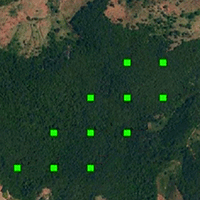
Contribution of legume and non-legume trees to litter dynamics and C-N-P inputs in a secondary seasonally dry tropical forest
iForest - Biogeosciences and Forestry, Volume 15, Issue 1, Pages 8-15 (2022)
doi: https://doi.org/10.3832/ifor3442-014
Published: Jan 13, 2022 - Copyright © 2022 SISEF
Research Articles
Abstract
Many studies have investigated nutrient cycling in seasonally dry tropical forests, but few have assessed the contribution of different functional groups to these processes. Here, we investigated general litter dynamics patterns and the contribution of legume and non-legume trees to litter dynamics and carbon (C), nitrogen (N), and phosphorus (P) inputs in a fragment of secondary seasonally dry tropical forest after half a century of forest succession in the Atlantic Forest biome in Brazil. Between 2016 and 2017, we quantified litterfall production, canopy cover, forest floor, and soil C and N storage in 11 permanent plots distributed in the fragment. Vegetation identity and structure had been previously assessed. We quantified the seasonal inputs of leaf litter and C, N, and P separately for each functional group (legume and non-legume tree species). We also analyzed the correlations between the variables measured for each functional group with the variables measured at the plot level. Litter dynamics and nutrient input were affected by climate and functional group. Litterfall production during the two driest months was three times higher than during the other periods of the year, suggesting that species synchronicity is likely to minimize drought-related damage on trees. Legume trees had twice the basal area attained by non-legume trees, but while legumes were larger, non-legumes were more abundant and dominant in the smaller diameter class. Legumes deposited twice as much N during the driest period of the year as non-legumes. Although leaf litter, C, and P inputs by legumes were generally higher than those of non-legumes, these differences during the dry season were not statistically significant. We also found that the legume variables correlated better with the plot-level variables, compared to the non-legume functional group. Our results also indicated potential effects of the leaf litter and nutrient inputs by the legume functional group on the decomposition constant and, consequently, on the time of forest floor decomposition. Further studies should assess the role of different functional groups in litter dynamics and nutrient inputs in seasonally dry tropical forests.
Keywords
Nutrient Cycling, Litterfall, Nutrient Input, Canopy Cover, Decomposition Rate, Fabaceae, Atlantic Forest
Introduction
Seasonally dry tropical forests (SDTF) represent 40% of the world’s tropical forests ([7]) and are distributed in regions of Africa, Australia, central and southern Latin America, India, and Southeast Asia ([9]). In Brazil, SDTFs occur where mean annual precipitation ranges between 700 mm and 2000 mm ([33]) and decreases below 30 mm during the dry season ([30]). The several SDTF remnants in Brazil add up to an area of 27.6 million hectares, which is equivalent to 3.2% of the original SDTF occurrence range ([13]). These fragments have different sizes, disturbance histories, and successional stages ([40], [30]).
The Fabaceae family, or legume family, is the most diverse in Brazil, with approximately 2807 species ([14]). Among its subfamilies, Mimosoideae, Faboideae, and Caesalpinioideae, it is estimated that 96%, 87%, and 24% of species, respectively, can perform symbiotic biological nitrogen fixation in association with bacteria ([6], [28]). Several species of the legume family play an important role in tropical forest succession, by colonizing abandoned pastures and agricultural areas in tropical regions ([12]). Biological nitrogen fixation is an important promoter of secondary succession in tropical rainforests ([4]) and SDTFs ([40]). N2-fixing legumes can provide more than 50% of the total nitrogen needed for forest growth in the first 12 years of secondary succession and approximately 8% of such in mature forests (> 100 years - [4]). N2-fixing ability provides a competitive advantage to trees during forest succession ([43]), but biological nitrogen fixation requires more energy from the plant than mineral N uptake directly from the soil ([35]).
Legume abundance and dominance are generally higher in secondary forests at advanced successional stages (> 50 years) in a seasonally dry tropical forest ([40]) than in wet tropical regions ([4]). Thus, forest productivity and nutrient inputs in SDTFs should be highly influenced by the performance of the legume functional group after long-term forest succession. Canopy cover variations in forest ecosystems are expected to influence understory productivity and solar radiation availability. Canopy cover could also be influenced by the spatial variability in abundance and dominance of different functional groups, such as legume and non-legume species, and evergreen and deciduous trees ([23]). However, N and P inputs by legume species are better understood in forest plantations and agroforestry systems ([11], [47]) than in biodiverse forests, both wet and dry ([38], [40], [4]). For example, plantations of pioneer legume trees, such as Acacia, Leucaena, and Albizia, may add to the soil over 130 kg ha-1 year-1 of N via leaf litter ([6]). The amounts of litterfall and nutrients deposited annually on SDTF soils are relatively well known; for litterfall, these values range between 4500 and 8000 kg ha-1 year-1 ([8], [34]); for N, between 102 and 300 kg ha-1 year-1; and for P, between 4 and 12 kg ha-1 year-1 ([10]).
