversible or irreversible effect of TT. To verify the reversibility of changes after treatments, a recovery test needs to be performed. It can be achieved by humidification of specimens at high relative humidity. A closed hermetic box was used, which was placed in a cold room. Specimens were placed between water-soaked cotton wool and then placed in this box for 3 weeks. After this step all the specimens were placed in a climatic room for 3 weeks to be stabilised (20 °C and 65% RH). After stabilisation, the physical and mechanical properties were measured as described above.
Results and discussion
Properties of untreated mulberry wood
The studied material of white mulberry wood had a specific gravity (γ) of 0.49 on average (from 0.43 to 0.53) and an equilibrium moisture content at 20 °C and 65%RH (EMC) of 9.3 ± 0.4%. These values can be compared to previously published studies by Se Golpayegani et al. ([44], [45]), who reported a significantly higher γ of 0.54 on average (from 0.45 to 0.60) and a slightly lower EMC of 8.0%. The wood used in the present study had narrower growth ring and consequently lower late wood percentage, which can explain the difference considering the ring-porous nature of this species.
In Fig. 2, the values of tanδ versus E’/γ for mulberry specimens in R and L directions are shown. Most of the values of specific modulus in L direction are remarkably close to those for R direction: the anisotropic ratio between E’L/γ and E’R/γ is of only 2.3 on average (from 1.6 to 3.3). This is a surprising result, because the L/R ratio in specific modulus is of 8 in average over many hardwood species ([2]). Reduced anisotropy for white mulberry (L/R ratio of moduli of 4) is already reported ([44]), but the anisotropy for the specimens in the current study was, interestingly, even lower. In a comparison of several studies on vibrational properties of mulberry ([45]), the lowest values of E’L/γ were found for a wood considered as of “superior quality” for instruments ([48]). It can be suggested that the extremely low value of E’/γ in L direction, accompanied by rather high values in R direction, may reflect an exceedingly high microfibril angle. Indeed, such low values of E’L/γ (6.9 GPa on average) as found here are only reported for compression wood, either of softwoods ([5]) or of the atypical hardwood Buxus, an angiosperm forming compression wood ([10]), both with microfibril angle in the range of 30° to 45°. Another hypothesis would be that, as Morus is a ring porous species ([20]), a very light wood as the one studied here would include a high proportion of vessels and parenchyma compared to that of fibres. This is consistent with previous observations that mulberry specimens with narrower rings (i.e., for this ring-porous wood, with wider proportion of vessel-rich earlywood and small proportion of fiber-rich latewood) tend to have lower values of both γ and E’L/γ ([45]). Across several studies (present results, [42], [44], [45]) there is a positive correlation between γ and E’L/γ, although E’L/γ is theoretically determined at cell-wall scale and thus is generally little dependent of specific gravity ([29]).
Fig. 2 - Relationship between damping coefficient and specific modulus of elasticity, in L (filled symbols) and R (open symbols) directions, for studied mulberry (green circles) compared to previous studies (brown circles - [44], [45]) and to spruce (triangles - [21]). The standard trends for hardwoods and/or softwoods, along L and other anisotropy directions, are shown for reference ([38], [39], [4]).
Apart from this atypically low anisotropy, measured values of vibrational properties were very consistent, with all the 81 L specimens from the global untreated sampling following a clear and little dispersed trend between tanδL and E’L/γ, that is of the same form as the standard relations found in the literature ([38], [39], [4]). This relation for mulberry wood is shifted downwards, to significantly lower damping coefficient than would be expected for equivalent specific modulus, and this can be ascribed to its particular extractives content and composition ([44], [45]). But this was even more pronounced on the current material, with a tanδL that was on average 35% lower than one predicted by the standard trend.
