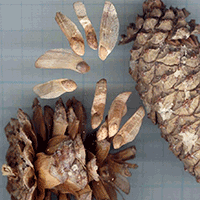
Seed germination traits of Pinus heldreichii in two Greek populations and implications for conservation
iForest - Biogeosciences and Forestry, Volume 15, Issue 4, Pages 331-338 (2022)
doi: https://doi.org/10.3832/ifor4045-015
Published: Aug 24, 2022 - Copyright © 2022 SISEF
Research Articles
Abstract
Seed germination traits were assessed on Pinus heldreichii H. Christ, a Tertiary relict, high-elevation Mediterranean pine, endemic in the western Balkan Peninsula and southern Italy; it is naturally grown at the northern Greece mountains, but also found in shrubby form above the timberline. Closed and mature cones were collected (October) for three consecutive years from Pindos Range and Mt. Olympus populations. Cone and seed morphological traits were recorded along with the seedling cotyledon number. Seed germination tests were performed under various ecologically meaningful temperatures and light regimes; climate change effects on seed germination and seedling development were assessed based on two climate scenarios. Final seed germination was moderate (~55%) on both, untreated seed lots at most favourable conditions (15 and 20 °C). Although cold stratification is not an absolute requirement, seed germination rate and final percentage are promoted by both a month of cold stratification (at least 30 or 45 days) and white light. The predicted climate change suggests that a prolonged drought period (>3 months) might turn out by the end of the century in the populations studied. Although the temperature increase might not have significant effects in the germination window of the species. The rainfall decrease will inevitably expose the young seedlings to the summer drought, thus increasing the potential mortality rate. Both ex situ (e.g., germplasm conservation) and in situ measures of conservation are recommended for the species survival in Greece, with a particular focus on the populations established at lower altitudes.
Keywords
Climate Change, Cold Stratification, Cotyledon Number, Heldreich’s Pine, Light Response, Seed Ecophysiology, Seed Mass
Introduction
Pinus heldreichii H. Christ. (1863) belongs to the high-elevation pines that grows in the treeline of the Mediterranean Basin ([11], [26]). Heldreich’s pine is a Tertiary relict species ([44]), a long-lived tree, exceeding 1400 years of age ([25]) and endemic to the mountains of the Balkan Peninsula ([10], [43], [18]). Its native, scattered populations are distributed from Bosnia and Herzegovina, Montenegro, Albania, Serbia, North Macedonia, and southwest Bulgaria to northern Greece (Fig. 1), while isolated populations occur in southern Italy ([7]). In Greece, P. heldreichii is naturally growing in the northern part of the country, at high elevations from 1300 to 2300-2600 m a.s.l., forming woodlands up to the treeline, in pure stands or mixed forests - often replacing P. nigra at higher altitude; it is also found in a shrubby form well above the timberline ([10], [16]). It covers a relatively small area (8300 ha) growing at the southern limits of the species world distribution ([36]) and its populations are distributed in Mount (Mt.) Olympus and the Pindos Range (Valia Kalda and Vikos-Aoos National Park, Mt. Smolikas and Zagori area), on serpentine, limestone or granite soils ([16]).
Fig. 1 - The distribution of Pinus heldreichii in the Mediterranean Basin, adapted from the European Forest Genetic Resources Programme ([43]).
P. heldreichii is a tolerant tree to wind, ice and heavy snowstorms and therefore, in certain cases, has been used on high-altitude reforestation ([43]). Due to its very thick bark (ash-grey in young trees, yellowish-brown, splitting into rhomboid patches at maturity), twigs and shoots, in addition to the unique needle arrangement, the species is considered as a pine with a high ornamental value ([44]).
