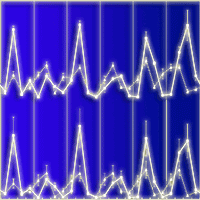
Assessing the relative role of climate on litterfall in Mediterranean cork oak forests
iForest - Biogeosciences and Forestry, Volume 11, Issue 6, Pages 786-793 (2018)
doi: https://doi.org/10.3832/ifor2825-011
Published: Dec 14, 2018 - Copyright © 2018 SISEF
Research Articles
Abstract
Litterfall plays a key role in the dynamic of forest ecosystems, ultimately determining forest productivity and carbon and nutrient cycling. Increasing our understanding on the role of structural and environmental factors controlling litterfall amount and seasonality is of paramount importance for modelling and estimating soil carbon sequestration and nutrient cycling under climate change scenarios. However, the effect of climatic conditions on litterfall has been scarcely studied, especially in Mediterranean ecosystems. Here, we used nine years of seasonally collected litterfall data in two contrasting Mediterranean cork oak forests to evaluate the effect of climatic variables on leaf fall and litterfall. First, we isolated the litterfall seasonal trend and the between-sites differences in production by using linear mixed models. Then, we evaluated the effect of climatic variables and whether this effect was site-specific. We found a consistent litterfall seasonal pattern, mainly determined by leaf shedding (70% of litterfall). Leaf fall mainly occurs in spring with a second but much smaller peak in autumn some years. Mean temperature, precipitation and mean wind speed strongly influenced litterfall, but this effect was site-specific. In the forest site located at higher latitude and altitude, leaf fall increased linearly with temperature and showed a positive quadratic response to precipitation. In the water-limited site, leaf fall was reduced as temperature increased and did not respond to precipitation. These results have implications for modelling and predicting soil carbon sequestration, nutrient cycling, and the forest ecosystem productivity. Specifically, carbon and nutrient cycling models can be improved by incorporating idiosyncratic forest sites responses to climatic variability.
Keywords
Climate, Leaf Fall, Litterfall, Modelling, Plant-soil Interactions, Quercus suber, Seasonality
Introduction
In forest ecosystems, litterfall is the largest source of aboveground organic material input to soil ([6]). It constitutes a key process in the dynamic of forest ecosystems determining the transfer of energy and nutrients ([44], [23]). As nutrient availability ultimately influences primary production, the amount, seasonality and the rate of decomposition of litterfall have profound effects on the productivity and the energy flow of forest ecosystems ([15], [13]). Besides maintaining soil fertility, litterfall plays other important roles in forest ecosystems, such as buffering changes in soil water content and temperature, reducing erosion, and increasing biodiversity ([47]). Soil respiration and soil organic content are also indirectly regulated by litterfall ([22]). Therefore, litterfall is of paramount importance for modelling and estimating soil carbon sequestration and nutrient cycling in forest ecosystems ([41], [32]). In this context, increasing our understanding of the role of structural and environmental factors on controlling litterfall amount and seasonality would help to improve models predictions ([32]), but also to guide forest management.
The amount of litterfall is related to environmental and structural properties, such as stand age, basal area, or canopy cover ([6], [44]). Nonetheless, several studies point to the pivotal role of climatic variables, such as temperature, radiation, or storms on litterfall amount and seasonality ([11], [3], [50], [8], [33]). Litterfall is influenced by phenology, evapotranspiration and photosynthesis ([39], [6], [50]). These mechanisms are ultimately affected by climatic conditions. Thus, climate change may have significant effects on litterfall. For example, climate warming is causing serious phenological changes in plants ([18]) that might induce changes in litterfall seasonality affecting soil fertility and the net primary production of forest ecosystems. A mechanistic understanding of the relationship between climatic conditions and litterfall is essential to accurately predict forest ecosystem productivity under climate change scenarios. This is especially true for those ecosystems in which water and nutrients are limiting.