Indicators of the effect of thermal treatment through time
Weight loss (WL) is classically used as indicator for the efficacy of thermal treatments. It is defined here as the relative oven-dry weight variation resulting from the treatment. The colorimetric measurements are also often used. In Fig. 3, the evolution of WL and of colour lightness (L*) as a function of TT is shown, both as a function of time as usual, and of the logarithm of time as a way to highlight the short-time processes. The global amplitude of WL due to this mild TT (150 °C and 0% RH) was comparable between mulberry and previous results on spruce ([21]), with a WL of approx. -4% at the end of the treatment. Untreated mulberry was obviously darker (by -20 points of L*) than spruce, but both species darkened similarly with a loss of about 30 points of L* at the end of TT. Schematically, WL expresses a degradation (loss of components) whereas colour changes express chemical transformations. Here, WL was moderate, while colour changes were important. The examination of these indicators in logarithmic scale of time (Fig. 3a, Fig. 3b) suggests that chemical transformations (approximated by L*) occurred from the starting of treatment, while degradation (approximated by WL) started to be significant (< -1%) from about 3 days of TT; but also, that the TT proceeds slightly faster on spruce than on mulberry.
Fig. 3 - Evolution of weight loss and of colour lightness as a function of thermal treatment duration, in logarithmic (a, b) or linear (c, d) time scale. Present results on mulberry (wide circles) are compared to a similar treatment on spruce (small triangles) from a previous study ([21]).
Beside colour lightness L*, the chromatic components a* (red-axis) and b* (yellow-axis) can provide further insight on the effect of natural or artificial ageing and of (hygro-) thermal treatments ([25], [26], [50], [51]). Fig. 4a and Fig. 4b show the evolution of a* and b* along TT duration, where trends for mulberry and spruce appeared quite different. However, much of these apparently different effects of TT on chromatic values actually come from the different values of the untreated wood of these two species, and from the peculiarities of the CIELab colorimetric space, where the “amount of colour” (a*, b*, and chromaticity C*) is intrinsically dependent on the position on the lightness L* axis ([27], [6]). When observing the evolution of a*, b*, C* and hue angle h° against the values of L* (Fig. 4c, Fig. 4d, Fig. 4e, Fig. 4f), the initial differences in untreated colour were clear, but, apart from different initial colour values, the trends of changes due to TT appeared in a very close continuity for mulberry and for spruce.
Fig. 4 - Evolution of chromatic colour parameters. Values on red-axis a* and yellow-axis b* plotted against duration of TT (a, b) or against concomitant evolution in colour lightness L* (c, d), and (e, f) evolution of chromaticity C* and hue angle (h°) along that in L*. Present results on mulberry (wide circles) are compared to a similar treatment on spruce (small triangles) from a previous study ([21]). Arrows indicate the changes in L* of samples with an increase in treatment duration.
Changes in physical properties after thermal treatment
Fig. 5 - Effects of mild thermal treatment on (a, c) specific gravity γ and on (b, d) EMC at 20 °C and 65% RH; (a, b) as a function of TT duration; (c, d) as a function of weight loss. Circles: mulberry; triangles: spruce (from [21]). The dashed symbols mean that data were averaged over R and L specimens.
Fig. 5 shows the changes in physical properties after thermal treatment. Results were here averaged over specimens in R and L directions, as γ and EMC are volumetric parameters, not directional. For EMC, the changes were expressed as absolute differences (EMCtreated - EMCuntreated), while for the other physical-mechanical properties, hereafter, the data shown in the graphs were the relative variations in properties according to the following equation (eqn. 6):
where ΔXf is the actual variation due to treatment, X’t and Xu are the treated value and untreated value for property X, respectively. X’t was corrected by subtracting the variations measured on the control groups, that accounted for the variations due to any physical and conditioning steps ([30], [37], [8], [8]) other than the effect of the applied treatments.
In Fig. 5a it can be deduced that increasing treatment duration decreased specific gravity, by as much as 4%, which was the result of changes in mass (Fig. 5c) and lesser so in dimensions. The reduction in γ was very close between mulberry and spruce. In Fig. 5b and Fig. 5d, the EMC decreased by -1.3 points of EMC for mulberry at the longest duration or highest weight loss. It can be expected that the dimensional changes due to changes in moisture content decrease while stability will improve ([16]). However this was a much more moderate difference than for spruce at a similar WL, where a decrease of -3.8 points of EMC is observed ([21]). Yet, as the untreated wood of both species showed very different initial EMC (9.3% for mulberry, 10.9% for spruce), their EMC after TT were relatively close (7.9% for mulberry, 7.3% for spruce).