Although the species has a rather limited distribution, its habitats are considered important, as they constitute climax plant communities of great ecological value ([16]). The habitat of the species is considered as Near Threatened (NT) in the wider range of the EU28 (European Red List of Terrestrial and Freshwater habitats - [23]). The species has been assessed as Least Concern (LC) at global and European level ([32]), forming fragmented populations found on edaphically limited sites and presenting a continuing decline in area, extent and quality of its habitat (IUCN Red List of Threatened Species - [8]). Human influence on the climate system is currently well documented and the continued emission of greenhouse gases will cause further warming and long-lasting changes in all climatic components, increasing the likelihood of severe, pervasive and irreversible impacts for ecosystems ([21]). Tree- and timber-lines have received growing interest in recent decades, due to their potential role as biological indicators of climate ([15], [3], [6], [45]) and land use change ([19]). Climate warming may cause shifts in the latitudinal and altitudinal distribution of species, increase extinction rates, and alter provision of ecosystem services; therefore, accurate predictions of the future potential distributions of key species, such as endemic forest trees ([9]) are greatly needed. A temperature increase in timberline zones can have various effects, including treeline shifts ([27], [34]), enhanced tree height growth and higher reproductive success ([28]). However, limited research has been conducted regarding climate change effects on seed germination and seedling growth but an overall negative impact of global warming on seed germination is anticipated ([42], [46], [12]).
Among the most important and probably devastating threats for P. heldreichii are (i) the potential hybridization with (planted) Pinus nigra J.F. Arnold ([8]); (ii) habitat fragmentation and reduced gene flow ([43]); (iii) intensive exploitation, road construction and tourism development; (iv) illegal cutting and grazing; and (v) wildfires and global climate change effects. A notable consequence of climatic change could be the increased wildland fire risk in high altitudes and for species not adapted to fire, e.g., Abies cephalonica ([14]). Specific ex situ conservation actions can significantly contribute to the protection of these species.
Heldreich’s pine is a monoecious tree, up to ca 35 m tall ([44], [10]), and seed production begins at the age of 15-20 years ([43]). “Flowering” occurs in spring, from May to June (or July). Female cones develop in a whorl, usually 1-2 (rarely in clusters of 3), that ripen in early autumn of the second year after pollination, from September (occasionally August) to October ([44], [36], [26]). Seeds are grey coloured, 5-7 mm long, with a 25-30 mm long wing. Seed dispersal occurs in autumn (September-October); intervals between large cone crops (masting years) have not been documented ([26]). According to the recommendations for breaking seed dormancy of the International Rules for Seed Testing ([22]), P. heldreichii is a species with an absolute pre-chilling requirement (42 days of cold stratification at 3-5 °C).
It is well known that reproduction, seed dispersal, germination and seedling establishment constitute the most critical stages in a plant life cycle. Germination characteristics of seeds collected from different sites can vary in many ways ([2]), and especially for P. heldreichii, in the degree of dormancy ([5]).
The aim of the present study is the investigation of P. heldreichii seed germination ecophysiology, with the use of laboratory tests performed under various ecologically meaningful temperatures, chilling treatments and light regimes. In addition, climate change effects on seed germination and seedling development are also assessed based on two climate scenarios. A three-year study of seed germinability was carried out on seeds collected from the two main P. heldreichii populations of Greece. Since relatively little research has been carried out on Heldreich’s pine seed germination ([4], [5]), data obtained in this study could contribute to a better understanding of germination mechanisms, seedling establishment and natural regeneration trends of P. heldreichii ([17]) concerning its scattered natural populations. Furthermore, the results of this study might be used as recommendations to both forest ecologists and managers towards performing successful germination tests and massive seedling production in nurseries, thus ensuring an effective conservation policy.