The Mediterranean region has been identified as a primary hotspot particularly vulnerable to the impacts of climate change ([21]). Scenarios for climate change predict increasing temperatures and decreasing precipitation for this area ([14]). The productivity of evergreen Mediterranean ecosystems depends on intra- and inter-annual climatic fluctuations ([19]). The impact of environmental stress on plant growth has inspired a vast amount of publications, however the effect of environmental conditions on litterfall has been scarcely studied ([40]). Most of this research has been focused on reporting litterfall seasonality and nutrient return to the soil ([5], [43], [9], [2]). In addition, studies aimed at disentangling the role of climate on litterfall reported contradictory results. Liu et al. ([33]) showed an increase in litterfall of Mediterranean tree species with decreasing precipitation in a rainfall-manipulation experiment, whereas Caritat et al. ([11]) and Andivia et al. ([3]) reported a positive relationship between precipitation and litterfall in Mediterranean evergreen oaks. Evaluating the relationship between litterfall and climatic conditions is challenging because of the large spatial, inter-annual and seasonal variability of both, litterfall and climatic conditions. Indeed, some studies have suggested that this evaluation should be addressed using within-site sampling ([35]). In addition, the attribution of litterfall trends to climatic variability is troublesome, and the effect of climate should be assessed after isolating the seasonality of litterfall patterns.
Cork oak is a sclerophyllous evergreen oak and one of the most important forest tree species of the Mediterranean basin. Cork oak woodlands support high levels of biodiversity and ecosystem services, and they represent a model of sustainable ecosystem management with co-existing human activities, such as cork extraction and natural resource conservation. This has led to its inclusion in the Annex I of the European Union Habitats Directive (92/43/CEE). In this study, we used nine years of seasonally collected litterfall data in two contrasting cork oak (Quercus suber L.) forests to evaluate the effect of climatic variables on litterfall and leaf fall. By using a combination of linear mixed models we first isolated the seasonal pattern of litterfall, and the idiosyncratic site effect attributable to between-sites differences in forest structure and litterfall production. Then, we evaluated the effect of climatic variables and whether this effect was site-specific.
Materials and methods
Study sites
The study sites were located in two contrasting forest areas, Hinojos and Montseny. These differ mainly in forest structure (tree density and basal area) and climate (Fig. 1) as a consequence of different management regimes, and its geographical distance. Thus, Hinojos is a dehesa system in the south of Spain, while Montseny is a natural forest in the north of the Mediterranean biome. The main use of both forests is cork extraction.
Fig. 1 - Locations of the study sites (Hinojos and Montseny) in the Iberian Peninsula and their climate diagram during the study period. The Mediterranean ecoregion is depicted in dark red.
The Montseny site is located in a Mediterranean cork oak forest in northeastern Spain (Barcelona, Catalonia), in a flat area with an altitude of 780 m a.s.l. (41° 44′ N, 02° 23′ W) and with a tree density of 1082 trees ha-1. Cork oak is the dominant tree species, with a density of 700 trees ha-1 and a basal area of 49.5 m2 ha-1, while holm oak (Quercus ilex L subsp. ilex) appears as secondary tree species (382 trees ha-1 and 3.2 m2 ha-1). The understory community is mainly composed of Arbutus unedo L., Crataegus monogyna Jacp., Erica arborea L. and Cistus salviifolius L. The climate is Mediterranean subhumid with a mean annual precipitation of 895 mm, a mean annual temperature of 11.3 °C, a summer drought period of two months, and little interannual variability in temperature and precipitation (data from Viladrau weather station located 12 km away from the study plot - Fig. 1). The soil is classified as Eutric Leptosol ([26]), a stony and acidic soil with formation of a cambic horizon with moderate illuviation.
The Hinojos site is located in a Mediterranean cork oak woodland in southwestern Spain (Huelva, Andalusia), in a flat area with an altitude of 100 m a.s.l. (37° 19′ N, 06° 25′ W) and with a tree density of 100 trees ha-1. Cork oak is the dominant tree species, with a density of 84 trees ha-1 and a basal area of 7.2 m2 ha-1, while holm oak appears as secondary tree species (16 trees ha-1 and 0.9 m2 ha-1). The understory community is mainly composed of Pistacia lentiscus L., Daphne gnidium L., Chamaerops humilis L., Halimium halimifolium L., and Cistus spp. The climate is typical Mediterranean with a mean annual precipitation of 579 mm, a mean annual temperature of 18.9 °C, a summer drought period of five months, and high interannual variability in temperature and precipitation (data from Pilas “Medina Garvey” weather station located 11 km away from the study plot - Fig. 1). The soil is classified as Haplic Regosol over a Stagnic Regosol ([26]).