Leaf litter production is an important indicator of primary production in SDTFs ([7], [27]). Accumulated leaf litter, mainly during the dry season, represents a nutrient reserve which favors tree growth in the next growing season ([3], [41]). Studies have shown a positive relationship between soil nutrient availability and the deposition of biomass, carbon, and nutrients on the forest floor ([15], [47]). Leaf litter decomposition rates are influenced by leaf litter quality (e.g., N content, C/N ratio, N/P ratio, lignin content, and polyphenol content - [16], [7]), which varies spatially depending on the abundance and dominance of different tree species. In addition, seasonal and local changes in water availability affect tree phenology, leaf litter input, and decomposition rates, the latter due to impacts on soil microbial activity ([9], [25]). As far as we know, few studies have assessed the influence of legume trees on litter decomposition ([38]).
Here, we investigate general litter dynamics processes and the contribution of different functional groups to litter dynamics and C, N, and P inputs in a 50-year-old secondary seasonally dry tropical forest of the Atlantic Forest biome in Brazil. We also assessed the correlations between the variables measured in the two functional groups and the variables measured at the plot level. We assessed the following: (i) general patterns of litterfall production and decomposition, canopy cover, and soil C and N storage in the forest fragment; and (ii) the influence of two functional groups (legumes and non-legumes) on litter dynamics and C, N, and P inputs in the forest fragment. We hypothesize that the legume functional group affects litter dynamics and nutrient inputs even after half a century of secondary succession in the studied SDTF.
Material and methods
Characterization of the study site
The study was carried out in a secondary SDTF fragment located in the Environmental Education Center at the Federal Institute of Espírito Santo, in the municipality of Alegre, Espírito Santo state, Brazil (Fig. 1). The study region has a mean annual precipitation (MAP) of 1305 mm and a mean annual temperature (MAT) of 23.7 °C ([17]). The driest period is comprised between June and August, when precipitation is below 30 mm, though the dry season extends between May and September when it is overall lower than 50 mm ([17]). These climatic conditions favor the occurrence of seasonally dry forest formations in the Atlantic Forest biome, which are officially classified in Brazil as semideciduous seasonal forests (“Floresta Estacional Semidecidual” - [46], [30]). The fragment has a total area of approximately 109 ha with varying disturbance histories, including coffee plantations, pasture for livestock, and selective harvesting. These disturbances have barely influenced the basal area of the forest after 50 years of natural regeneration ([21]). The altitude at the site varies between 120 and 660 m a.s.l., with an undulating relief ranging between 3% and 45% in slope ([21]). Latosol predominates in the study site with an average sand content of 56%, an average clay content of 34%, a pH of 5.2, 2.5 mg dm-3 of P, and 9.1 cmolc dm-3 of total soil exchange capacity ([22]).
Fig. 1 - Plot distribution throughout the study site. (a): Map of Brazil; (b): map of the Espírito Santo state; (c) orthophoto of the forest fragment.
The data were collected for one year, from September 2016 to September 2017, in 11 permanent plots of 400 m² (20 × 20 m) that were distanced 200 m from each other (Fig. 1). These plots were originally installed by Lorenzoni-Paschoa ([22]) to conduct a phytosociological study in the site. In 2014 and 2015, all trees with a diameter at breast height equal to or higher than 5 cm in the plot had their diameters measured and most were identified to the species level ([21]). Tree abundance, defined as the number of trees identified in the plots standardized per hectare, was higher among non-legumes than among the legume functional group in the diameter classes below 15 cm (Fig. 2a). Tree dominance was defined as the sum of basal areas (in m²) of trees identified in the plots standardized per hectare. Non-legumes were dominant in the smaller diameter class and legumes were dominant in the largest diameter classes (Fig. 2a). The basal area of unidentified trees represented less than 1% of the total basal area of the plots.
Fig. 2 - Abundance and dominance of functional groups in the study site. (a): Abundance (circles) and dominance (bars) of legume (black) and non-legume trees (grey) by diameter class with an amplitude of 2 cm. (b) Abundance (triangle) and dominance (bars) percentage of the species of major importance value. The legume species are indicated by arrows.
Most legume trees identified in the plots were deciduous and about 50% of them were capable of biological N2 fixation (Tab. 1). Among the 11 species with the greatest abundance and dominance values in the plots, six belong to the legume functional group and five to the non-legume functional group (Fig. 2b). These non-legume species were Alseis pickelli Pilg. & Schmale, Erythroxylum pulchrum A. St. Hill., Cariniana ianeirensis R. Knuth, Trichilia casareti C. DC., and Astronium concinnum Schott.
Tab. 1 - Legume trees recorded in the study site along with their subfamilies and information on leaf deciduousness and N2-fixing capacity based on the literature. (F): N2-fixing; (NF): non-N2-fixing. Source: [21] (adapted).