Materials and methods
Cone collections, biometric characteristics and seed processing
Closed, mature cones of P. heldreichii (n = 20-32) were gathered from ~25 randomly selected trees per collection during the maturation period (October) from two natural populations in the Pindos Range (Metsovo, Katara - 39° 48′ 5.91″ N, 21° 15′ 11.09″ E; elevation 1400 m a.s.l.) and Mt. Olympus (Katerini, Agapitos Refuge - 40° 04′ 38.35″ N, 22° 22′ 18.11″ E; elevation 2200 m a.s.l.). Cone collections were carried out by the local Forestry Departments of: (i) Metsovo in 2011, 2012 and 2013 (hereafter batches A, B and C, respectively), and (ii) Katerini in 2012 and 2013 (hereafter batches D and E, respectively). Closed cones (Fig. 2a) were left to air-dry in a sunny place at room conditions (~20 °C) until they opened (after 1-2 weeks) without any additional treatment, as recommended by Krugman & Jenkinson ([26]). Fresh cone mass (g) and mass of sound seeds per cone (g) were weighed in the laboratory (Tab. 1) with an analytical balance (N34120® Navigator portable electronic balance, OHAUS, Switzerland - accuracy 0.1 mg). Seeds were extracted from the cones manually (Fig. 2b) and their wings were removed. For each cone, the total number of seeds was counted (20-130 min-max values) and the total mass of the seeds from each cone was measured; the average individual seed mass (mg) was calculated based on the total seed mass that was divided by the number of seeds per cone. Seeds of the same collection year and provenance were merged into a single batch (a total of 5 batches, A-E).
Fig. 2 - (a) Mature, open and closed Pinus heldreichii cones bearing long-winged seeds; (b) processed seeds prepared for germination tests; (c) young seedlings.
Tab. 1 - Cone mass and seed characteristics of Pinus heldreichii according to Greek provenances and date of collection.
Provenance | Altitude (m a.s.l.) |
Date of collection |
Cone mass (g) (min-max) |
n | Seeds per cone (min-max) |
Seed mass (mg) (min-max) |
Seed/cone mass (%) (min-max) |
---|---|---|---|---|---|---|---|
Pindos Range (Metsovo) |
1400 | 21/10/2011 | 15.89 ± 0.72 | 20 | 61.05 ± 3.33 | 31.13 ± 1.34 | 12.10 ± 0.75 |
(9.65-24.35) | (36-84) | (17.13-39.11) | (6.39-17.52) | ||||
11/10/2012 | 11.80 ± 0.56 | 30 | 60.07 ± 3.05 | 23.72 ± 1.63 | 11.59 ± 0.57 | ||
(7.50-16.90) | (20-85) | (8.16-50.00) | (4.94-18.00) | ||||
Mt Olympus (Agapitos Refuge) |
2200 | 15/10/2012 | 15.59 ± 0.63 | 32 | 78.66 ± 3.89 | 22.62 ± 0.85 | 11.37 ± 0.53 |
(10.10-24.00) | (24-130) | (12.35-32.39) | (4.74-16.59) |
Seed germination experiments
Germination tests for each treatment were performed with 5 samples of 20 seeds per Petri dish (diameter 9 cm, lined with two discs of filter paper and moistened with 6 ml of deionised water). Seed germination experiments were performed in temperature-controlled plant growth chambers (model KBW 240, Binder, Tuttlingen, Germany), where temperature was kept constant within ± 0.5 °C, in either alternating light/dark conditions (L/D, photoperiod 12:12 h) or continuous darkness (D). For the experiments in continuous darkness, Petri dishes were placed in metal, light-proof containers.
Germination experiments were carried out at: (i) constant (10, 15, 20, 25 and 30 °C) and alternating temperatures (20/10 °C) for untreated (non stratified) seeds; and (ii) at constant temperatures (10, 15 and 20 °C) after various chilling durations (0, 15, 30 and 45 days) for treated (stratified) seeds. Furthermore, in the case of batch A, seeds were transferred under L/D, D and Far Red light (FR/D) at 20 °C, after 30 and 45 days of cold stratification. Daily Far Red light in the plant growth chamber was obtained by filtering white light through 2 blue + 1 red plexiglass filters during the day, thus simulating the light conditions for seeds lying on the soil surface under a closed forest canopy ([40]). Cold, moist stratification was carried out by keeping the imbibed seeds in darkness, at a temperature of 2-4 °C (in a refrigerator). A number of the treatments were not applied in certain batches (indicated with “×” in Figs. 4 and 5) as a result of limited seed availability.