Litterfall sampling
Litterfall was collected using the trapping method ([43], [11], [2]) at 6 and 12 sampling points in Montseny and Hinojos, respectively. In Montseny, the six 0.25 m2 circular traps were randomly placed in a 0.04 ha plot. Because Montseny site is located in a dense forest (1082 trees ha-1) sampling points were all beneath tree canopies. The diameter at breast height (DBH) of cork oaks in the site ranged between 7 and 19 cm. In Hinojos sampling points were established beneath the canopy of 12 randomly selected trees in a study area of 2 ha. The DBH of the sampled trees ranged between 22 and 35 cm and the mean DBH was 27.7 cm, representing the central diameter class of all trees in the plot. To avoid any effect of orientation, four 0.16 m2 circular traps were placed at each cardinal point of every sampled tree at a distance corresponding to three quarters of the crown radius measured from the stem. Litterfall samples were then pooled by tree.
Samples were collected monthly for three years (2004-2006), and seasonally (winter, spring, summer and autumn) for six more years (2007-2012). Once collected, litterfall samples were oven-dried at 65 °C for 48 h, separated into cork oak leaves, cork oak twigs, acorns (if present) and others (mainly flowers and other plant remains), and finally weighed (± 0.01 g). Litterfall and leaf fall data were expressed as g m-2, by dividing the dry weight of litter collected from each sampling point by the surface area of the litter traps. Acorns were not included as a part of litterfall. For seasonal analysis, monthly samples were pooled by season in order to analyze the whole sampled period (2004-2012).
Climatic variables
Climatic covariates were obtained from an automatic weather station at each site. Air temperature, precipitation, relative humidity, PAR radiation, and wind speed were registered every 15 minutes. Climatic covariates, including mean temperature, mean minimum temperature, mean maximum temperature, mean relative humidity, minimum relative humidity, precipitation, maximum precipitation in 24 hours, mean solar PAR radiation, mean wind speed and absolute wind speed, were calculated per season for each site.
Data analysis
To analyze the seasonality of litterfall (not including acorns) and leaf fall (main litterfall fraction) we used linear mixed models (LMM). Models were fitted to log-transformed leaf fall and litterfall data in a season (9 years, from 2004 to 2012) and in a monthly basis (3 years, from 2004 to 2006), respectively. Leaf fall and litterfall data was log-transformed to achieve normality and homocedasticity. In both cases, our experimental design resulted in temporary autocorrelation due to the fact that litterfall series results in non-independency among observations within the same sampling point (i.e., repeated measurements). For these reasons, we considered each sampling point as a random term in the model. We also tested different autoregressive correlation structures for leaf fall and litterfall measurements. To do this, we adjusted models by Restricted Maximum Likelihood (REML) using different orders for the temporary autocorrelation structure and selected the one with the lowest value of the Akaike’s Information Criterion adjusted for sample size (AICc).
Once the best random structure was selected, we identified the best-supported fixed effect structure by following a backward model selection procedure. First, we fitted the full model including site, year, season or month (depend on the set of data), and the interaction between them, and we compared it with a reduced model in which the triple interaction was dropt. Then, if necessary, we compared the selected model with models that ignored each pairwise interaction and all the main effects, respectively. If the difference in AICc between two models was ≤ 4, then the simpler model was selected ([51]). All alternative models were fitted by the Maximum Likelihood method (ML), whereas the parameters of the selected model were estimated by REML.
To analyze the effect of climatic variables on leaf fall and litterfall, we first fitted LMMs with the selected random structure (see above) but considering site and season as main fixed effect, respectively. Then, we extracted the Pearson’s residuals from the selected models. These residuals are values of leaf fall and litterfall, without the effect of seasonality and site. This procedure allowed to estimate the relative effect of climatic variables avoiding likely confounding effects due to: (i) the seasonality of both, litterfall production and climatic variables; and (ii) differences in the amount of litterfall due to site characteristics. Before fitting the model, we performed a Pearson’s correlation analysis for the initial set of 10 meteorological covariates (Tab. S1 in Supplementary material). In order to avoid multicollinearity, we included in the model those variables that showed a correlation coefficient (r) lower than 0.6. When two or more covariates showed r > 0.6, we selected only one of them. Selected covariates were mean temperature (mT), precipitation (P) and mean wind speed (mWS). In order to account for non-linear effects, we considered these covariates in the model as second degree polynomials. To evaluate whether the effect of climatic variables was site-specific, we introduced in the model the pairwise interaction between the factor site and the selected covariates. We evaluated if the parameters of the selected model were significantly different from zero by a t-test (p< 0.05). If the quadratic coefficient of a climatic covariate was not significant, then we simplified the model by considering only the linear term. Climatic covariates were standardized to allow comparisons across model-estimated parameters ([51]). Multicollinearity was evaluated in all models using the Variance Inflation Factor (VIF > 3 indicating multicollinearity). All statistical analyses were performed in R ver. 3.2.5 using the package “nlme” ([42]).