Species | Subfamily | Deciduous | N2-fixing |
---|---|---|---|
Pseudopiptadenia contorta (DC.) | Mimosoideae | Y | - |
Parapiptadenia pterosperma (Benth.) | Mimosoideae | Y | F |
Apuleia leiocarpa (Vogel) | Caesalpinioideae | Y | NF |
Dalbergia nigra (Vell) | Faboideae | Y | F |
Senegalia sp. | Mimosoideae | Y | F |
Peltophorum dubium (Spreng.) Taub. | Caesalpinioideae | Y | NF |
Amburana cearensis (Allemão) A.C.Sm. | Faboideae | Y | F |
Myrocarpus frondosus Allemão | Faboideae | Y | - |
Lonchocarpus sericeus (Poir.) Kunthex DC. | Faboideae | Y | F |
Copaifera lucens Dwyer. | Caesalpinioideae | Y | F |
Adenanthera colubrina (Vell.) Brenan | Mimosoideae | Y | F |
Machaerium inincorruptibile Vogel | Faboideae | Y | F |
Machaerium paraguariense Hassl. | Faboideae | Y | F |
Acosmium lentiscifolium Schott | Faboideae | N | - |
Machaerium nyctitan (Vell.) Benth. | Faboideae | Y | - |
Platymiscium floribundum Vogel | Faboideae | N | F |
Bauhinia forficata Link | Caesalpinioideae | Y | F |
Poeppigia procera C. Presl | Caesalpinioideae | N | NF |
Machaerium pedicelatum Vogel | Faboideae | Y | F |
Platypodium elegans var. major Benth. | Faboideae | Y | F |
Pseudopiptadenia sp. | Mimosoideae | Y | - |
Swartzia acutifolia Vogel | Faboideae | N | F |
Inga hirsuta G. Don | Mimosoideae | N | F |
Barnebydendron riedelii (Tul.) J. H. Kirkbr | Caesalpinioideae | Y | - |
Senegalia kallunkiae (J. W. Grimes & Barneby) Seigler & Ebinger | Mimosoideae | Y | F |
Soil carbon and nitrogen storage
We obtained a composite topsoil sample (0-20 cm) for N and C analysis collected with the aid of an auger at five points in each plot. We measured soil bulk density of three soil layers (0-5, 5-10, and 10-20 cm) at two points per plot, using a metal cylinder with a diameter of 5.3 cm and a volume of 106.7 cm³. The soil samples used to assess bulk density were weighed on a precision balance after being dried at 105 °C for 72 h. The soil samples used to determine N and C contents were air-dried for 15 days, macerated in a mortar, and sieved (0.5 mm). Total soil N was determined using the Kjeldahl methodology, and total soil C was determined following Barrie & Prosser ([5]) using a 20-20 Hydra mass spectrometer (ANCA-GSL, SERCON Co., Crewe, UK).
Litterfall collection
In each plot, we installed four round collectors with an area of 0.196 m2 at 50 cm high, totaling 44 collectors over the entire site. We arranged the collectors systematically in a zigzag fashion within each plot, ensuring a minimum distance of 5 m between each collector and the plot’s edges. The litterfall was collected monthly between October 25, 2016, and September 25, 2017. After each collection, the material was dried and separated into leaf and miscellaneous fractions. We then separated the leaf fraction between legume trees (by mixing the leaves of trees belonging to the Fabaceae family) and non-legume trees (by mixing the leaves of trees belonging to other plant families). We were able to visually separate the leaves (aided by herbarium exsiccates of the recorded species) because of distinguishable leaf size differences between the two functional groups. After separation, the different fractions were dried at 60 °C for three days and then weighed in an analytical balance. The dry litter mass contained in each collector was converted to a hectare basis.
Leaf litter carbon, nitrogen, and phosphorus contents
We separately determined the concentrations of C, N, and P in the leaves of legume and non-legume trees. In each plot, the leaves of each functional group were mixed every three months and ground in a Wiley mill (1-mm mesh). To quantify leaf litter C, we used the dry mass loss on ignition method, as described by Kiehl ([19]). To quantify leaf litter N and P, we followed Tedesco et al. ([44]) and Malavolta et al. ([24]). N was determined through the Kjeldahl method, using sulphuric acid for wet digestion and sodium hydroxide for distillation. P was determined using nitro-perchloric digestion and optical spectrophotometry. The C, N, and P concentrations were then multiplied by the leaf litter mass of each functional group (legume and non-legume) in each plot to estimate C, N, and P inputs in each season: spring (October-December), summer (January-March), autumn (April-June), and winter (July-September).
Forest floor and decomposition constant
We sampled forest floor litter in two separate occasions, at the end of the rainy season (April 2017) and at the end of the dry season (September 2017), using a square frame (25 × 25 cm) set up beside the collectors at four points per plot. The material was sieved (2 mm mesh) to remove the soil, dried at 60 °C for five days, and weighed on an analytical balance. The dry matter accumulated on the forest floor was converted to a hectare basis. For each plot, we estimated the decomposition rates during the rainy season, during the dry season, and over the entire year of observation. This was achieved by dividing litterfall mass by forest floor mass, through the instantaneous rate of decomposition (k - [31]). We then calculated the time required for 50% of the material to be decomposed using a factor of 0.693 divided by k ([37]).