As a follow-up experiment, after completing the constant temperature tests without stratification, the non-germinated seeds were further transferred, to alternating or constant temperatures under L/D (12:12 h) as follows: (i) from 10 °C (L/D and D), 15 °C (L/D) and 30 °C (D) to 20/10 °C L/D for batch A; and (ii) from 10 °C L/D to 20 °C L/D for batches B-E.
Germination was recorded every 2-3 days in a darkroom, under green safelight and germinated seeds were discarded after each count. The criterion of germination was visible radicle protrusion. Germination experiments were completed when no additional seeds germinated for 2 weeks ([36]). When germination tests were completed, cut tests were performed on the non-germinated seeds and final germination percentages were corrected for sound seeds. The germination rate (T50) of untreated seeds, expressed as time to 50% of final germination, was calculated following Skourti & Thanos ([37]).
Cotyledons
Following each germination test, germinants (Fig. 2c) were developed in a plant growth chamber for a short period (2-3 weeks at 15 °C) and then transplanted outdoors in the nursery, in planting trays (1 part humus : 1 part peat : 1 part perlite). The number of cotyledons per individual was counted in a total of 555 seedlings (81, 85, 36, 125, and 228 seedlings from batches A to E, respectively).
Present and future climatic conditions
Climatic data were extracted from the WorldClim datasets ([48]), in order to investigate the possible effects of climate change on P. heldreichii natural populations. For the present period, the climate data for 1984-2014 were used to construct the Walter & Lieth ([47]) ombrothermic diagrams for both study areas, produced using the “climatol” R package with minor changes on its base code. For the future climatic conditions, the CNRM-ESM2-1 earth data model ([35]) for the period 2081-2100, was used along with two shared socio-economic pathways (SSP245 and SSP585 - [31]). For both cases the spatial resolution used was 2.5 min (~21 km2).
Statistical analysis
Germination percentages were arcsine transformed prior to analysis. Differences among light conditions in various temperatures in untreated seeds and durations of chilling pretreatment for each seed batch were tested by one-way analysis of variance (ANOVA), followed by either Tukey’s multiple comparisons test, or the non-parametric Kruskal-Wallis test, depending on whether the ANOVA assumptions were met (normal distribution and homogeneity of variance). All analyses were performed using the software package SPSS Statistics® v. 23 (IBM, Armonk, NY, USA).
Pearson’s Chi-squared test of goodness of fit for normality was performed for the number of cotyledons (confidence level 95%), using R v. 3.6.3 Programming Language ([30]). Throughout the study, the ± values refer to the standard errors of the mean (SE).
Furthermore, a model of Quasi-Poisson family and log link function were fitted to predict germination (g) with chilling (C), temperature (T), light (L), transfer (t) and location (S) (formula: g = C+T+L+t+S). The model’s intercept is at -3.81 (t = -16.75, p < 0.001).
Results
The average mass of freshly collected cones varied among batches from 11.8 ± 0.6 to 15.9 ± 0.7 g, with minimum and maximum values of individual cones at 7.5 and 24.3 g, respectively. The mean number of seeds per cone ranged between 60.1 and 78.7 seeds, depending on the collection year and provenance. Seed mass (average per cone) ranged from 22.6 ± 0.9 to 31.1 ± 1.3 mg (Tab. 1) with an overall mean 26.7 ± 0.8 mg. The average contribution (%) of seeds to the total cone mass varied among the 5 batches from 11.4% to 12.1%, with marginal values (of individual cones) at 4.7% and 18.0% (min and max). The mean number of cotyledons per seedling was 7.25 ± 0.04 (n = 555), the absolute values ranged from 5 to 9 and, although non-continuous variables, its frequency approximates the normal distribution (p-value = 0.8921). The overall mean percentage (%) of empty seeds, estimated from post-germination cut-tests, was 10.2%, 24.4% and 51.6% for Pindos Range batches A, B and C, respectively. Similarly, for Mt. Olympus, the relevant percentages were 12.1% and 19.9% for batches D and E, respectively.