Changes in vibrational properties after thermal treatment
In Fig. 6 variations in specific modulus of elasticity and damping during treatment are shown. In Fig. 6a, there was a clear decrease in E’/γ along TT duration, with a trend that seemed to match that of spruce for L direction. For mulberry the reduction in specific modulus was comparable in R and L directions, while for spruce there was an increase in R instead of the decrease observed in L. Concerning tanδ in Fig. 6b, there was first a decrease in damping for mulberry in both directions, and then it seemed to increase again with TT duration. In the results of Kubojima et al. ([23]) on spruce, it is proposed that an increase in crystallinity would predominate in the earlier stages of treatment, while the degradation of cell-wall polymers would gradually become predominant over longer durations of heating. However, extractives might suffer some degradation at an earlier stage than wood constitutive polymers. As extractives represent approx. 12% in mulberry heartwood and are responsible for its proportionally low EMC and damping ([44]), their possible early degradation might hide some more general effects due to cell wall polymers. The amplitude of variations in tanδ for mulberry was very moderate, with maximum values of only -6%, smaller than the apparent trend for spruce in L direction, and much smaller than for spruce in R direction, where tanδ was reduced by nearly 30% ([21]).
Fig. 6 - Effects of mild thermal treatment on (upper row: a, c, e) specific modulus of elasticity E’/γ and on (lower row: b, d, f) damping coefficient tanδ; (a, b) as a function of TT duration; (c, d) as a function of weight loss; (e, f) as a function of TT-induced differences in EMC. Circles: mulberry; triangles: spruce (from [21]); filled symbols: L direction; open symbols: R direction.
Fig. 6c and Fig. 6d expresses these trends in vibrational properties as a function of weight loss (WL), which was increased with TT duration and reached 4.1%. WL can be considered as an indicator of the intensity of TT ([14], [21]). An increase of WL (Fig. 3) can be the result of some degradation in wood polymers or in extractives. However, there may be competing effects of crystallization and of degradation, especially in the early stages of treatment ([23]). Furthermore, apparent WL is only a rough estimate of phenomena, as hemicelluloses could be significantly degraded into sugars, but that remain into the TT-treated wood, and can contribute to observed variations in both vibrational properties and hygroscopicity ([36], [50]).
Fig. 6e and Fig. 6f plots the relation between differences in EMC and variations of vibro-mechanical properties. In Fig. 6e, when EMC was decreasing, the specific modulus of elasticity was decreasing for both R and L directions in mulberry, with a much steeper slope than observed for L spruce, and with opposite effects on E’R/γ than observed for R spruce ([21]). In Fig. 6f, the slight decrease in tanδ for mulberry could appear in a similar order of magnitude of the tanδ-EMC trend as for spruce in L direction, but clearly not for R direction where spruce tanδ strongly decreased.
In the literature, it was first proposed that TT at short duration of treatment (at 0% or low RH) would increase E’/γ at mild temperatures (≤160 °C) and decrease it at higher temperatures, with stronger amplitudes in R than L directions ([23]). For damping, it was suggested that at the beginning TT would always increase tanδ in L direction and then decrease it ([23]). However, later studies have indicated (for TT at 120 °C and low RH) a slight increase in E’L/γ and slight decrease in tanδL, both mostly due to the effects of decrease in EMC ([14]); then (for TT at 140 °C and 0% RH, and once recovery effects taken into account) a slight decrease both in E’L/γ and in tanδL ([50], 2019b), along with a reduction in EMC.