Results
Litterfall amount
Mean yearly litterfall (± SD) for the study period was 391.1 ± 147.2 g m-2, with overall greater production in Montseny than in Hinojos (429.3 ± 183.8 g m-2 and 353.0 ± 110.5 g m-2, respectively). Litterfall was only greater in Hinojos in three out of the nine study years (2005, 2007 and 2010 - Tab. 1). Yearly litterfall for both sites were not significantly correlated (p = 0.75). In fact, the years of maximum and minimum amount of litterfall differ between study sites (Tab. 1). In Montseny, litterfall maximum and minimum occurred in 2004 (678.7 ± 110.8 g m-2) and 2007 (174.9 ± 67.6 g m-2), respectively, whereas in Hinojos the greatest and lowest amount was found in 2007 (534.0 ± 113.7 g m-2) and 2006 (238.7 ± 93.1 g m-2), respectively. Leaves were the main component of litterfall accounting for 68% of the total litterfall amount followed by the fraction others (18%) and twigs (14%).
Tab. 1 - Yearly mean amounts (± standard deviation) for each litterfall fraction and location (g m-2).
Location | Year | Leaves | Twigs | Others | Total |
---|---|---|---|---|---|
Hinojos | 2004 | 275.1 ± 63.4 | 72.9 ± 48.4 | 68.3 ± 16.3 | 416.3 ± 71.6 |
2005 | 201.4 ± 66.8 | 17.3 ± 28.5 | 34.3 ± 18.6 | 252.9 ± 83.4 | |
2006 | 149.5 ± 46.8 | 48.1 ± 57.3 | 41.0 ± 23.4 | 238.7 ± 93.1 | |
2007 | 225.8 ± 69.0 | 175.3 ± 185.8 | 133.0 ± 49.7 | 534.0 ± 113.7 | |
2008 | 215.0 ± 53.4 | 21.2 ± 22.0 | 65.4 ± 20.3 | 301.6 ± 85.4 | |
2009 | 244.7 ± 61.3 | 0.1 ± 0.2 | 71.6 ± 18.2 | 316.4 ± 105.2 | |
2010 | 236.0 ± 43.4 | 62.1 ± 56.0 | 157.3 ± 82.7 | 455.5 ± 71.9 | |
2011 | 241.4 ± 49.9 | 37.4 ± 40.1 | 95.2 ± 70.8 | 374.0 ± 90.5 | |
2012 | 175.6 ± 49.0 | 50.9 ± 120.5 | 60.9 ± 25.9 | 287.3 ± 88.5 | |
Montseny | 2004 | 392.0 ± 79.5 | 206.9 ± 79.6 | 79.6 ± 20.2 | 678.7 ± 110.8 |
2005 | 210.2 ± 46.1 | 29.6 ± 34.5 | 41.0 ± 35.6 | 280.7 ± 72.5 | |
2006 | 340.7 ± 54.5 | 44.2 ± 36.4 | 72.8 ± 24.0 | 457.7 ± 66.5 | |
2007 | 147.5 ± 43.8 | 3.8 ± 4.0 | 23.6 ± 12.2 | 174.9 ± 67.6 | |
2008 | 354.0 ± 52.8 | 77.3 ± 109.6 | 43.5 ± 6.8 | 474.8 ± 73.2 | |
2009 | 176.3 ± 17.2 | 54.5 ± 33.9 | 28.6 ± 12.6 | 259.5 ± 75.4 | |
2010 | 331.6 ± 84.5 | 18.1 ± 11.9 | 104.1 ± 51.1 | 453.8 ± 110.6 | |
2011 | 480.0 ± 182.3 | 16.4 ± 16.3 | 63.2 ± 22.2 | 559.5 ± 299.3 | |
2012 | 458.8 ± 47.0 | 19.2 ± 21.5 | 46.3 ± 11.0 | 524.3 ± 74.1 |
Seasonality of litterfall and leaf fall
The first-order autoregressive structure was selected as the random term structure for the LMMs used to analyze leaf fall and litterfall in a seasonally and monthly basis (Tab. S2 in Supplementary material). This means that a given observation within the same sampling point shows a temporary autocorrelation with the previous one. In all cases, the best supported model for the structure of the fixed effects included the triple interaction between site, year, and season or month (depending on the set of data). When models including the triple interaction were compared with models without this but considering pairwise interactions between fixed effects, the increase in AICc was greater than at least 100 units (Tab. S3). In the selected models, VIF values were lower than 2 units indicating an acceptable degree of collinearity.