According to the presented climate change scenarios, the under-study populations may face increased temperatures and prolonged periods of drought (Fig. 3). Seed germination of untreated seeds (Fig. 4) was promoted by white light, with best results obtained at constant temperatures, 15 or 20 °C (12:12 h L/D) with some exceptions (e.g., in batch C). However, final germination of untreated seeds under light was low or moderate (<55%) for all batches and treatments at 15 or 20 °C; at lower or higher temperatures (10 and 30 °C) no germination was recorded. Final germination of cold stratified seeds under 15 and 20 °C, but not under 10 °C, was significantly improved both in L/D and D (Fig. 5), whereas a 45-day chilling pretreatment (in some cases even a 30-day-long period) was sufficient for full germination under light/dark conditions at 15 and 20 °C.
Fig. 3 - Ombrothermic diagrams (according to [47]) for the locations of Agapitos Refuge (Mt. Olympus - upper panels) and Katara, Metsovo (Pindos Range - lower panels). Data were extracted from the WorldClim database for the years 1984-2014 (leftmost panels) and 2081-2100, based on the model CNRM-ESM2-1 and for the shared socioeconomic pathways 245 (central panels) and 585 (rightmost panels).
Fig. 4 - Final germination in untreated (non-stratified) Pinus heldreichii seeds, tested over a range of constant (10, 15, 20, 25 and 30 °C) and alternating temperatures (20/10°C), in the light (12 h photoperiod, white bars) or in continuous darkness (black bars). Different small-case letter labels indicate significantly different values between light and dark treatments within each temperature (p < 0.05). Results from Pindos Range collections are shown in graphs A (collection year 2011), B (2012) and C (2013), while Mt. Olympus collections appear in graphs D (2012) and E (2013). Non germinated (untreated) seeds of the non-stratified treatment were subsequently transferred as follows: (i) from 10 (L/D and D), 15 (L/D) and 30 °C (D) to 20/10 °C L/D for batch A; and (ii) from 10 °C L/D to 20 °C L/D for batches B-E (results are presented by grey bars). The “×” mark indicates lack of experimental data in the respective germination conditions.
Fig. 5 - Effect of various chilling durations (0, 15, 30 or 45 days) on final germination of Pinus heldreichii seeds at 10, 15 and 20 °C, under white light (12 h photoperiod, white bars), dark (black bars) and Far Red light (spotted bars). Different small-case letter labels indicate statistically significant differences among germination values obtained by different chilling durations within each temperature (p < 0.05). Results from Pindos Range collections are in graphs A (2011), B (2012) and C (2013), while collections from Mt. Olympus appear in graphs D (2012) and E (2013). The “×” mark indicates lack of experimental data in the respective germination conditions.
The effect of (i) chilling period (t = 17.65, p < 0.001), (ii) temperature (t = 4.02, p < 0.001), (iii) light (t = 10.22, p < 0.001), (iv) transfer to more favorable temperatures and light (t = 6.54, p < 0.001), and (v) provenance (t = 7.08, p < 0.001) were all statistically significant and positive, as shown by the GLM model (Tab. 2).
Tab. 2 - Generalized linear regression model (GLM) predicting the influence of variables on Pinus heldreichii seed germination.
Predictors | Incidence Rate Ratios |
Confidence Intervals (CI) |
p-value |
---|---|---|---|
(Intercept) | 0.02 | 0.01 - 0.03 | <0.001 |
chilling | 1.03 | 1.01 - 1.04 | <0.001 |
temperature | 1.03 | 1.02 - 1.05 | <0.001 |
light | 1.44 | 1.34 - 1.54 | <0.001 |
transfer | 1.34 | 1.23 - 1.46 | <0.001 |
provenance | 1.49 | 1.33 - 1.66 | <0.001 |
R2Nagelkerke | 0.446 |
All non-germinated seeds from the lowest, constant temperature (10 °C) experiments, both experiencing alternating light/dark conditions and continuous darkness, did not germinate after 5-6 weeks at 10 °C; after being transferred to a higher temperature (20 or 20/10 °C) in light/dark (grey bars in Fig. 4), they germinated to a considerable degree (41-89%).