Canopy cover
The canopy cover index ([45]) was measured in four points in each plot on one occasion at the end of each season using a hemispheric fisheye lens attached to a smartphone. On cloudy days, canopy cover was preferably assessed during the early morning. The photographs were taken next to the litterfall collectors and pointed towards the direction of the magnetic north, respecting the zenith angle ([45]). The images were processed in the GLAMA application (Gap Light Analysis Mobile App).
Data analysis
The data used in our analyses were collected in multiple plots established in a natural forest with intrinsically variable structure and heterogeneous resource availability. Our main goal in analyzing these data was to find significant differences in leaf litter and nutrient inputs between the two functional groups (legume and non-legume trees). Thus, we used the non-parametric Mann-Whitney rank-sum test to compare leaf litter and nutrient inputs between legumes and non-legumes. We also calculated Spearman’s correlations between the variables measured in each functional group and the plot-level variables. The analyses were performed with the software SigmaPlot® v. 13.0 ([42]) using a statistical threshold value of 0.05.
Results
General patterns
During the entire year of collection, 8611 kg ha-1 of litterfall were produced. During the wet season (six months between October and March), litterfall production was 3202 kg ha-1, and during the dry season (six months between April and September) 5409 kg ha-1 of litterfall were produced. Litterfall production varied little during the rainy months, with an average of approximately 500 kg ha-1 month-1 (Fig. 3a). In contrast, the litterfall production was higher than 1500 kg ha-1 month-1 in the driest months (August and September). Also, the highest litterfall production coincided with drought events during the autumn and the winter, in which the temperatures varied between 15 and 25 °C and the accumulated precipitation was lower than 10 mm. The contribution of miscellaneous fractions to the litterfall produced was 1344 kg ha-1 in the rainy season and 593 kg ha-1 in the dry season. On average, the leaf litter made up 77% of the total litterfall collected during the year of observation.
Fig. 3 - Intra-annual variation of litterfall, canopy cover, and climate in the study site. The monthly litterfall production (black squares), daily precipitation (grey bars), and daily mean air temperature (grey circles) are shown with the seasons highlighted on top (a). The relationship between leaf litter and canopy cover index throughout the seasons in the experimental plots are shown along with their respective values of accumulated precipitation (mm) and mean air temperature (°C) in the top table (b). The study period began after installing the litterfall collectors at the end of September. Error bars indicate the standard deviation (n=11).
The seasonal production of leaf litter was inversely related to changes in the canopy cover and the precipitation regime over the year (Fig. 3b). Canopy cover during spring, summer, and autumn was, on average, 78%, 73%, and 68%, respectively. During these seasons, the site received a total of 1186 mm of precipitation and produced a total of 1659 kg ha-1 of litterfall. In contrast, during the winter, canopy cover in the site decreased to 45%, accumulated precipitation was 43 mm, and a total of 2057 kg ha-1 of litterfall were produced. Therefore, the leaf litter produced during the three winter months coincided with the observed reduction in canopy cover, which was about 30% on average.
The amount of litter deposited on the forest floor was higher during the dry season, compared to the rainy season, with an annual average of 8314 kg ha-1 (Tab. 2). The litterfall and forest floor mass were statistically indistinguishable in the study area. The decomposition constant during the dry season was higher than during the rainy season. The k value calculated over the year of observations ranged between 0.8 and 2.3 in the plots, with a mean value of 1.0. The annual decomposition rate implied that 244 days are needed to decompose half of the litter accumulated on the forest floor (Tab. 2).
Tab. 2 - Mean values (± standard error, n=11) of litterfall and forest floor mass, decomposition constant, and time of half-life of litter in the forest fragment.
Variable | Dry season | Rain season | Annual average |
---|---|---|---|
Litterfall (kg ha-1) | 5408.9 ± 729.0 | 3201.8 ± 404.4 | 8610.7 ± 1560.6 |
Litter on the forest floor (kg ha-1) | 9180.7 ± 5108.9 | 7446.7 ± 3430.8 | 8313.7 ± 1226.1 |
Decomposition constant (k) | 0.6 | 0.4 | 1.0 |
T50% | 1.2 | 1.6 | 0.7 |
Half-life (in days) | 429 | 588 | 244 |
C storage at 0-20 cm of soil depth ranged between 30,806 to 88,996 kg ha-1 in the plots, with a mean value of 57,029 kg ha-1. The mean value of soil N storage was 5172 kg ha-1, with minimum and maximum values of 3241 and 9493 kg ha-1, respectively. Soil C and N storage correlated significantly with one another, with a correlation coefficient (r) of 0.864. Soil C (r = 0.591, p=0.051) and soil N (r = 0.773) were also positively related to forest floor mass in the plots. Furthermore, the decomposition rate constant k correlated significantly with soil N storage (r = 0.673), but not to soil C storage (r = 0.509, p=0.102).
