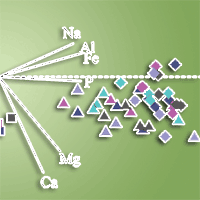
Mid-rotation fertilization and liming of Pinus taeda: growth, litter, fine root mass, and elemental composition
iForest - Biogeosciences and Forestry, Volume 14, Issue 2, Pages 195-202 (2021)
doi: https://doi.org/10.3832/ifor3626-014
Published: Apr 24, 2021 - Copyright © 2021 SISEF
Research Articles
Abstract
Forest floor litter can influence biogeochemical cycling and root growth in Pinus taeda systems, especially on low soil fertility sites. The impact of fertilization and liming on forest floor litter (quantity, elemental composition and root presence) was evaluated in a Pinus taeda stand in southern Brazil. A nutrient omission experiment was initiated in November 2008 on an 11 year-old Pinus taeda plantation. The experiment was a randomized block design with seven treatments and four blocks. The treatments were: complete (macro + micro + lime); minus macronutrients; minus micronutrients; minus K; minus Zn; minus lime; and control. In 2012, forest floor litter samples were collected, divided by layer (new litter, old litter, coarse fragmented forest layer > 2mm, fine fragment forest floor < 2mm, and fine roots) and analyzed for concentrations of Na, Al, and total nutrients. Results indicated that lime increased Ca and Mg concentrations, reduced Al toxicity, and improved fine root growth. An increase in fine roots was observed in treatments without K. There were large increases in Fe and Al as a function of litter age and increased Mn in fragmented litter when lime was applied. There was little variation in forest floor litter accumulation in all treatments. Elemental abundance was C>N>Fe>P>Ca>K>Mg>Mn under control conditions and C>N>Ca>Mg>P>Fe>Mn>K for the complete treatment. Occurrence of needle chlorosis, similar to that reported for Mg, and low growth under lime omission indicate that Mg was a major factor limiting growth. Fertilization and liming affected the bio-cycling of nutrients, Al toxicity, and root growth.
Keywords
Nutrient Concentration, Litter, Ca:Al Ratio, Forest Management
Introduction
Brazilian pine plantations have often been established on land not well-suited for agriculture crops due to very low soil fertility ([7]). Similar to others regions, nutrient removal by forest harvests can lead to soil nutrient exhaustion ([16]). Thus, replacing these exported nutrients is essential for maintaining long-term sustainability and yield ([29]). Replenishing pine plantation nutrients via fertilization at mid-rotation has proven more beneficial than initial fertilization ([11]).
The forest floor is relevant to soil protection and nutrients cycling. This distinctive feature of forest ecosystems is composed of litter (e.g., leaf, branches, bark, etc.) that are in different stages of decomposition above the soil surface. Efforts to preserve or maintain this forest component are critical for ensuring sustainability of forest systems ([29]). Soil fertility can play an important role on forest floor litter mass in pine systems since an inverse relationship between forest floor litter and soil fertility has been reported ([50]). This relationship might be associated with low residue decay due to low soil microbiological activity and poor nutrient quality of litterfall ([8]).
Residue nutrient quality, in terms of total available nutrients and C:N ratio, has been shown to be an important parameter in predicting residue decay ([53]). Nutrients such as Mn have been shown to affect decay ([9]). In both cases, total nutrients and Mn can be influenced by fertilizer and lime applications. Pinus spp. is known to generate poor residue with low nutrients and low decomposition rates that result in thick forest floors ([54]). Sanchez ([42]) confirmed that fertilization can accelerate decomposition and can also increase litterfall input.
Enhanced microbial activity due to fertilizer and lime amendment was noted as being responsible for a 70 % reduction in forest floor mass 20 years after application ([18]). Reductions in forest floor litter have been reported under different conditions by others ([24], [36]). In contrast, Kiser et al. ([20]) reported that fertilization had no influence on forest floor decomposition. Other studies have reported no change ([35]) or increased forest floor mass from fertilization ([34]). Contrasting effects of liming on forest floor mass were also observed with soil type ([38]) and application rate ([30]). Overall, these observations suggest that soil type and amendment rate may be important factors for maintaining forest floor litter and forest sustainability.
Nutrient amendments can be absorbed by roots within forest floor litter and soil ([22]); these nutrients enter into the forest biocycle ([7]) and affect litterfall composition. The influence of fertilization on forest floor composition varies among nutrients, since the rate of nutrient absorption and redistribution (from senescent needles to new tissue) varies from high for K, P, Cu and B, to very low for Ca and Al ([49]).
On the other hand, nutrient amendment can directly change the chemical composition of the forest floor when scattered over the litter ([7]). Applied nutrients can be physically bound in forest floor litter and immobilized or adsorbed by organic compounds resulting from different degrees of litter decomposition ([24], [7]).