The germination rate of untreated seeds was rather low; T50 ranged between 9 and 14 days in light germination experiments and 13 to 22 days under continuous darkness. A remarkable reduction of germination rate was achieved following stratification (T50 is lower than one week in seeds prechilled for 45 days). The time courses of P. heldreichii seed germination, for both untreated and stratified seeds, at 15 °C, in L/D and D presented in Fig. 6a and Fig. 6b, respectively, show a clear and considerable enhancement of germinability by light and cold stratification.
Fig. 6 - Time course of Pinus heldreichii seed germination at 15 °C, (a) in alternating light/dark conditions (12 h photoperiod), and (b) in continuous darkness, following cold stratification (at 2-4 °C in darkness) for 0, 15, 30 or 45 days. Seeds were collected from the Pindos Range provenance in 2012.
Discussion
Natural regeneration of Pinus heldreichii depends exclusively on seeds, therefore seed germination data, concerning temperature dependence as well as light and/or stratification requirements, are of paramount importance for the biology and conservation of the species, especially when climate change is taken into consideration.
On the basis of both morphological and molecular evidence ([24]), P. heldreichii belongs to the subsection Pinaster, section Pinus, subgenus Pinus (diploxylon or hard pines), together with the typical, low-altitude Mediterranean pines P. halepensis, P. brutia, P. pinaster and P. pinea and the closely interrelated pair of P. canariensis and P. roxburghii (Canary Islands and Himalayas, respectively). Average seed mass values obtained in this study for P. heldreichii (22.6 up to 31.1 mg), were between those reported for low-altitude Mediterranean pines P. halepensis (20 mg) and P. brutia (51 mg), although they might vary among collections and years and might be related to seed germination and seedling performance ([41], [13]). The cotyledon number per seedling, an indirect measure of both seed mass and initial photosynthetic capacity, significantly affects seedling survival ([13]). Mean cotyledon number in P. heldreichii seedlings (7.25) was quite similar with those reported for P. halepensis (7.4 - [13]), P. brutia (8.4 - [41]) and P. canariensis (8.2), with higher values obtained in heavier P. pinea (11.1) and P. roxburghii (11.2) seeds ([33]).
Masting has not been solidly proven for P. heldreichii, although there is some circumstantial evidence such as the irregularity of seed production between years ([38]) as well as variable germination percentages ([39]). High proportions (about 50%) of empty seeds in the seed batches of the Pindos Range provenance (C) shown in the present study is an additional indication of masting occurrence.
Without any pretreatment, seeds of P. heldreichii from both populations are observed to germinate to low or moderate levels (<55%), at 15-25 °C under alternating light/dark conditions. The inability of germination in darkness, mentioned previously for Greek populations ([36]), seems to be significantly reduced by white light at all temperatures tested. At low and high temperatures (10 and 30 °C), germination was fully suppressed in the dark; however, upon transfer of the non germinated seeds from darkness to light, significant levels of germination were recorded. It must be pointed out that higher germination percentages were also achieved upon transfer of non-germinated seeds from low to higher temperatures, i.e., from 10 to 20 oC, indicating a “chilling” pretreatment requirement ([4]).