Contribution of the functional groups to litter dynamics and C, N, and P inputs
The seasonal and annual leaf litter inputs were not statistically different between functional groups (Tab. 3). However, legume species contributed, on average, 25% more to the annual leaf litter production than non-legumes. In addition, during the winter, the leaf litter input from legume species was about 50% higher than that of non-legumes.
Tab. 3 - Mean values (± standard error, n=11) of leaf litter mass and contents of carbon (C), nitrogen (N), and phosphorus (P) in legume and non-legume leaf litters collected in the study site. Different letters indicate significant differences (p<0.05).
Season | Group | Leaf litter (kg ha-1) |
N (%) |
C (%) |
P (%) |
N (kg ha-1) |
C (kg ha-1) |
P (kg ha-1) |
---|---|---|---|---|---|---|---|---|
Spring |
Leg | 493.3 ± 298.5 a | 2.8 ± 0.2 a | 48.1 ± 3.2 a | 0.15 ± 0.01 a | 14.0 ± 8.8 a | 227.3 ± 142.9 a | 0.7 ± 0.4 a |
Non-Leg | 511.5 ± 454.9 a | 2.5 ± 0.6 a | 47.3 ± 5.1 a | 0.12 ± 0.03 b | 12.6 ± 9.1 a | 241.9 ± 224.7 a | 0.6 ± 0.4 a | |
Summer |
Leg | 469.4 ± 269.4 a | 2.7 ± 0.3 a | 52.7 ± 4.8 a | 0.11 ± 0.02 a | 12.9 ± 7.1 a | 247.5 ± 143.1 a | 0.5 ± 0.5 a |
Non-Leg | 383.3 ± 176.5 a | 2.4 ± 0.5 a | 51.6 ± 13.0 a | 0.11 ± 0.01 a | 9.0 ± 3.9 a | 198.0 ± 106.3 a | 0.4 ± 0.2 a | |
Autumn |
Leg | 696.5 ± 403.9 a | 2.2 ± 0.5 a | 49.9 ± 1.6 a | 0.10 ± 0.07 a | 15.6 ± 11.5 a | 347.4 ± 207.2 a | 0.7 ± 0.3 a |
Non-Leg | 664.2 ± 383.7 a | 1.6 ± 0.5 b | 47.8 ± 5.4 a | 0.08 ± 0.01 a | 10.9 ± 9.9 a | 317.6 ± 198.2 a | 0.5 ± 0.3 a | |
Winter |
Leg | 2056.6 ± 924.8 a | 1.9 ± 0.2 a | 51.7 ± 1.2 a | 0.05 ± 0.01 a | 40.1 ± 20.0 a | 1064.3 ± 487.5 a | 1.0 ± 0.5 a |
Non-Leg | 1398.1 ± 554.7 a | 1.5 ± 0.2 b | 50.3 ± 1.1 a | 0.06 ± 0.01 a | 21.2 ± 7.8 b | 703.6 ± 278.4 a | 0.8 ± 0.4 a | |
Annual |
Leg | 3715.9 ± 500.9 a | - | - | - | 83.4 ± 12.4 a | 1905.3 ± 256.7 a | 2.8 ± 0.4 a |
Non-Leg | 2955.2 ± 342.5 a | - | - | - | 53.5 ± 5.8 a | 1476.3 ± 170.3 a | 2.3 ± 0.3 a |
The concentrations of C, N, and P throughout the seasons were often higher among legume leaves compared to non-legume leaves (Tab. 3). However, the only statistically significant difference in this regard was found for N concentration over the autumn and the winter and for P concentration during the spring.
The seasonal and annual inputs of C, N, and P via leaf litter were generally higher among legume trees compared to non-legume trees (Tab. 3). However, statistical differences between the groups were only found for N input over the winter, when legumes provided 90% more N than non-legumes. The C/N ratio for legume and non-legume leaf litters showed no significant differences, varying between 17 and 22 (spring), 22 and 24 (summer), 24 and 35 (autumn), and 27 and 34 (winter), respectively.
The legume trees had a higher basal area than non-legume trees in the assessed plots (15.4 vs. 10.1 m2 ha-1). In general, the variables measured in the legume functional group correlated with the variables measured at the plot level (Tab. 4). The variables measured in the non-legume functional group, such as leaf litter and leaf litter C, N, and P contents tended to positively correlate with canopy cover and leaf P input. Legume basal area correlated positively and significantly with plot-level basal area, leaf litter, and leaf litter C and N. Also, legume inputs of leaf litter C, N, and P correlated positively and significantly with plot-level leaf litter, C, N, and P. Moreover, the two functional groups often showed antagonistic patterns, although not significant in most cases. Antagonistic patterns were found between litterfall production, leaf litter, forest floor leaf nutrients, canopy cover, decomposition rate constant (k), and soil C and N storage. Also, leaf litter C, N, and P inputs from legumes correlated negatively and significantly with the decomposition rate constant k.