The continual process of litterfall deposition and decay creates layers in the forest floor differing in age and chemical composition ([29]). Nutrient concentrations in forest floor layers can have either direct or inverse relationships with residue age and degree of decomposition ([47]). Leaching and root absorption can result in decreased concentrations while mass loss from decomposition and/or input from tree canopy litterfall can have an inverse effect. In addition, Fe and Al are major soil constituents that can strongly affect litter composition by direct soil contact and deposition via soil bioturbation ([24], [39], [37]).
The influence of fertilization and liming on forest floor roots has been evaluated under different conditions and in different plant species ([43]). Fertilization can reduce roots found in forest floor litter ([6]). This response can vary by applied nutrients, and K application has been reported to decrease the amount of roots ([51], [44]). The influence of fertilization and liming on root responses in forest floor litter appears to be complex and few studies have evaluated this aspect under the conditions found in southern Brazil.
The objective of this study was to evaluate the influence of lime and fertilizer application on tree growth, quantity and quality of forest floor litter, as well as impacts on fine root production in an 11-year-old Pinus taeda stand in southern Brazil, where the standard length of the rotation is 22 years.
Materials and methods
Study site
The experimental area was located in the Jaguariaíva county (24° 18′ 58.66″ S, 49° 44′ 15.58″ W), Paraná state, southern Brazil. The regional climate is a transition between Cfa and Cfb ([2]) with average rainfall varying from 1400 to 1600 mm yr-1 and an altitude of 1070 m a.s.l. The soil was classified as a Red-yellow Oxisol and physical and chemical characteristics are shown in Tab. 1.
Tab. 1 - Soil physical and chemical properties of experimental area before fertilizer and lime application in Paraná state, southern Brazil. (ECEC): Effective cation exchange capacity; (m): Aluminum saturation.
Variable | Units | Soil layer (cm) | ||||
---|---|---|---|---|---|---|
0-5 | 5-10 | 10-20 | 20-40 | 40-60 | ||
pH CaCl2 | - | 3.2 | 3.6 | 3.7 | 3.8 | 3.8 |
Al3+ | mmolc dm-3 | 30.7 | 21.7 | 18.0 | 15.0 | 13.0 |
H + Al | mmolc dm-3 | 112.5 | 73.3 | 59.0 | 50.0 | 40.3 |
Ca2+ | mmolc dm-3 | 3.5 | 3.5 | 3.5 | 3.3 | 3.5 |
Mg2+ | mmolc dm-3 | 1 | 1 | 1 | 1 | 1 |
K+ | mmolc dm-3 | 0.63 | 0.45 | 0.38 | 0.40 | 0.35 |
Na+ | mmolc dm-3 | 0.2 | 0.1 | 0.1 | 0.2 | 0.1 |
ECEC | mmolc dm-3 | 35.98 | 26.75 | 22.93 | 19.73 | 17.90 |
m | % | 85 | 81 | 79 | 76 | 73 |
P | mg dm-3 | 3.8 | 3.7 | 1.4 | 1.5 | 1.2 |
Sand | g kg-1 | 753 | 771 | 794 | 779 | 747 |
Clay | g kg-1 | 133 | 140 | 153 | 138 | 140 |
Soil chemical analysis consisted of: pH-CaCl2 0.01 M (1:2.5 - soil:solution ratio), soil buffer to pH 7 (H + Al), exchangeable Al, Ca, and Mg (KCl 1 M - extraction), available P, K, Fe, Mn, Zn, and Cu (Mehlich I - extraction), and organic C according to procedures of Marques & Motta ([23]). Soil granulometric analysis of clay, silt, and coarse and fine sand was performed according to Donagema et al. ([13]) using the pipette methodology.
Experimental design
The experiment was a randomized block design with 7 treatments and 4 blocks. Parcels had 16 useful trees spaced 2 m within rows and 3 m between rows with two border rows. An 11-year-old pine stand in the second rotation with no history of fertilizing or liming was selected for the experiment. Prior to experimental setup, trees in this area exhibited low growth rates with some visible symptoms of nutrient deficiency. Using the omission diagnosis technique, treatments were manually applied in November 2008 and in January 2010. The amount of nutrients applied per year were: 40 kg ha-1 of N (urea); 60 kg ha-1 of P2O5 (triple super phosphate); 80 kg ha-1 of K2O (as potassium chloride); 3.0 kg ha-1 of Zn (zinc sulfate); 2.0 kg ha-1 of B (as ulexite); 1.5 kg ha-1 of Cu (cooper sulfate); and 20 g ha-1 of Mo (sodium molybdate). Additionally, lime with a total neutralizing power of 89.4% was applied as a Ca and Mg source at 1.300 kg ha-1 (28.9% and 20.6 % of CaO and Ca, respectively; 19.9% and 11.9 % of MgO and Mg, respectively). Lime was manually applied over the litter surface, followed by other nutrient applications on the same day. The treatments were: T1, complete; T2, minus macronutrients; T3, minus micronutrients; T4, minus K; T5, minus Zn; T6, minus lime; and T7, control with no lime or fertilizer.
