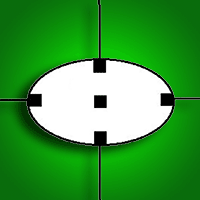
Influences of forest gaps on soil physico-chemical and biological properties in an oriental beech (Fagus orientalis L.) stand of Hyrcanian forest, north of Iran
iForest - Biogeosciences and Forestry, Volume 13, Issue 2, Pages 124-129 (2020)
doi: https://doi.org/10.3832/ifor3205-013
Published: Apr 07, 2020 - Copyright © 2020 SISEF
Research Articles
Abstract
Understanding the effects of silvicultural practices including single-tree selection on soil properties is essential for forest management in temperate broadleaved beech forests. Changes in physico-chemical and biological soil properties in 15 harvest-created gaps under single-tree selection and the adjacent closed canopies, with five replications for each, were studied 6 years after gap creation in an oriental beech (Fagus orientalis L.) stand of the Hyrcanian forest. Gaps were classified into three size classes: small (85-130 m2), medium (131-175 m2) and large (176-300 m2). Soil cores were collected at the center and at the edge of gaps, and under the adjacent closed canopy. Results indicated that gap size significantly affected soil texture and bulk density, whereas soil organic carbon (SOC), total nitrogen and pH showed a significant gradient from the center to the edge of gap independently form their size. SOC and total nitrogen at the center of gaps were also significantly lower than closed-canopy, in particular for the medium-gap; contrastingly, the bulk density with the highest mean value was found at the center of the large-gap. Gap size had no significant influence on soil microbial biomass. These results highlighted that similar conditions in terms of many soil properties were still present among gaps and adjacent closed-canopy stands six years after logging, though canopy openness triggered a reduction in carbon and nitrogen availability along with the related microbial activity at the center of gaps, independently from their size. Therefore, if aimed at preserving an uneven aged structure along with soil quality in temperate broadleaved deciduous forest as the oriental beech stands in the Hyrcanian region, single-tree selection practice for harvesting trees can be recommended as sustainable forest management type.
Keywords
Artificial Gap, Oriental Beech, Temperate Forests, Soil Properties
Introduction
Hyrcanian hardwood forests are characterized by a significant set of characteristics that make them ecologically and economically attractive. On the other hand, these forests suffered a lot of natural (e.g., wind) and artificial (e.g., logging) disturbance events, which frequently result in the formation of canopy gaps. Forest gaps are a critical factor of the disturbance regime in Hyrcanian temperate forests. Therefore, the understanding of the ecological processes following the gap formation are essential for sustainable forest management systems. Forest gaps are different sized spaces in the canopy and are due to the damage or death of one or more trees ([35]). Many environmental variations take place after the formation of a gap ([11]), among which the changes in the distribution of living and non-living resources, whose impact is crucial on both aboveground and belowground ecological processes in forests ([17]). Indeed, gaps influence the forest soil which is a vital part of the ecosystem functioning ([37]). According to Scharenbroch & Bockheim ([31]), different elements may influence soil processes including climate, organisms, physiography, parent material, time, and human activities. For example, the soil microbial biomass plays an important role in the nutrients cycle ([36]), resulting an important indicator of soil fertility for the ecological research and sustainable forest management ([26]). The canopy gap alters the microclimatic features of the soil and, consequently, the soil microbial biomass and respiration ([5]). Knowing about the amount of soil nutrients and its relationship to gap dynamics can afford valuable insights on forest ecosystem functioning. Previous studies have shown that soil physic-chemical and biological features were affected by gaps in different forest types. In old growth northern hardwood-hemlock forests (USA), Scharenbroch & Bockheim ([31]) reported that the value of exchangeable cations in the 0-25 cm mineral soil depth was lower in gaps than in closed canopy. In European silver fir stands (Abies alba Mill) in Italy, Muscolo et al. ([18]) reported the occurrence of chemical and microbiological variations in the soil of gaps. Other studies investigating soil respiration ([29], [23]), microclimate variables ([13]), microbial community ([32], [16]), microbial biomass ([4], [19]), enzyme activities ([37]) have also confirmed the influences of forest gaps on soil properties. The study of forest gaps dynamics is essential to understanding ecosystem function and predicting how forest ecosystems respond to disturbance and to forest management.
Oriental beech (Fagus orientalis L.) which belongs to the family Fagaceae is one of the most widespread broadleaved trees in mixed and pure stands of the Hyrcanian temperate forests. In these stands, different gap sizes have been generated by using different silvicultural systems, especially the single-tree selection harvesting method. Despite the importance of forest gaps in the development of Hyrcanian temperate forests, there is inadequate information of their effects on soil properties. This research aimed at determining the influences of forest gaps on soil features in broad-leaf, deciduous beech stand. Specific objectives of this study were: (i) to determine the effect of gap size on physico-chemical and biological properties of soil; (ii) to compare soil features among different positions (center, edge of gap and closed canopy); and (iii) to evaluate the correlation between gap size and soil properties.