Leaf fall and litterfall showed a similar pattern both, at seasonal and monthly basis (Fig. 2, Fig. 3). Litterfall and leaf fall peaks mainly occurred in spring whereas the lowest values were found in winter, especially for leaf fall (Fig. 2). However, in some years in Montseny, litterfall and leaf fall showed a small peak in summer. Interestingly, a second litterfall and leaf fall peak occurred some years in autumn in both sites. The monthly analysis of litterfall data confirms these patterns. Litterfall peaks occurred earlier in Hinojos (between March and May) than in Montseny (between May and July - Fig. 3). The autumn peak was more frequent in Montseny than in Hinojos, and was mainly concentrated in October.
Fig. 2 - Seasonal pattern of litterfall (above) and leaf fall (below) for each study site over the study period. Error bars represent the standard error. Different years are separated by vertical lines. (Wi): winter; (Sp): spring; (Su): summer; (Au): autumn.
Fig. 3 - Monthly pattern of litterfall (above) and leaf fall (below) for each study site for the period 2004-2006. Error bars represent the standard error. Different years are separated by vertical lines. Months are numerically indicated in the horizontal axis.
Effect of climatic variables on litterfall and leaf fall
The pairwise interactions between site and the climatic covariates (mT, P and mWS) were included in the selected models for seasonal leaf fall and litterfall (Tab. 2, Tab. S4 in Supplementary material). The best supported model included the effect of precipitation as a quadratic term, and the effect of mean temperature and wind speed as linear terms (Tab. 2). Mean temperature and wind speed showed an opposite effect between sites (Fig. 4, Fig. S1). Seasonal leaf fall and litterfall increased linearly with mean temperature and wind speed in Montseny site, while remained constant or slightly decreased in Hinojos. Precipitation had a positive effect on seasonal leaf fall and litterfall production in Hinojos site. In Montseny site, however, the greatest amount of leaf fall and litterfall occurred at the extremes of the precipitation values (Fig. 4). Interestingly, leaf fall during the second annual litterfall peak (i.e., autumn) was positively correlated with autumnal mean temperature (r = 0.26, p< 0.001) but neither with precipitation or mean wind speed.
Tab. 2 - Mean values (± SE) for each estimated parameter in the selected models for evaluating the effect of climate covariates on seasonal leaf fall and litterfall. For all models, “Hinojos” is the reference level for the site factor. (mT): mean temperature; (P): precipitation; (mWS): mean wind speed; (*): p < 0.05; (**): p < 0.01; (***): p < 0.001.
Parameter | Leaf fall | Litterfall |
---|---|---|
Intercept [“Hinojos”] | 0.12 ± 0.04** | 0.13 ± 0.04** |
Site [“Montseny”] | 0.72 ± 0.11*** | 0.62 ± 0.11*** |
mT | -0.31 ± 0.06*** | -0.21 ± 0.06** |
mT 2 | - | - |
P | 1.55 ± 1.38 | 2.25 ± 1.43 |
P2 | -0.74 ± 1.22 | -2.19 ± 1.26 |
mWS | -0.06 ± 0.04 | -0.17 ± 0.04*** |
mWS 2 | - | - |
Site [“Montseny”] × mT | 1.28 ± 0.10*** | 1.03 ± 0.10*** |
Site [“Montseny”] × mT2 | - | - |
Site [“Montseny”] × P | -4.79 ± 2.25* | -6.82 ± 2.34** |
Site [“Montseny”] × P2 | 8.46 ± 2.10*** | 9.56 ± 2.18*** |
Site [“Montseny”] × mWS | 0.26 ± 0.09** | 0.32 ± 0.09*** |
Site [“Montseny”] × mWS 2 | - | - |
Fig. 4 - Model predictions and 95% CI for the effect of climatic covariates on seasonal leaf fall in the study sites (Montseny and Hinojos). Leaf fall for each covariate was predicted using a fixing mean value for the other two covariates.
Discussion
Our results show similar patterns in the seasonality of litterfall and leaf fall between contrasting sites in terms of forest structure and climate. Once seasonality was removed, mean temperature, precipitation and mean wind speed were identified as the main determinants of litterfall and leaf fall. Interestingly, the effect of climatic variables differed between study sites, indicating an idiosyncratic forest response to climatic variability.