In addition to white light, germination of P. heldreichii seeds was promoted by cold stratification as well (reported also by [5]). Cold stratification treatments have been traditionally used to break physiological dormancy (PD) in tree seeds, while if untreated, these seeds require more than 4 weeks of incubation prior to the initiation of germination ([2]). In accordance with older studies ([5], [20], [2]), a long period (70 days) of cold stratification is required to break PD in P. heldreichii seeds. Our results suggest that cold stratification is not an absolute requirement for seed germination, although a chilling period of 30-45 days enhances both final percentage and rate of germination, in agreement with the International Rules for Seed Testing ([22]). Therefore, according to an ecophysiological interpretation of our germination experiments, under the current climatic conditions, P. heldreichii seeds, following their autumn dispersal from cones, are subjected to cold stratification (under winter conditions) that will lead to a late spring-early summer germination, when temperatures are favourable (>15 °C) for seedling establishment, growth and survival. These results are in accordance to the earlier findings for the Italian Heldreich’s pine populations ([4], [5]).
According to the various climate change scenarios, the Mediterranean region will be particularly vulnerable to an anticipated temperature increase and precipitation decrease, likely resulting in amplified drought episodes and extreme temperatures, with cold resistant conifer habitats of high mountains being quite sensitive ([49]).
Taking into account the germinability of a proportion of seeds without prior chilling stratification, we may expect that the rising temperature, according to the two SSPs scenarios, may not affect significantly the seed germination and the subsequent initiation of seedling establishment. According to the climatic predictions, a high possibility of an extensive period of drought during early summer to late autumn (3-4 months) is obvious, especially in the case of the Pindos Range (Metsovo), as presented in the ombrothermic graphs for both the study areas. The probable increased drought season may have a significant effect on P. heldreichii seedling recruitment by increasing the mortality rate. The predicted change of both temperature and rainfall appear more extreme in the case of the Pindos Range. In the case of Mt. Olympus, where the population is growing at a higher elevation, we expect (at least for the milder SSP scenario) no significant drought period in the summer. Based on the above assumptions, the population in Pindos Range will face harsher climatic conditions, which may jeopardize its survival in the next century.
Finally, P. heldreichii, a thin-barked and non-serotinous pine, is not adapted to fire ([29]); the species does not survive even after swift surface fires, and shows a very limited post-fire regeneration, however it may invade burned areas from neighbouring unburned clusters. So far, no specific protocol for post-fire management has been proposed ([1]).
Conclusion
In the face of climate change, with predicted higher temperatures, prolonged drought periods and increased wildfire risk, P. heldreichii populations and habitats in Greece may be threatened, rendering particularly vulnerable populations established at lower altitudes; ex situ (e.g., germplasm conservation) and in situ measures (e.g., selection for drought tolerance) are recommended for the future conservation of the species.
Acknowledgements
Authors gratefully acknowledge the collaboration of the Forestry Directorates of Metsovo (Ioannina Prefecture, Epirus Region) and Katerini (Pieria Prefecture, Central Macedonia Region, Greece) in cone collections, Dr Muhammad Saeed for his assistance and Mrs Danae Panayiotopoulou, librarian at the Institute of Mediterranean & Forest Ecosystems, for the additional proof reading service. We thank the anonymous reviewers whose constructive comments and suggestions greatly helped to clarify and improve this manuscript.
Author contributions
Conceived and designed the experiments: END and CAT; performed the experiments: END; drafting of the manuscript and analyzed the data: END, KK and SO; contributing to materials/analysis tools: KK and SO; critical revision of the manuscript: CAT.
The authors have declared that no competing interests exist.