For untreated wood, a reduction in EMC should bring an increase in E’/γ and a decrease in tanδ, and all the more so in R direction ([30], [8]). The applied TT (at 150 °C and 0% RH) appears to have an intrinsic effect of decreasing E’/γ, despite reduced EMC, while a significant part of the decrease in tanδ could be due to the reduction in EMC following TT. Because of reduction in moisture content of wood it can be deduced that the accessibility to hydroxyl groups has been reduced, which could be because of the degradation of hemicelluloses or crystallisation of celluloses ([23], [24]). The contrasted effects observed on spruce in R direction (increase in E’R/γ, strong decrease in tanδR) could be explained by its strong natural anisotropy and by the much higher sensitivity of R direction to EMC changes. The contrasted effects of TT on mulberry compared to spruce could be explained by the atypical properties of native mulberry: its very small degree of anisotropy was reflected in TT having similar effects in L and R directions, while its naturally low EMC and tanδ, due to its particular extractives, probably explain why TT was not bringing much additional reduction in its EMC and tanδ.
Effects of specimens’ histories
Fig. 7 and Fig. S2 (Supplementary material) show the relationship between the changes (relative to untreated wood) in EMC and in E’/γ and in tanδ. For each group (=duration of TT), the arrow indicates the shift from untreated data, to data after TT, and to data after reconditioning/recovery.
Fig. 7 - Compared effects of reconditioning on mulberry (for 2 longest TT durations, diamonds and squares) and on spruce (triangles, from [21]). Upper row (a, c) for specific modulus, lower row (b, d) for damping; left side (a, b) for L direction, right side (c, d) for R direction. Empty symbols correspond to values after thermal treatment; filled symbols correspond to values after reconditioning.
For mulberry there was, globally, an almost complete recovery of the initially observed reduction in EMC after TT. These findings agreed with previous studies on reconditioning that show recovery of temporary changes in EMC ([14], [35], [21]). According to Obataya ([35], [37]) and Endo et al. ([14]), the reversibility of EMC (and associated changes in physical-vibrational properties) results from the physical ageing of polymers in wood structure ([19]). Closure of micro pores can also cause such a temporary reduction in EMC ([14]). Besides, the chemically irreversible effects of TT (such as hydrolysis and crystallisation) cannot be considered as an explanation for EMC reversible change ([21]). In L direction, E’L/γ (Fig. 7a, Fig. S2 in Supplementary material) was now even slightly increased compared to untreated wood. A slight increase in E’L/γ is compatible with previous findings on effects of TT at mild temperatures and low RH; however, previous studies found that E’L/γ was re-decreased during recovery ([14], [35]), while in R direction, for the groups of longest TT duration and most significant WL, E’R/γ remained significantly reduced. For tanδ (Fig. S2b, Fig. S2d - Supplementary material), the effects of reconditioning were inconsistent for groups with the shorter TT durations (≤1 day) and smaller WL (≤1%), although there was a complete recovery in EMC and often some recovery also in tanδ. For the group treated for 3 days (WL of -1.8%), after the initial TT-induced decrease there was a complete recovery. For the longest and most efficient TT (9 days, WL -4.1%), tanδ was re-increased relative to untreated values.
In Fig. 7, the effect of reconditioning is compared between mulberry, for the two longest durations of TT (3 and 9 days), and spruce (7 days of TT). For spruce, TT-induced variations were only partially recovered (to 8% EMC instead of 11% for untreated wood). The reversible part of EMC changes was smaller than reported by Obataya et al. ([31]) or Endo et al. ([14]); however, the TT here applied was either at higher temperature or for longer duration. Following this recovery in EMC, E’/γ decreased again as compared to the initial TT-induced decrease. This tendency was logical but the decrease after recovery was stronger than could be expected for a re-gain in EMC of only +1 point. A possible explanation would be that TT induced some degradation of hemicelluloses into sugars, that would limit the moisture re-uptake ([36]), and act as plasticizers in the wood cell-wall reducing E’/γ. Results on mulberry were contrasted. The TT-induced reduction in EMC was more completely recovered than for spruce. E’/γ of mulberry was completely recovered in L direction, but remained decreased in R. The re-increase in E’/γ (notable in L, slight but visible in R), along a re-increase in EMC, is difficult to explain, as well as some increase in L E’/γ (shorter TT durations - Fig. S2 in Supplementary material) relative to untreated values. The oven-dry TT, but also possibly the initial oven-drying of untreated specimens, could have caused drying stresses decreasing E’/γ and recovered by high humidity conditioning ([34], [37]). In spruce, the much wider amplitude of changes in EMC could hide these effects. For mulberry, the remaining decrease in E’/γ in R direction, that is more affected by cell-wall matrix components than L, could express some kind of degradation in hemicelluloses as in spruce ([50]) or in particular extractives of mulberry, that strengthen its E’/γ in native wood ([44]). Actually, a transport of extractives from the core to the surface of specimens during TT has been reported ([9]).