The amount of litterfall in our study (3.9 Mg ha-1 year-1) was similar to those values reported in other studies in Mediterranean cork oak (3.9-5.1 Mg ha-1 year-1 - [12], [10], [43], [45]) and holm oak forests (3.8-6.9 Mg ha-1 year-1 - [9], [3], [8]). Litterfall was greater in Montseny than in Hinojos as might be expected by the greater basal area and wetter climatic conditions in Montseny site ([6], [44]). Other factors, such as stand age or soil nutrient richness might also explain differences between sites. Independently of the study site, litterfall showed a strong seasonal pattern with a major peak in spring (March-June). These results are consistent to those reported for Mediterranean evergreen oaks ([5], [43], [9], [3], [8]). This seasonal pattern is tightly determined by leaf fall, which constitutes approximately 70% of the total litterfall amount. The main cause of leaf fall in spring is the renewal of the foliar cover after the bud flush ([17]). Sá et al. ([45]) reported that in average the leaf longevity of cork oak trees is 12 months, and the process of leaf shedding coincides in time with leaf emergence ([5], [9]). Leaf emergence in Mediterranean evergreen species tends to be concentrated in a single flush at the beginning of the growing season ([34]). This process requires high temperatures and good water status to maintain cell turgor and construct new xylem tissue ([49]). Thus, Mediterranean evergreen trees could take advantage of the most physiologically favorable period of the year to renew their crown prior to the onset of the dry summer ([9]).
Whereas leaf fall peak always occurs in spring in Hinojos, we found high summer leaf fall values during some years in Montseny (years 2004, 2009, 2011 - Fig. 2). This might be related either to: (i) a delay in leaf fall because of low spring temperatures in Montseny. Mean minimum temperatures in May 2004 were 2.1 °C (average value of 8.2 °C for the study period); (ii) drought-induced xylem cavitation that can accelerate branch and leaf fall in summer ([38], [36]). The summer of 2009 was especially dry with mean maximum temperatures and precipitation of 27.6 °C and 42.9 mm, respectively (average value of 25.9 °C and 180.6 mm, respectively); or (iii) unusual summer storms, such as in 2011 with more than 200 mm of precipitation between June and July. When leaf fall was analyzed on a monthly basis, we observed that leaf fall peaks also show a delay between sites, occurring earlier in Hinojos than in Montseny (Fig. 3). This difference can be due to an earlier spring phenology in Hinojos as a consequence of the higher temperatures due to the contrasting latitude and altitude between both sites ([6]). In some years, leaf fall showed a second but much smaller peak in autumn, which has been also observed in other Mediterranean forests ([9]). This second peak may occur because of a second sprouting after summer drought supported by favorable environmental conditions (temperature, precipitation) in early autumn ([9], [11]). In fact, we found a positive correlation between autumnal leaf fall and autumnal temperatures. The strong seasonality of litterfall has important implications for other processes at ecosystem scale, such as soil respiration and nutrient cycling ([39], [15], [1]). In general, Mediterranean plants reduce nutrient losses through litterfall by the translocation of nutrients from senescent leaves to new leaves before leaf shedding ([17], [39]). As a consequence, litterfall shows higher C:N ratios leading to lower decomposition rates ([46]). This strategy may enhance the ecosystem’s capacity to retain nutrients in the upper part of the forest soil, ensuring nutrient availability to trees under favorable soil temperature and moisture ([39], [46]).