Tab. 4 - Spearman’s correlation between plot-level variables, and the variables measured in the legume (leg) and the non-legume (non-leg) functional groups. (*): p<0.05; (†): p<0.10.
Functional group level |
Basal area (BA) |
Litterfall | Leaf litter |
Leaf C | Leaf N | Leaf P | Canopy cover |
Forest floor |
k constant |
Soil C | Soil N |
---|---|---|---|---|---|---|---|---|---|---|---|
BA leg | 0.609* | 0.482 | 0.591† | 0.636 * | 0.673* | 0.164 | -0.100 | -0.327 | -0.473 | -0.400 | -0.227 |
BA non-leg | 0.345 | -0.109 | -0.236 | -0.227 | -0.409 | -0.009 | 0.264 | 0.245 | 0.373 | 0.455 | 0.355 |
Leaf litter leg | 0.245 | 0.445 | 0.718* | 0.718* | 0.809* | 0.264 | -0.018 | -0.209 | -0.609* | -0.345 | -0.282 |
Leaf litter non-leg | 0.118 | 0.245 | -0.09 | -0.127 | -0.218 | 0.282 | 0.518* | 0.255 | 0.273 | 0.345 | 0.327 |
C leg | 0.245 | 0.445 | 0.718* | 0.718* | 0.809* | 0.264 | -0.018 | -0.209 | -0.609* | -0.345 | -0.282 |
C non-leg | 0.273 | 0.509 | 0.264 | 0.273 | 0.164 | 0.527† | 0.627* | 0.336 | 0.036 | 0.164 | 0.173 |
N leg | 0.245 | 0.445 | 0.718* | 0.718* | 0.809* | 0.264 | -0.018 | -0.209 | -0.609* | -0.345 | -0.282 |
N non-leg | 0.336 | 0.645* | 0.373 | 0.310 | 0.255 | 0.609* | 0.682* | 0.409 | -0.009 | 0.318 | 0.327 |
P leg | -0.009 | 0.345 | 0.591† | 0.555† | 0.718* | 0.373 | -0.109 | -0.291 | -0.664* | -0.391 | -0.382 |
P non-leg | 0.436 | 0.600 | 0.400 | 0.345 | 0.264 | 0.791* | 0.609* | 0.318 | -0.036 | 0.391 | 0.418 |
Discussion
The studied SDTF is highly productive compared to other neotropical dry forests ([8], [34]). The mean annual precipitation and the way it is distributed along the year in the study region favor primary production at the site, more so than in other dry forests, such as those located in the Caatinga biome ([40]). Litter production, which can constitute up to 50% of dry forest primary production, is tightly linked to seasonal water availability in dry forests ([7], [41]). The inter-plot variability in canopy cover and leaf litter production observed in the study site increased between autumn and winter. This result may be explained by the natural changes in resource availability that occur across seasons, which shape forest structure and functional group composition. In our study site, most of the primary production was returned via leaf litter over the three winter months, when tree growth is limited. This result reveals the synchronism incorporated by the different functional groups in the study site, likely to minimize drought-related damage in trees. Similar results were observed in other tropical dry forests with different dry season intensities ([3], [41]).
The occurrence of a nearly 5-month long dry season in the study region implies the prevalence of functional groups adapted to water scarcity during forest succession ([3]). The most abundant and dominant tree species in the site have a set of functional traits that reinforce this prevalence, including high wood density (> 0.6 g cm-3) and leaf senescence during the dry season ([48], [21], [30]). Legume trees had twice the average basal area attained by non-legume trees in the plots. Moreover, the ability to engage in symbiotic interactions with N2-fixing bacteria, as is the case for 50% of the legumes identified on the site, suggests a competitive advantage of legume trees over non-legumes. The legume species, which were dominant in the larger diameter class, were probably the first functional group to occupy the area following the disturbances that occurred more than 50 years ago in the fragment ([21]). Non-legume trees, on the other hand, were abundant and dominant in the smaller diameter class, as well as among regenerating trees in the understory (Neves NM, unpublished data). These results suggest that the occupation of non-legume species throughout forest succession is facilitated by changes in the forest’s microclimatic and soil conditions. Furthermore, the prevalence of non-legumes in the smaller diameter class could affect forest canopy cover, as suggested by the positive and significant correlation observed between annual canopy cover and non-legume contributions to leaf litter, C, N, and P.