Root and forest floor litter sampling
Forest floor litter was sampled in July 2012 using a square wooden template (100 cm2). Litter layers were classified according to Babel ([4]) as: new litter (NL); old litter (OL); and fragmented layer (FL). The NL fraction was characterized by recently fallen needles with little or no color change. The OL fraction was characterized by needles with color change. The FL fraction was characterized by fractured litter and was passed through a 2 mm sieve to separate into coarse fragmented layer (CFL) and fine fragmented layer (FFL). Dry mass and nutrient concentrations of fractions were determined. All roots were manually removed from the FFL for dry mass and nutrient concentration. In addition, roots samples were manually separated into thick and fine roots (< 1 mm diameter); fine roots were scanned for length and volume using WinRhizo™ (Regent Instrument Inc., Sainte-Foy, Quebec, Canada).
Litter and fine root nutrient measurements
After fraction separation, samples were oven dried at 60 °C until constant weight. For nutrient measurements, the dry digestion methodology of Martins & Reissmann ([25]) was used (500 °C with HCl 3 mol L-1). Concentrations of Ca, Mg, Mn, Fe, and Al were determined by atomic absorption spectrophotometry. Concentrations of K and Na were determined by flame spectrophotometry, and P concentration by the colorimetric method.
Tree growth
Tree growth was monitored by measuring diameter at breast height (DBH) and height of 16 trees in each plot. Measurements were performed in 2008 (start) and 2015 (end).
Statistical analysis
Data were subjected to Shapiro-Wilk and Bartlett tests to verify the assumptions of residual normality and variance homogeneity, respectively. Variables that did not present these characteristics were submitted to Box-Cox transformation. The Student-Newman-Keuls (SNK) test was used at the 5% probability level to check for possible differences between treatments. For tree growth data, the 10% probability level was used in the SNK test. After ensuring normality and homogeneity of data, a Principal Component Analysis (PCA) based on a correlation matrix was performed on litter layer elemental compositions and C:N ratio. Statistical analyses were performed using the R software.
Results and discussion
Forest floor litter fractions
Almost four years after treatment applications, significant differences were observed in new litter layer concentrations of Ca, Mg, and Na (Tab. S1 in Supplementary material); Ca and Mg increased while Na decreased in treatments with lime. Additionally, results indicated high concentrations of Al and Mn, which could be attributed to high soil acidity.
The highest K concentration for NL and OL was obtained by omission of lime (Ca and Mg source), suggesting that Ca and Mg led to reduced K absorption. The antagonistic effect of Ca and Mg on K is well known ([14]). Omission of K (-K treatment) lowered K concentration only in the fine fragmented layer. However, omission along with other macronutrients (-macro) did not result in decreased K. Thus, lime and micronutrient applications enhanced K uptake and maintenance for fall needs. The low concentration in treatments not receiving K was expected due to the low level in soil.
In general, K concentration was very low for both NL and OL. Rabel et al. ([37]) reported higher levels in old and new litter for a P. taeda experiment in a nearby state. In addition, it is well known that large portions of K can be redistributed at rates greater than 90% to growing parts of plants via translocation ([49]), especially under K deficiency. In this context, Rabel et al. ([37]) reported that the second needle flush had ten times more K than the new litter layer. This high rate of redistribution could be a possible explanation for the lack of response.
Potassium concentration in forest floor fractions diminished as a result of tissue age. Diminishing K was expected since it is weakly bound to organic matter and can be easily leached from residues ([31]). The observed decrease was not high, and it appears that the remaining K in dead needles stayed in tissue compartments susceptible to leaching. Precipitation passing through the canopy may have helped maintained K concentration.
In all forest floor layers analyzed, the major difference in composition was Ca and Mg concentrations (Tab. S1 in Supplementary material). These results were expected since Ca and Mg were added in larger amounts compared to P and K, and soil at the experimental site had low levels of these bases. The interaction of bases was observed in NL since the minus K treatment had the highest concentration of Mg and a trend for Ca.
Lime application overcame Ca and Mg loss due to forest floor aging in the control and enhanced concentration (more than 10 fold) in fragmented layers (Tab. S1). This accumulation in fragmented layers could be a result of adsorption by organic compounds and physical trapping of coarse lime particles. The presence of lime particles in coarse and fine fragmented layers was observed during sampling and laboratory processing. Large Ca and Mg increases in forest floor litter have been reported when lime has been applied ([27], [24], [17], [40]).
Although P concentration in NL was low, omission of this nutrient did not lead to further decreases, which was similar to findings for K (Tab. S1 in Supplementary material). As discussed for K, this could be related to high P redistribution from old to new tissue. In general, P concentration increased with litter age, possibly from P binding to organic compounds during the litter decay process ([31]). The minus lime and control treatments had lower P concentration in the CFL, but P concentration for these two treatments did not increase from OL to CFL as seen in the other five treatments. This could be related to a delay in litter decay. Under acidic conditions, Martins ([26]) reported an increase in P availability when lime application increased soil pH. The same author observed that a lack of response to fertilizer application can be associated with high translocation and the dilution effect.