For tanδ, the effect of recovery on spruce followed exactly the same tanδ-EMC path, in opposite direction, than the initial TT-induced variations, except that the recovery was not complete. For mulberry, a recovery following the same tanδ-EMC path as the TT effect was also observed for the group treated 3 days (that had the lowest TT-induced decrease in tanδ and only -1.8% WL). However, for the longest TT (9 days), that had a smaller TT-induced decrease in tanδ, damping was clearly increased after recovery above untreated values. It might be speculated that for this treatment with the highest WL, the extractives that were responsible for the low tanδ of native mulberry started to be degraded.
Conclusions
Out results indicated that: (i) Mulberry wood confirmed its highly atypical properties: low density for a hardwood, very low specific modulus of elasticity, very low anisotropy, and yet a lower-than-expected damping coefficient; (ii) equilibrium moisture content (EMC) reduced with TT duration, which can be attributed to the hemicellulose degradation and accordingly reduced accessibility to hydroxyl groups; (iii) specific gravity (γ) and specific modulus of elasticity (E’/γ) decreased with TT duration, while for damping (tanδ) there was a decrease in the beginning, but that became smaller at end of TT; (iv) the decreases in EMC might have been attributed to the degradation of hemicelluloses. However, after reconditioning most of the changes have been recovered; therefore, most part of TT effects would rather be due to physical changes in wood (or polymers internal configuration) during thermal treatment; (v) the extractives content of mulberry wood may be an explanation for its different reaction both to thermal treatment, and recovery; its very low anisotropy also induced differential effects compared to spruce; (vi) the thermal treatment, which has been used in this study for mulberry wood, showed a reversible effect after high humidity re-conditioning. An “improvement” in vibrational properties of wood has not been achieved, however aesthetic appeal of the treated mulberry wood specimens was considerable.
It is suggested to investigate different treatments conditions on Morus alba, and to study naturally aged Morus alba which is used as the material of musical instruments. More generally, it would be interesting to study thermal and hygrothermal treatments on various species with peculiar extractives and/or native properties, such as those used in instrument making, that would also help to better understand the various phenomena involved.
Acknowledgements
The authors acknowledge the help of Mr. Samad Zare, musical instrument maker from Iran, for providing the studied material of qualified mulberry wood, and to Dr. Pierre Cabrolier (wood scientist and craftsman from France) for fine machining of test specimens. Also, the authors are grateful for the supporting and funding of the thesis of EK by Campus France and Embassy of France in Iran. Part of this work has been supported by Iranian Center for International Research and Collaboration and French Embassy in Tehran in the frame of Gundishapur project “Mechanical effects of pre-treatment and finishing on wood panels of cultural heritage”. EK is grateful for its current Post-Doc position that is supported by the project “Advanced research supporting the forestry and wood-processing sector’s adaptation to global change and the 4th industrial revolution”, contract no. CZ.02.1.01/0.0/0.0/16_ 019/0000803 financed by OP RDE.