References
CrossRef | Gscholar
CrossRef | Gscholar
Gscholar
Gscholar
Gscholar
CrossRef | Gscholar
Gscholar
Gscholar
Online | Gscholar
Gscholar
CrossRef | Gscholar
Online | Gscholar
Gscholar
CrossRef | Gscholar
CrossRef | Gscholar
Gscholar
Gscholar
Gscholar
Gscholar
Gscholar
Gscholar
Gscholar
Authors’ Info
Authors’ Affiliation
Spyridon Oikonomidis 0000-0003-4779-6897
Institute of Mediterranean and Forest Ecosystems, Hellenic Agricultural Organization “DEMETER”, Terma Alkmanos str., 11528 Athens (Greece)
Spyridon Oikonomidis 0000-0003-4779-6897
Costas A Thanos 0000-0001-8748-8093
Department of Botany, Faculty of Biology, National and Kapodistrian University of Athens, Panepistimiopolis, 15784 Athens (Greece)
Green Fund, Ministry of Environment and Energy, Villa Kazouli, Kifisias 241, 14561 Kifisia (Greece)
Corresponding author
Paper Info
Citation
Daskalakou EN, Koutsovoulou K, Oikonomidis S, Thanos CA (2022). Seed germination traits of Pinus heldreichii in two Greek populations and implications for conservation. iForest 15: 331-338. - doi: 10.3832/ifor4045-015
Academic Editor
Gianluca Piovesan
Paper history
Received: Dec 23, 2021
Accepted: Jun 20, 2022
First online: Aug 24, 2022
Publication Date: Aug 31, 2022
Publication Time: 2.17 months
Copyright Information
© SISEF - The Italian Society of Silviculture and Forest Ecology 2022
Open Access
This article is distributed under the terms of the Creative Commons Attribution-Non Commercial 4.0 International (https://creativecommons.org/licenses/by-nc/4.0/), which permits unrestricted use, distribution, and reproduction in any medium, provided you give appropriate credit to the original author(s) and the source, provide a link to the Creative Commons license, and indicate if changes were made.
Web Metrics
Breakdown by View Type
Article Usage
Total Article Views: 23289
(from publication date up to now)
Breakdown by View Type
HTML Page Views: 20622
Abstract Page Views: 1619
PDF Downloads: 827
Citation/Reference Downloads: 3
XML Downloads: 218
Web Metrics
Days since publication: 820
Overall contacts: 23289
Avg. contacts per week: 198.81
Article Citations
Article citations are based on data periodically collected from the Clarivate Web of Science web site
(last update: Feb 2023)
(No citations were found up to date. Please come back later)
Publication Metrics
by Dimensions ©
Articles citing this article
List of the papers citing this article based on CrossRef Cited-by.
Related Contents
iForest Similar Articles
Research Articles
Delineation of seed collection zones based on environmental and genetic characteristics for Quercus suber L. in Sardinia, Italy
vol. 11, pp. 651-659 (online: 04 October 2018)
Review Papers
Genetic diversity and forest reproductive material - from seed source selection to planting
vol. 9, pp. 801-812 (online: 13 June 2016)
Research Articles
The effect of seed size on seed fate in a subtropical forest, southwest of China
vol. 9, pp. 652-657 (online: 04 April 2016)
Research Articles
Seed trait and rodent species determine seed dispersal and predation: evidences from semi-natural enclosures
vol. 8, pp. 207-213 (online: 28 August 2014)
Research Articles
Pollen contamination and mating patterns in a Prosopis alba clonal orchard: impact on seed orchards establishment
vol. 12, pp. 330-337 (online: 14 June 2019)
Research Articles
The effectiveness of short-term microwave irradiation on the process of seed extraction from Scots pine cones (Pinus sylvestris L.)
vol. 13, pp. 73-79 (online: 13 February 2020)
Technical Reports
Conservation and use of elm genetic resources in France: results and perspectives
vol. 13, pp. 41-47 (online: 03 February 2020)
Research Articles
Seedling emergence capacity and morphological traits are under strong genetic control in the resin tree Pinus oocarpa
vol. 17, pp. 245-251 (online: 16 August 2024)
Research Articles
Optimum light transmittance for seed germination and early seedling recruitment of Pinus koraiensis: implications for natural regeneration
vol. 8, pp. 853-859 (online: 22 May 2015)
Review Papers
Methods of soil seed bank estimation: a literature review proposing further work in Africa
vol. 15, pp. 121-127 (online: 26 March 2022)
iForest Database Search
Search By Author
Search By Keyword
Google Scholar Search
Citing Articles
Search By Author
Search By Keywords
PubMed Search
Search By Author
Search By Keyword