Concentrations of Al and Fe were increased about three times in OL versus NL, with higher values being noted in FL (Tab. S1). These observations could be explained by a combination of three factors. First, the loss of CO2 during residue decay decreases mass and concentrates nutrients present in the residue. Second, elements that are strongly bond to organic matter undergo little impact from leaching; both Al and Fe are known to have strong adsorption capacity to organic matter ([19]). Third, decomposition of Pinus residue can result in large amounts of organic acids under very acidic conditions, which are then able to attack and solubilize some contacted soil minerals. Since Al and Fe are major components of mineral soils and are strongly bound to organic acids, they remain in solution in large amounts. After solubilization, acid solutions rich in Al and Fe can move upwards by capillarity action under dry conditions, thereby enhancing their concentrations in upper layers. The third explanation seems more plausible and was used by Trevisan ([47]) to explain high concentrations of these elements. Litter contamination by Al due to soil bioturbation was likely since there was an increase in total Al with litter age, but the proportion of Al weakly and strongly bound to organic matter was different ([39]). On the other hand, Rabel et al. ([37]) recently showed that these more aged fractions usually have mixed soil, which can overestimate the concentration of Fe and Al.
The C:N ratio decreased to less than half its value when comparing the least decomposed layer (NL) with the most decomposed layer (FFL - Tab. S1). Principal component analysis (PCA) indicated that PC1 explained 53.7% of data variance, and clearly showed the effect of litter aging (Fig. 1, Tab. S2 in Supplementary material). The less decomposed litter layers (NL and OL) were associated with a higher C:N ratio, while the opposite was true for the more decomposed layers. PCA also reinforced the strong effect of lime as evidenced by the position of the minus lime and control treatments, which were separated from other treatments for the same litter layer. These were also associated with lower concentrations of Mn, Ca, and Mg.
Fig. 1 - Principal component analysis of litter layer elemental compositions and C:N ratio for a Pinus taeda L. stand subjected to fertilizer and lime treatments in Paraná state, southern Brazil. Litter layers are represented by: new litter (circles); old litter (squares); coarse fragmented layer (triangles); fine fragmented layer (diamonds).
Acidity appeared to have a strong influence on Mn. For NL, the Mn concentration exhibited a trend to be higher for the control and minus lime treatments than limed treatments, which was expected since acidity increases soil Mn availability ([33]). In contrast, the high acidic conditions found in the minus lime and control treatments resulted in a great loss of Mn, which was reflected by a sharp decline from OL to CFL, followed by maintenance of concentration in FFL. The higher Mn loss when lime was not applied can be associated with low CEC and retention to forest floor organic material ([19]). The increase in total or exchangeable Mn forms in forest floor litter from liming has been observed under different conditions ([27], [24], [17]). In addition, Berg et al. ([9]) showed that Pinus species exhibited greater loss of Mn from litter compared to other plant species due to high acidity of their residues.
In general, Mn concentration increased from NL to OL but in smaller proportion than observed for Fe and Al. This was expected since contributions from soil contamination were less probable for Mn compared to Al and Fe given its lesser abundance in soil.
Composition of fine roots shown in Tab. 2indicated that treatments only affected Ca, Mg, Mn, and Na concentrations. Treatments that included lime displayed large increases in Ca and Mg root concentrations similar to that observed for litter fragmented fractions (Tab. S1 in Supplementary material). This result suggested that Ca and Mg found in these litter layers could be available to plants.
Tab. 2 - Element concentration (nutrients, Al, and Na) in fine roots from a Pinus taeda L. stand subjected to fertilizer and lime treatments in Paraná state, southern Brazil. Averages followed by the same letter in a column do not statistically differ (p>0.05) after Student-Newman-Keuls test. (CV): coefficient of variation.
Treatment | Ca (g kg-1) |
Mg (g kg-1) |
P (g kg-1) |
K (mg kg-1) |
Mn (mg kg-1) |
Fe (mg kg-1) |
Na (mg kg-1) |
Al (g kg-1) |
---|---|---|---|---|---|---|---|---|
Complete | 8.3 a | 2.7 a | 1.12 ns | 329.5 ns | 92.3 abc | 620 ns | 106.9 ab | 2.4 ns |
- Macro | 7.0 a | 2.6 a | 0.97 | 443.3 | 129.0 ab | 432 | 112.1 ab | 1.7 |
- Micro | 6.7 a | 2.4 a | 1.03 | 398.8 | 172.4 a | 521 | 69.2 c | 1.8 |
- K | 7.2 a | 2.5 a | 1.04 | 355.8 | 83.4 abc | 595 | 135.4 a | 1.5 |
- Zn | 7.6 a | 2.5 a | 1.01 | 365.1 | 35.7 bc | 597 | 88.6 b | 1.5 |
- Lime | 0.6 b | 0.1 b | 1.03 | 387 | 18.4 c | 551 | 138.9 a | 1.8 |
Control | 0.2 b | 0.1 b | 0.85 | 298.6 | 115.1 abc | 529 | 139.7 ab | 2.1 |
p-value | <0.01 | <0.01 | 0.05 | 0.08 | <0.01 | 0.58 | <0.01 | 0.31 |
CV | 25.9 | 9.7 | 10.4 | 17.1 | 51.5 | 25.9 | 0.66 | 33 |
Liming did not reduce root Al concentration despite the high enhancement of Ca and Mg. It is well known that Al is an important factor limiting pine tree development under extreme acidic conditions, and that Ca can mitigate Al toxicity, suggesting that the Ca:Al molar ratio can be used to diagnose Al toxicity ([48]). Thus, lime application that enhanced Ca concentration without changing Al concentration (as seen in our study - Fig. 2) indicated a diminishment of Al toxicity ([5]).