References
Gscholar
Online | Gscholar
Gscholar
Gscholar
Gscholar
Gscholar
Gscholar
Gscholar
Gscholar
Gscholar
Gscholar
Gscholar
Gscholar
CrossRef | Gscholar
Authors’ Info
Authors’ Affiliation
Iris Brémaud 0000-0001-7999-6661
Sandrine Bardet 0000-0003-2156-6014
Tancrède Almeras
Wood Team, LMGC, CNRS, Univ. Montpellier, Montpellier (France)
Department of Wood Processing and Biomaterials, University CULS, Prague (Czech Republic)
Patrick Langbour 0000-0001-6037-255X
BioWooEB, CIRAD, Montpellier (France)
Department of Wood and Paper Science and Technology, Faculty of Natural Resources, University of Tehran, Karaj (Iran)
Université Clermont Auvergne, CNRS, Institut Pascal, Clermont-Ferrand (France)
Corresponding author
Paper Info
Citation
Karami E, Brémaud I, Bardet S, Almeras T, Guibal D, Langbour P, Pourtahmasi K, Gril J (2022). Reversible and irreversible effects of mild thermal treatment on the properties of wood used for making musical instruments: comparing mulberry to spruce. iForest 15: 256-264. - doi: 10.3832/ifor4074-015
Academic Editor
Petar Antov
Paper history
Received: Feb 06, 2022
Accepted: May 16, 2022
First online: Jul 20, 2022
Publication Date: Aug 31, 2022
Publication Time: 2.17 months
Copyright Information
© SISEF - The Italian Society of Silviculture and Forest Ecology 2022
Open Access
This article is distributed under the terms of the Creative Commons Attribution-Non Commercial 4.0 International (https://creativecommons.org/licenses/by-nc/4.0/), which permits unrestricted use, distribution, and reproduction in any medium, provided you give appropriate credit to the original author(s) and the source, provide a link to the Creative Commons license, and indicate if changes were made.
Web Metrics
Breakdown by View Type
Article Usage
Total Article Views: 5434
(from publication date up to now)
Breakdown by View Type
HTML Page Views: 2240
Abstract Page Views: 1789
PDF Downloads: 1028
Citation/Reference Downloads: 2
XML Downloads: 375
Web Metrics
Days since publication: 1090
Overall contacts: 5434
Avg. contacts per week: 34.90
Article Citations
Article citations are based on data periodically collected from the Clarivate Web of Science web site
(last update: Mar 2025)
Total number of cites (since 2022): 1
Average cites per year: 0.25
Publication Metrics
by Dimensions ©
Articles citing this article
List of the papers citing this article based on CrossRef Cited-by.
Related Contents
iForest Similar Articles
Technical Notes
Improving impregnation properties of fir wood to acid copper chromate (ACC) with microwave pre-treatment
vol. 8, pp. 89-94 (online: 01 April 2014)
Research Articles
Kinetic analysis of poplar wood properties by thermal modification in conventional oven
vol. 11, pp. 131-139 (online: 07 February 2018)
Research Articles
Thermo-modified native black poplar (Populus nigra L.) wood as an insulation material
vol. 14, pp. 268-273 (online: 29 May 2021)
Research Articles
The physicomechanical and thermal properties of Algerian Aleppo pine (Pinus halepensis) wood as a component of sandwich panels
vol. 15, pp. 106-111 (online: 21 March 2022)
Short Communications
Influence of thermo-vacuum treatment on bending properties of poplar rotary-cut veneer
vol. 10, pp. 161-163 (online: 13 June 2016)
Research Articles
Improving dimensional stability of Populus cathayana wood by suberin monomers with heat treatment
vol. 14, pp. 313-319 (online: 01 July 2021)
Research Articles
Physical-mechanical properties and bonding quality of heat treated poplar (I-214 clone) and ceiba plywood
vol. 8, pp. 687-692 (online: 17 December 2014)
Technical Advances
Technical properties of beech wood from aged coppices in central Italy
vol. 8, pp. 82-88 (online: 04 June 2014)
Research Articles
Heat treatment of poplar plywood: modifications in physical, mechanical and durability properties
vol. 16, pp. 1-9 (online: 09 January 2023)
Research Articles
Dielectric properties of paraffin wax emulsion/copper azole compound system treated wood
vol. 12, pp. 199-206 (online: 10 April 2019)
iForest Database Search
Search By Author
Search By Keyword
Google Scholar Search
Citing Articles
Search By Author
Search By Keywords
PubMed Search
Search By Author
Search By Keyword