After accounting for seasonality, our results identify mean temperature, precipitation and mean wind speed as the most important climatic factors affecting litterfall and leaf fall. Curiously, the direction of this effect was site-specific suggesting an idiosyncratic control of climatic conditions over litterfall. This differential effect might be mediated by endogenous factors, such as forest structure or age, but also by contrasting environmental severity between sites (Fig. 1). On the one hand, wind speed increased litterfall in Montseny while slightly affecting litterfall in Hinojos, in spite of the fact that wind and storms are expected to trigger leaf and twig shedding ([27]). On the other hand, temperature has disparate effects on leaf fall in Montseny and Hinojos, respectively. In Montseny (northern Spain, 780 m a.s.l.), leaf fall increased with temperatures probably because of a higher leaf production and crown renewal. Overall, increasing temperatures have positive effects on forest productivity through the expansion of the growing period, enhancing meristem activity and photosynthesis ([29]). However, increasing temperatures might also amplify drought severity in areas with water constraints ([31]). In fact, the effect of temperature on leaf fall in Hinojos (southern Spain) was negative. Under water scarcity conditions, the production of new leaves may be reduced increasing leaf retention and longevity, which would consequently decrease litterfall ([24], [36]). Nonetheless, drought conditions can also increase leaf fall because of xylem cavitation ([38], [36]), by accumulating abscisic acid and ethylene hormones inducing senescence and abscission ([20], [50]), or due to a general reduction in leaf area as a conservative strategy to reduce transpiration, maintain hydraulic conductance and avoid cavitation risk ([30], [4]). Liu et al. ([33]) reported that litterfall was 10% higher in plots with reduced water availability compared to control plots in a rainfall-exclusion experiment under similar environmental conditions than in Montseny. In line with these results, we found greater litterfall and leaf fall with lower precipitation in Montseny. In addition, the high forest density in Montseny might exacerbate summer drought leading to crown defoliation in order to adjust transpiration to water availability ([30]). Contrastingly, litterfall and leaf fall were also greater with high precipitation, which might be related either to a greater leaf production during the spring or to a second litterfall peak in autumn ([11], [3]). On the contrary, litterfall and leaf fall were not related to precipitation in Hinojos, where precipitation was lower than 600 mm in seven of the nine study years. Under these conditions, temperatures are expected to exert a greater control on litterfall than precipitation through modifying evapotranspiration ([6]). In this regard, Erkan et al. ([16]) reported a non-significant relationship between litterfall and precipitation in pine forests but litterfall production increased significantly with aridity (as the ratio between temperature and precipitation).
Our results have practical implications for forest carbon cycle and productivity models. Most of these models use simplified algorithms to simulate litterfall process ([25], [48]), which might result in large uncertainties in soil respiration calculations ([50]). Our results showed that litterfall seasonality differed between years and between sites, but more importantly the effect of climatic variables on litterfall was site-specific. Thus, incorporating this source of variability in these models seems appropriate to increase the accuracy of model predictions under climate change scenarios. In addition, further studies should explore the effect of different climate change scenarios on the patterns of litterfall production. In this regard, increasing our understanding of the influence of previous-year temperatures and precipitation on litterfall patterns is of pivotal importance to increase the accuracy of model predictions. This is especially important since there is some controversy as to whether resource limitation or year-to-year variation in climatic conditions drive certain aspects of forest productivity, such as masting ([28], [37], [7]).
Conclusions
Litterfall in Mediterranean cork oak forests shows a consistent seasonal pattern regardless of differences in forest structure. This pattern is mainly determined by leaf fall seasonality, which contributes to 70% of the total litterfall. Leaf fall is concentrated in spring, matching with the renewal of the foliar cover after the bud flush. In addition, a second but much smaller peak was observed some years in autumn. Differences in seasonality between sites were confined to an earlier leaf fall in spring in the study site located at lower latitude.
Mean temperature, precipitation and mean wind speed were the most important climatic factors affecting litterfall and leaf fall. Curiously, the effect of climatic variables differed between study sites indicating an idiosyncratic forest site response that might be mediated by differences in forest characteristics and environmental severity. Specifically, temperature increased leaf fall in the forest site located at a higher latitude and altitude, while leaf fall response to precipitation followed a positive quadratic relationship. Contrastingly, under water scarcity conditions leaf fall was reduced with increasing temperatures and did not respond to precipitation.
Acknowledgments
This study was supported by Ministry of Science and Innovation of Spain, the National Agriculture Research Institute (INIA; ref: SUM2006-00026-00-00), and the Diputació de Girona, Spain. EA was supported by a postdoctoral grant founded by the Universidad Complutense in Madrid. We gratefully acknowledge the logistic support from the Montseny Natural Park, and the valuable help of numerous people during the sampling along the study period. We are also very grateful to Zoë Rohrer for the revision of the English language. The comments and suggestions of two anonymous reviewers contributed to improve previous version of this manuscript.
Author Contributions
All authors contributed to the study design and data collection. EA performed statistical analyses with inputs from JVP. EA wrote the manuscript with contributions from the rest of authors.
Conflicts of Interest
The authors declare no conflict of interest.