Fig. 2 - Molar ratio of Ca:Al concentrations in fine roots from a Pinus taeda L. (average ± standard deviation) stand subjected to fertilizer and lime treatments in Paraná state, southern Brazil.
The Ca:Al molar ratio critical threshold has been studied by many authors; 40% reported the critical threshold to be between 0.3-0.7, 50% reported a value between 0.2-0.3, and 10% reported values below 0.2 ([48]). The Ca:Al molar ratio for the treatments without lime and control showed that our studied pine stand was under Al toxicity.
There were slight changes in concentration of Na, K, and P that ranged from 69.2 to 139.7 mg kg-1, 298.6 to 443.3 mg kg-1, and 0.9 to 1.1 g kg-1, respectively. The lowest values for K and P were observed in the control treatment.
Higher amounts of Fe were found in roots (Tab. 2) than in the NL layer (Tab. S1 in Supplementary material); this suggests that upward translocation ability was greater for Mn than Fe. Roots had low values of Mn (Tab. 2), but high values in NL suggests more translocation of this nutrient to the aboveground biomass. The lowest Mn concentration was observed with omission of lime, which was in agreement with total concentration values observed in FL (Tab. S1). However, the concentration in the control was much higher than the minus lime treatment despite the low total concentration found in FL. More study is required to evaluate the effects of lime on root Mn concentrations.
Forest floor litter accumulation
The total amount of forest floor litter accumulation varied from 43.9 to 52.1 t ha-1 (Tab. 3), which was within the large range of 20.3 to 90.6 t ha-1 reported by Bizon ([10]) in a Pinus survey for this region. The amount of litter found in our study can be considered high and was indicative of a poor growth site with low soil fertility ([29], [50]).
Tab. 3 - Forest floor litter layer accumulation (t ha-1) from a Pinus taeda L. stand subjected to fertilizer and lime treatments in Paraná state, southern Brazil. (CV) coefficient of variation. Averages followed by the same letter do not statistically differ (p>0.05) after Student-Newman-Keuls test.
Treatments | Forest floor litter layers | ||||
---|---|---|---|---|---|
NL (t ha-1) |
OL (t ha-1) |
CFL (t ha-1) |
FFL (t ha-1) |
Total | |
Complete | 4.32 | 5.37 | 26.28 | 16.15 | 52.12 |
- Macro | 3.89 | 5.98 | 25.24 | 15.51 | 50.62 |
- Micro | 4.13 | 6.03 | 22.88 | 15.81 | 48.85 |
- K | 3.74 | 5.95 | 21.32 | 14.75 | 45.76 |
- Zn | 4.33 | 6.04 | 21.48 | 12.10 | 43.95 |
- Lime | 4.27 | 5.11 | 23.08 | 16.97 | 49.43 |
Control | 4.38 | 5.28 | 23.80 | 16.19 | 49.65 |
Average | 4.15 c | 5.68 c | 23.44 a | 15.35 b | - |
p-value Treatments | 0.62 | - | - | - | - |
p-value Layers | <0.01 | - | - | - | - |
p-value T × L | 0.98 | - | - | - | - |
CV Treatments (%) | 39 | - | - | - | - |
CV Layers (%) | 34.4 | - | - | - | - |
Poor growth sites are generally characterized by low soil fertility that leads to low soil microbiological activity. Plants grown under soil nutrient deficiency generally have low tissue nutrient concentrations that may restrict decomposition rates.
There was no difference in forest floor litter accumulation among treatments. Similar results for fertilization were reported by Moro ([28]) who evaluated stands of P. taeda that were one, five, and nine years old. Prescott ([35]) also observed that mass loss of pine forest floor litter was similar in control plots and plots fertilized with N or NPK.
The small variation in the amount of new litter layer mass ranging from 3.74 to 4.38 t ha-1 (Tab. 3) suggests similar needle fall regardless of treatment application. The combined amount of NL and OL represents only 17.1% to 23.6% of total forest floor litter. This amount was close to the annual litter depositions (6.4 and 9.3 t ha-1) observed by Bizon ([10]) under similar soil and climatic conditions and by Piovesan et al. ([32]) who found 7.1 t ha-1 in an 8-year-old pine plantation. Schumacher et al. ([45]) also reported that P. taeda (5 to 7 years old) had a deposition of 4.52 t ha-1 year-1. Our finding suggests that these litter layers were composed of recently fallen needles (one year old).