Overall, the leaf litter from legumes displayed higher levels of C, N, and P than the leaf litter from non-legumes. We presume that the small differences in N and P contents found between the two functional groups were caused by the mix of N2-fixing and non-N2-fixing trees in the legume functional group. Furthermore, the presence of N2-fixing trees contributes to an increase in C, N, and P fluxes among the legume group ([35], [38], [15]). Slightly higher levels of carbon in legume tree leaves may be related to the presence of secondary compounds widely abundant in legumes ([36]). The higher P content found in the legume leaf litter, compared to non-legumes during the spring, may be explained by the role of P in the N2-fixation process during regrowth following the dry season ([35], [15]). The higher N content found among the legume leaf litter during the driest months may be explained by the dominance of deciduous legume trees and the abundance of N2-fixing species in this functional group. The small amounts of leaf litter produced, regardless of legume dominance, may be due to the small sizes of leaves that dominate the overstory, which often belong to Pseudopiptadenia contorta, Parapiptadenia pterosperma, Dalbergia nigra, and Peltophorum dubium trees. Therefore, the N inputs from legume trees could help to reduce fertilization costs during SDTF restoration. The total amounts of N returned to the soil via leaf litter after half a century of secondary succession could reach 4100 kg ha-1, assuming constant annual N inputs of 83 kg ha-1. Future studies could be carried out to assess the contribution of biological N2-fixation to sustain the primary productivity of this forest fragment.
The forest floor functions as a reserve of nutrients that are slowly released into the soil by the action of water and organisms ([39], [16], [32]). The mean annual decomposition constant values observed in our study were lower than those estimated for tropical dry forests (1.2-1.7 - [2]), although it ranged between 0.8 and 2.3 in the plots. These results suggest that, other than water seasonality, other factors influence litter decomposition, such as quality of the litter material and decomposers ([7], [26]). Litter decomposition is likely influenced by the mix of species and functional groups in the plots. Moreover, the higher N inputs from the legume leaf litter during the winter could affect decomposition at the beginning of the growing season and, consequently, nutrient availability for other plants ([16], [49]). However, N-rich leaf litter may decrease decomposition in the long term, since N inhibits oxidative enzymes involved in lignin degradation ([1], [20], [16]). Moreover, the phenols, greases, lignin, cellulose, and hemicellulose present in the plant tissue change according to the proportion of species and functional groups in the litter mixture, affecting litter decomposition ([29], [18], [20]). Also, these secondary compounds, which often show allelopathic effects, are common among legumes and other drought-adapted families ([1]). Our results also suggested that legume leaf litter, C, N, and P inputs may have potential effects on the decomposition constant k. Increases in leaf litter and nutrient inputs from legume trees tended to decrease the value of k and, consequently, forest floor decomposition.
Conclusion
We showed that litter dynamics and nutrient inputs in a secondary seasonally dry tropical forest are affected by the season and the dominance of different functional groups. The substantially higher litterfall production during the two driest months of observation suggests phenological synchronicity among different functional groups. Legume trees, which attained twice the basal area attained by non-legumes, dominated among the largest diameter class. Legume trees deposited twice the amount of N deposited by non-legumes during the winter. Although legumes contributed higher quantities of leaf litter, C, and P mainly during the winter, the differences between legumes and non-legumes were statistically indistinguishable. We also found that, compared to non-legumes, the variables measured among the legume functional group correlated better with the plot-level variables. For example, legume basal area correlated positively with plot-level basal area, leaf litter, and leaf litter C and N contents. At the same time, non-legume basal area showed no significant correlation with any plot-level variable. Our results indicate potential effects of leaf litter and nutrient inputs from the legume functional group on the decomposition constant and, consequently, on the time of forest floor decomposition. Finally, we would encourage further studies to assess the role of different functional groups in litter dynamics and nutrient cycling in seasonally dry tropical forests.
Author contributions
RRP and NMN conceived the idea and methodology of the study; NMN, EAA, and RGG collected the data in the field and performed laboratory analysis; NMN, RRP, and EAA analyzed statistically the data; RRP and NMN wrote and reviewed the manuscript; SHK and KMPA supervised the study and contributed to manuscript. All the authors approved the publication. The authors declare no interest conflict.
Acknowledgements
We would like to thank the Fundação de Amparo à Pesquisa e Inovação do Espírito Santo (grants 71416382/2016), the Conselho Nacional de Desenvolvimento Científico e Tecnológico (Finance code: 300369/2016-6), and the Coordenação de Aperfeiçoamento de Pessoal de Nível Superior (Finance code: 300369/2016-6) for the financial support. We are also grateful to Laboratório de Recursos Hídricos e Solos of DCFM/UFES, Laboratório de Química da Madeira of DCFM/UFES and Laboratório de Isótopos Estáveis of CENA/USP for their technical support. We would like to thank the Instituto Federal de Ciência e Tecnologia do Espírito Santo, Campus de Alegre, for the available study area. We thank our colleague Luciana Lorenzoni Paschoa for providing fundamental scientific data for this research. We are also grateful to the anonymous reviewers whose comments helped to improve to the manuscript.