References
CrossRef | Gscholar
Gscholar
CrossRef | Gscholar
Gscholar
CrossRef | Gscholar
CrossRef | Gscholar
Gscholar
CrossRef | Gscholar
Gscholar
Authors’ Info
Authors’ Affiliation
Departamento de Biodiversidad, Ecología y Evolución, Universidad Complutense de Madrid (Spain)
Antonia Caritat
Lluís Vilar
LAGP-Flora i Vegetació, Institut de Medi Ambient, Universitat de Girona (Spain)
Reyes Alejano
Javier Vázquez-Piqué
Departamento de Ciencias Agroforestales, Escuela Técnica Superior de Ingeniería, Universidad de Huelva (Spain)
Corresponding author
Paper Info
Citation
Andivia E, Bou J, Fernández M, Caritat A, Alejano R, Vilar L, Vázquez-Piqué J (2018). Assessing the relative role of climate on litterfall in Mediterranean cork oak forests. iForest 11: 786-793. - doi: 10.3832/ifor2825-011
Academic Editor
Andrea Cutini
Paper history
Received: Apr 24, 2018
Accepted: Oct 15, 2018
First online: Dec 14, 2018
Publication Date: Dec 31, 2018
Publication Time: 2.00 months
Copyright Information
© SISEF - The Italian Society of Silviculture and Forest Ecology 2018
Open Access
This article is distributed under the terms of the Creative Commons Attribution-Non Commercial 4.0 International (https://creativecommons.org/licenses/by-nc/4.0/), which permits unrestricted use, distribution, and reproduction in any medium, provided you give appropriate credit to the original author(s) and the source, provide a link to the Creative Commons license, and indicate if changes were made.
Web Metrics
Breakdown by View Type
Article Usage
Total Article Views: 40826
(from publication date up to now)
Breakdown by View Type
HTML Page Views: 34930
Abstract Page Views: 2675
PDF Downloads: 2447
Citation/Reference Downloads: 18
XML Downloads: 756
Web Metrics
Days since publication: 2169
Overall contacts: 40826
Avg. contacts per week: 131.76
Article Citations
Article citations are based on data periodically collected from the Clarivate Web of Science web site
(last update: Feb 2023)
Total number of cites (since 2018): 6
Average cites per year: 1.00
Publication Metrics
by Dimensions ©
Articles citing this article
List of the papers citing this article based on CrossRef Cited-by.
Related Contents
iForest Similar Articles
Research Articles
Predicting phenology of European beech in forest habitats
vol. 11, pp. 41-47 (online: 09 January 2018)
Research Articles
Carbon storage in degraded cork oak (Quercus suber) forests on flat lowlands in Morocco
vol. 9, pp. 125-137 (online: 08 August 2015)
Research Articles
Adjustment of photosynthetic carbon assimilation to higher growth irradiance in three-year-old seedlings of two Tunisian provenances of Cork Oak (Quercus suber L.)
vol. 10, pp. 618-624 (online: 17 May 2017)
Research Articles
Is cork oak (Quercus suber L.) woodland loss driven by eucalyptus plantation? A case-study in southwestern Portugal
vol. 7, pp. 193-203 (online: 17 February 2014)
Research Articles
SimHyb: a simulation software for the study of the evolution of hybridizing populations. Application to Quercus ilex and Q. suber suggests hybridization could be underestimated
vol. 11, pp. 99-103 (online: 31 January 2018)
Research Articles
Combined pre-hardening and fall fertilization facilitates N storage and field performance of Pinus tabulaeformis seedlings
vol. 9, pp. 483-489 (online: 07 January 2016)
Research Articles
Delineation of seed collection zones based on environmental and genetic characteristics for Quercus suber L. in Sardinia, Italy
vol. 11, pp. 651-659 (online: 04 October 2018)
Research Articles
Leaf morphology of progenies in Q. suber, Q. ilex, and their hybrids using multivariate and geometric morphometric analysis
vol. 11, pp. 90-98 (online: 31 January 2018)
Research Articles
Carbon and nutrient contents in the miscellaneous fraction of litterfall under different thinning intensities in a semiarid Pinus halepensis afforestation
vol. 12, pp. 375-382 (online: 12 July 2019)
Research Articles
The cork oak in the Mountains of Palermo (Italy): ecological insights from the south-eastern edge of its distribution range
vol. 13, pp. 336-344 (online: 07 August 2020)
iForest Database Search
Search By Author
Search By Keyword
Google Scholar Search
Citing Articles
Search By Author
Search By Keywords
PubMed Search
Search By Author
Search By Keyword