The CFL and FFL litter layers comprised 50% and 30% of total forest floor litter mass, respectively. Age of the fragmented residue was not established, but some portion of the litter layer may have been contributed by the first pine rotation since fire was not used to clear the area after the first rotation harvest. Forest floor litter composed of fine material could be more stable and a major reservoir of nutrients, as well as a source of energy for microorganisms.
In the case of fine roots (Fig. 3A), there was no significant effect, although increased biomass was observed in the complete treatment compared to the no lime treatment; this supports the Ca:Al ratio results. This shows that roots developed more when nutrient availability increased. Lehto ([21]) in Scotland observed that there was an increase in fine root biomass in Picea abies with liming (after 30 years) and boron (after 2 years). Under temperate conditions, Bakker et al. ([6]) found a decrease in fine root biomass of Pinus pinaster with the addition of nutrients (N, P, K, Ca, and Mg) in both the forest floor and soil compartments. Working with P. taeda, Albaugh et al. ([1]) found increases in total aboveground biomass and coarse roots with fertilization, but no change in fine root biomass, which indicated a greater allocation of metabolic resources to foliage (photosynthesizing tissue) over fine roots. Samuelson et al. ([41]) observed no change in P. taeda fine root biomass as a result of fertilization.
Fig. 3 - Dry mass (A), length (B), and volume (C) of fine roots (average ± standard deviation) present in forest floor litter from a Pinus taeda L. stand subjected to fertilizer and lime treatments in Paraná state, southern Brazil. (ns): not significant.
In general, fine root biomass obtained in this study was higher than seen in other studies (3.5 t ha-1 maximum) that considered forest floor litter and/or soil ([1], [41], [22]). Under limiting conditions, fine roots in pine forest floor litter play important roles in recycling nutrients and providing nutrients and water to trees ([22], [29]).
Omission of K alone (minus K) resulted in higher fine root volume, indicating that it may be a limiting element and that its absence stimulates plants to produce more roots for enhanced absorption of this nutrient. Yavitt et al. ([51]) showed root decreases with K application in a tropical forest in Panama during a 4 year evaluation. Following the same logic, Sayer et al. ([44]) studied nutrient cycling in a tropical forest and found that fine root activity was lower in sites with added K.
Nutrients and Al in forest floor litter
Total amounts of immobilized elements in forest floor litter are shown in Tab. 4, while values for individual litter layers are given in Tab. S3 (Supplementary material). Results from the control treatment followed the sequence C>N>Al>Fe>P>Ca>K>Mg>Mn>Na, while the complete treatment followed the sequence C>N>Ca>Al>Mg>P> Fe>Mn>K>Na. Matzner et al. ([27]) reported similar results for spruce forest floor litter with the order C>N>Fe>Al>Ca> P>K>Mg>Mn for the control and C>N> Ca>Fe>Mg>Al>P>Mn>K for fertilizer treatments. Given the observed total forest floor biomass values, C and N maintenance in forest floor litter were not affected by lime and fertilizer applications in contrast to findings of Huber et al. ([15]).
Tab. 4 - Total nutrients (C, N, Ca, Mg, P, K, Mn, and Fe), Al, and Na in forest floor litter from a Pinus taeda L. stand subjected to fertilizer and lime treatments in Paraná state, southern Brazil. Averages followed by the same letter in a column do not statistically differ (p>0.05) after Student-Newman-Keuls test. (CV): coefficient of variation.
Treatment | C (kg ha-1) |
N (kg ha-1) |
Ca (kg ha-1) |
Mg (kg ha-1) |
P (kg ha-1) |
K (kg ha-1) |
Mn (kg ha-1) |
Fe (kg ha-1) |
Al (kg ha-1) |
Na (kg ha-1) |
---|---|---|---|---|---|---|---|---|---|---|
Complete | 23,130 | 577 | 475 a | 135 a | 47.7 | 12.7 | 19.9 a | 44.5 | 268 | 4.38 |
- Macro | 21,023 | 525 | 562 a | 135 a | 43.5 | 13.3 | 21.8 a | 36.2 | 222 | 5.22 |
- Micro | 25,108 | 640 | 545 a | 144 a | 58.5 | 14.2 | 19.2 a | 44.9 | 235 | 4.47 |
- K | 20,401 | 515 | 376 a | 107 a | 45.6 | 10.3 | 18.1 ab | 31.0 | 200 | 4.17 |
- Zn | 18,911 | 486 | 440 a | 107 a | 43.9 | 11.6 | 18.1 ab | 35.0 | 180 | 3.46 |
- Lime | 23,162 | 578 | 46 b | 11 b | 38.8 | 13.0 | 5.4 bc | 35.5 | 215 | 4.41 |
Control | 22,266 | 584 | 31 b | 10 b | 31.8 | 10.3 | 4.8 c | 36.7 | 205 | 4.20 |
p-value | 0.47 | 0.33 | <0.01 | <0.01 | 0.06 | 0.28 | <0.01 | 0.36 | 0.66 | 0.36 |
CV | 18.9 | 16.9 | 34.3 | 22.3 | 23.4 | 21.2 | 37.7 | 25.2 | 31.2 | 22.2 |
Again, the main observed differences were related to Ca and Mg, with no differences for other macronutrients (N, P, K). Rosberg et al. ([40]) and Marschner & Wilczynski ([24]) reported large increases in Ca (213-1476 kg ha-1 and 140-1540 kg ha-1, respectively) and Mg (34-690 kg ha-1 and 40-107 kg ha-1, respectively) from liming and fertilization, but low increases in N (1092-1159 kg ha-1 and 1060-930 kg ha-1, respectively), P (62-74 kg ha-1 and 89-102 kg ha-1, respectively) and K (57-72 kg ha-1 and 34-41 kg ha-1, respectively).