References
CrossRef | Gscholar
Gscholar
CrossRef | Gscholar
Online | Gscholar
Gscholar
CrossRef | Gscholar
Gscholar
Gscholar
CrossRef | Gscholar
CrossRef | Gscholar
Gscholar
CrossRef | Gscholar
CrossRef | Gscholar
Gscholar
Gscholar
CrossRef | Gscholar
Authors’ Info
Authors’ Affiliation
Ranieri Ribeiro Paula 0000-0002-2520-1779
Eduardo Alves Araujo 0000-0003-2300-1332
Gomes Gorsani Rodrigo
Sustanis Horn Kunz 0000-0001-6937-7787
UFES, Departamento de Ciências Florestais e da Madeira, Av. Governador Carlos Lindemberg, 316, Jerônimo Monteiro-ES, CEP 29.550-000 (Brazil)
UFES, Programa de Pós-Graduação em Produção Vegetal, Alto Universitário, s/n, Alegre-ES, CEP 29.500-000 (Brazil)
Instituto Nacional da Mata Atlntica - INMA-MCTI, Avenida José Ruschi, km 136, Santa Teresa, Espírito Santo, CEP 29650-000 (Brazil)
Laboratório de Ecologia e Evolução de Plantas - Programa de pós-graduação em Botnica, Universidade Federal de Viçosa, CEP 36570-900, Viçosa, Minas Gerais (Brazil)
Instituto Federal de Educação, Ciência e Tecnologia do Espírito Santo - Ifes, Campus de Alegre, Rod. 482, km 47, 29520-000, Alegre, ES (Brazil)
Corresponding author
Paper Info
Citation
Neves NM, Paula RR, Araujo EA, Gorsani Rodrigo G, Abreu KMP, Kunz SH (2022). Contribution of legume and non-legume trees to litter dynamics and C-N-P inputs in a secondary seasonally dry tropical forest. iForest 15: 8-15. - doi: 10.3832/ifor3442-014
Academic Editor
Maurizio Ventura
Paper history
Received: Apr 08, 2020
Accepted: Oct 31, 2021
First online: Jan 13, 2022
Publication Date: Feb 28, 2022
Publication Time: 2.47 months
Copyright Information
© SISEF - The Italian Society of Silviculture and Forest Ecology 2022
Open Access
This article is distributed under the terms of the Creative Commons Attribution-Non Commercial 4.0 International (https://creativecommons.org/licenses/by-nc/4.0/), which permits unrestricted use, distribution, and reproduction in any medium, provided you give appropriate credit to the original author(s) and the source, provide a link to the Creative Commons license, and indicate if changes were made.
Web Metrics
Breakdown by View Type
Article Usage
Total Article Views: 23080
(from publication date up to now)
Breakdown by View Type
HTML Page Views: 20434
Abstract Page Views: 1247
PDF Downloads: 1157
Citation/Reference Downloads: 4
XML Downloads: 238
Web Metrics
Days since publication: 926
Overall contacts: 23080
Avg. contacts per week: 174.47
Article Citations
Article citations are based on data periodically collected from the Clarivate Web of Science web site
(last update: Feb 2023)
(No citations were found up to date. Please come back later)
Publication Metrics
by Dimensions ©
Articles citing this article
List of the papers citing this article based on CrossRef Cited-by.
Related Contents
iForest Similar Articles
Research Articles
Effects of stand age on litter quality, decomposition rate and nutrient release of Kazdagi fir (Abies nordmanniana subsp. equi-trojani)
vol. 13, pp. 396-403 (online: 03 September 2020)
Research Articles
Assessing the relative role of climate on litterfall in Mediterranean cork oak forests
vol. 11, pp. 786-793 (online: 14 December 2018)
Research Articles
Carbon and nutrient contents in the miscellaneous fraction of litterfall under different thinning intensities in a semiarid Pinus halepensis afforestation
vol. 12, pp. 375-382 (online: 12 July 2019)
Research Articles
Effects of arbuscular mycorrhizal fungi on microbial activity and nutrient release are sensitive to acid deposition during litter decomposition in a subtropical Cinnamomum camphora forest
vol. 16, pp. 314-324 (online: 13 November 2023)
Research Articles
The estimation of canopy attributes from digital cover photography by two different image analysis methods
vol. 7, pp. 255-259 (online: 26 March 2014)
Research Articles
Nursery fertilization affected field performance and nutrient resorption of Populus tomentosa Carr. ploidy levels
vol. 15, pp. 16-23 (online: 24 January 2022)
Short Communications
Estimation of canopy attributes of wild cacao trees using digital cover photography and machine learning algorithms
vol. 14, pp. 517-521 (online: 17 November 2021)
Research Articles
Modifying harvesting time as a tool to reduce nutrient export by timber extraction: a case study in planted teak (Tectona grandis L.f.) forests in Costa Rica
vol. 9, pp. 729-735 (online: 03 June 2016)
Short Communications
Variation in soil carbon stock and nutrient content in sand dunes after afforestation by Prosopis juliflora in the Khuzestan province (Iran)
vol. 10, pp. 585-589 (online: 08 May 2017)
Research Articles
Nutrient uptake, allocation and biochemical changes in two Chinese fir cuttings under heterogeneous phosphorus supply
vol. 11, pp. 411-417 (online: 05 June 2018)
iForest Database Search
Search By Author
Search By Keyword
Google Scholar Search
Citing Articles
Search By Author
Search By Keywords
PubMed Search
Search By Author
Search By Keyword