As previously discussed, a portion of applied lime was trapped in CFL and FFL and could be visually observed during sample manipulation. Huber et al. ([15]) reported the complete dissolution of 4 t ha-1 of dolomitic lime 6 years after application, which confirms the slow solubility of lime applied over forest floor litter. Some lime was probably solubilized and Ca and Mg were adsorbed to negative charges associated with forest floor litter ([17]). This supports observations of Rosberg et al. ([40]) who found that ~90% of Ca applied as lime was found in forest floor litter four years after application.
The observed increase in Ca was proportionally higher than Mg since the Ca:Mg ratio of the utilized lime was 1.7:1, while the forest floor litter had a 3:1 ratio. Since there is an inverse relationship between adsorption strength and loss by leaching, the higher adsorption capacity of Ca compared to Mg resulted in the maintenance of Ca in forest floor litter. In a long-term study, Huber et al. ([15]) also found much higher leaching of Mg than Ca.
Treatments with lime increased Mn in litter, similar to observations by Marschner & Wilczynski ([24]) and Matzner et al. ([27]). Huber et al. ([15]) found a decrease of Mn due to lime application in seepage water at a 40 cm depth, confirming its maintenance in forest floor litter and soil.
Matzner et al. ([27]) also reported increased Fe and Al, while Marschner & Wilczynski ([24]) did not report differences. Matzner et al. ([27]) suggested that soil bioturbation could be a reason for increases in Al, Fe, and Mn; this was not observed in our study. Thus, the low amount of Mn under acidic conditions could be related to the adsorption capacity of organic matter.
Observed results also indicated that forest floor litter can be a major source of P when fertilizer and lime were not applied. This was likely true in our study since P was found in high amounts in forest floor litter and was less exported and extracted by pine trees ([26]).
In contrast, forest floor litter displayed little potential as a K source since low amounts were available to meet the large demands of P. taeda. This was expected due to the redistribution capacity from old growth to new growth. Thus, K was redistributed from needles prior to senescence and litterfall ([49]).
Tree growth
Small increases in DBH and tree height (similar to control) as a result of lime omission (Fig. 4) suggest a probable lack of Mg and/or Ca. Since Mg availability was lower than Ca and was very low within the 0-60 cm depth (Tab. 1), it is plausible that Mg was more limiting when lime was omitted. Omission of lime also resulted in the appearance of chlorotic symptoms in needles tips (yellowish color), especially in the first needle flush. These symptoms resembled the lack of Mg reported for Pinus radiata (D. Don) plantations in New Zealand that displayed distal yellowing of older needles ([52]).
Fig. 4 - Diameter at breast height (DBH) and height increases (average ± standard deviation) from 2008 to 2015 for each treatment.
At our study site, omission of K produced the highest tree growth despite extremely low soil K levels. Potassium levels in plants may be highly associated with non-exchangeable K in soil, indicating an ability of P. taeda to acquire soluble forms ([3]). In addition, the well known antagonistic interaction between K and Mg ([46], [12]) can decrease Mg uptake when K is applied.
Conclusions
There were high increases in Ca and Mg concentrations in forest floor litter due to the high amounts of lime applied. Better growth was observed with lime application since lime is a good source of Ca and Mg that provides more optimal conditions for fine root growth and can lead to decreased Al toxicity. Also, symptoms of Mg deficiency with lime omission illustrates the importance of dolomitic lime in Pinus taeda forests having very low levels of available soil Mg. In our poor soil system, K was not present in great quantities in litter and fine root fractions likely due to the efficient redistribution capacity of pine trees from old growth to new growth. Fertilizer addition caused little variation in the amount of accumulated forest floor litter, indicating a balance of inputs and outputs or that nutrition had little impact on forest floor litter decomposition. Forest floor litter acted as a nutrient pool and had the following order of availability under control conditions: C>N>Fe>P>Ca>K>Mg>Mn. Under amended conditions the sequence was: C>N>Ca> Mg>P>Fe>Mn>K. Lime application increased the amount of Ca and Mg in litter but had no effect on C, N, K, and P.
Acknowledgements
The authors thank the staff (Renato Teixeira Lima, Antônio - Mineiro, Felipe Mazurki Perucio) of the “Vale do Corisco” and “Valor Florestal” Companies for field work support, and Barry Dorman of the USDA-ARS National Soil Dynamics Laboratory for technical review of the manuscript. ACVM is grateful to the National Council for Scientific and Technological Development of Brazil (CNPq) for financial support and to the “Coordination for the Improvement of Higher Education Personnel” (CAPES, Brazil) for scholarship support.
References
Gscholar
Gscholar
Gscholar
Gscholar
Gscholar
Gscholar
Gscholar
Gscholar
Gscholar
CrossRef | Gscholar
CrossRef | Gscholar
Gscholar
CrossRef | Gscholar
Authors’ Info
Authors’ Affiliation
Epagri - Empresa de Pesquisa Agropecuária e Extensão Rural de Santa Catarina, Florianopolis, SC (Brazil)
Ederlan Magri 0000-0001-5046-4653
Antônio Carlos Vargas Motta 0000-0001-9117-1881
Rubia Luciane Dominschek Lima 0000-0002-4240-0147
Department of Soils and Agricultural Engineering, Federal University of Paraná, 1540 Funcionários St., Curitiba, PR (Brazil)
USDA-ARS National Soil Dynamics Laboratory, Auburn, Alabama (USA)
Corresponding author
Paper Info
Citation
Adam WM, Rodrigues VDS, Magri E, Motta ACV, Prior SA, Moraes Zambon L, Lima RLD (2021). Mid-rotation fertilization and liming of Pinus taeda: growth, litter, fine root mass, and elemental composition. iForest 14: 195-202. - doi: 10.3832/ifor3626-014
Academic Editor
Gianfranco Minotta
Paper history
Received: Aug 14, 2020
Accepted: Feb 18, 2021
First online: Apr 24, 2021
Publication Date: Apr 30, 2021
Publication Time: 2.17 months
Copyright Information
© SISEF - The Italian Society of Silviculture and Forest Ecology 2021
Open Access
This article is distributed under the terms of the Creative Commons Attribution-Non Commercial 4.0 International (https://creativecommons.org/licenses/by-nc/4.0/), which permits unrestricted use, distribution, and reproduction in any medium, provided you give appropriate credit to the original author(s) and the source, provide a link to the Creative Commons license, and indicate if changes were made.
Web Metrics
Breakdown by View Type
Article Usage
Total Article Views: 32878
(from publication date up to now)
Breakdown by View Type
HTML Page Views: 28567
Abstract Page Views: 1997
PDF Downloads: 1867
Citation/Reference Downloads: 2
XML Downloads: 445
Web Metrics
Days since publication: 1483
Overall contacts: 32878
Avg. contacts per week: 155.19
Article Citations
Article citations are based on data periodically collected from the Clarivate Web of Science web site
(last update: Mar 2025)
Total number of cites (since 2021): 5
Average cites per year: 1.00
Publication Metrics
by Dimensions ©
Articles citing this article
List of the papers citing this article based on CrossRef Cited-by.
Related Contents
iForest Similar Articles
Research Articles
Effects of stand age on litter quality, decomposition rate and nutrient release of Kazdagi fir (Abies nordmanniana subsp. equi-trojani)
vol. 13, pp. 396-403 (online: 03 September 2020)
Review Papers
Recent insights in soil nutrient cycling: perspectives from Pinus and Eucalyptus forest studies around the world
vol. 17, pp. 394-404 (online: 20 December 2024)
Research Articles
Soil stoichiometry modulates effects of shrub encroachment on soil carbon concentration and stock in a subalpine grassland
vol. 13, pp. 65-72 (online: 07 February 2020)
Research Articles
The manipulation of aboveground litter input affects soil CO2 efflux in a subtropical liquidambar forest in China
vol. 12, pp. 181-186 (online: 10 April 2019)
Research Articles
Effects of arbuscular mycorrhizal fungi on microbial activity and nutrient release are sensitive to acid deposition during litter decomposition in a subtropical Cinnamomum camphora forest
vol. 16, pp. 314-324 (online: 13 November 2023)
Research Articles
Soil fauna communities and microbial activities response to litter and soil properties under degraded and restored forests of Hyrcania
vol. 14, pp. 490-498 (online: 11 November 2021)
Research Articles
No home-field advantage in upper Andean tropical forests despite strong differences in site environmental characteristics
vol. 17, pp. 286-294 (online: 27 September 2024)
Research Articles
Effect of plant species on P cycle-related microorganisms associated with litter decomposition and P soil availability: implications for agroforestry management
vol. 9, pp. 294-302 (online: 05 October 2015)
Research Articles
Soil respiration along an altitudinal gradient in a subalpine secondary forest in China
vol. 8, pp. 526-532 (online: 01 December 2014)
Short Communications
Variation in soil carbon stock and nutrient content in sand dunes after afforestation by Prosopis juliflora in the Khuzestan province (Iran)
vol. 10, pp. 585-589 (online: 08 May 2017)
iForest Database Search
Search By Author
Search By Keyword
Google Scholar Search
Citing Articles
Search By Author
Search By Keywords
PubMed Search
Search By Author
Search By Keyword