Materials and methods
Site description
The study was carried out in an uneven-aged beech (Fagus orientalis Lipsky) forest located in the Hyrcanian region, northern Iran (36° 12′ N, 53° 24′ E), approximately from 1000 to 1200 m a.s.l. This site covers an area of about 40.4 ha on a 0-30% north-facing slope. The climate is humid, with a mean annual precipitation of 858 mm mostly concentrated in autumn, and a mean annual temperature of 10.5 °C. The dominant soil types are pseudogleyic and gley. Oriental beech trees occupy all forest layers, from overtopped to dominant ones. To a lesser extent, other tree species including alder (Alnus subcordata C.A. Mey), hornbeam (Carpinus betulus L.) and maple (Acer velutinum Boiss.) are commonly present. The broadleaved, multi-layered and uneven-aged beech stand originated from natural regeneration. The stand was managed under the single-tree selection silvicultural system in 2011. Under the current silvicultural system, different canopy gaps have generally been created within the stand. These harvest-generated gaps were randomly extended at the site. In total, 15 artificial gaps were sampled and categorized in three size classes of five replicates each: small (85-130 m2), medium (131-175 m2) and large (176-300 m2). The gap area was measured using the formula for an ellipse: A = (πLW) / 4, where L is the longest distance within the gap (m), and W is the largest distance perpendicular to L (m - Fig. 1). These distances were measured between stems of the trees at the border. All gaps were oriented in north exposition within forest (Fig. 1).
Fig. 1 - Sketch of location of the sampling points within gap and adjacent closed canopy. Capital letters indicate cardinal points.
Soil sampling
Soil samples were taken in October 2017, six years after gap creation. After removal of the litter layer, soil samples were collected by hand soil corer (8 cm diameter) from the shallower rooted soil layer up to 20 cm depth. The sampling protocol followed the scheme reported in Fig. 1. For each gap, 4 soil samples were collected at the cardinal points of the gap border and 4 at the adjacent closed canopy at a distance of 20 m from the gap (Fig. 1). The four gap-edge-cores were mixed to produce one composite sample (hereafter named gap-edge-core); the same was done for closed canopy cores (hereafter named close-canopy-core). One more core was collected at the center of the gap (hereafter named gap-center-core), so that soil samples totaled three per gap. Each composite soil sample was air dried and sieved to 2 mm mesh size, and any living plant material was removed manually from the sieved soil to accomplish physico-chemical analysis. A subsample of each soil was stored at 4 °C.
Physico-chemical analysis of soil
Physico-chemical properties of soil were analyzed based on Page ([22]). Soil pH was determined using a pH meter after shaking the soil:water (1:1, w/v) suspension for 1 hour. Bulk density (BD, g cm-3) at air-dried moisture content was calculated by clod method; soil bulk density was measured by calculating soil mass and volume using paraffin wax using the following steps. In clod method, soil bulk density is calculated through computing soil mass and volume by using paraffin wax and going through these steps: at first, the weight of a clod is determined and its volume is measured by covering it in paraffin wax heated up to 65-70 °C and the clod is plunged in the wax bath for roughly 24 h. The waxed clod is first weighed in the air and then weighed in the given volume of water where the water temperature is measured and the mass is checked yet again ([3]). Soil texture (sand, silt and clay) was determined using the Bouyoucos hydrometer method. In the case of hydrometer method, the methodology described in the work of Bouyoucos ([6]) and Papuga et al. ([24]) was applied. Soil moisture (%) was obtained by drying soil samples at 105 °C for 24 h. Water content was obtained by dividing the difference between wet and dry masses by the mass of the dry sample. Soil organic carbon (SOC, %) was assessed using Walkley & Black ([34]) method which involves oxidation of organic matter by K2Cr2O7 with H2SO4 heat of dilution and then titrated with ferrous ammonium sulfate. Total N content was determined by the Kjeldahl method ([7]). Phosphorus (P, mg kg-1) was obtained by the method of Olsen et al. ([21]). This method used a 2.5-g sample shaken with 50 ml 0.5 m NaHCO3, buffered at pH 8.5, for 30 minutes and filtering extracts through Whatman no. 42 filter paper. P concentration was determined by inductively coupled plasma atomic emission spectroscopy (ICP-AES); blank and standards were prepared in Olsen P extracting solution ([10]).
Soil microbial biomass C, N and P
Soil microbial biomass carbon (MBC), nitrogen (MBN) and phosphorous (MBP) were obtained using the chloroform fumigation-extraction method based on Brookes et al. ([7], [8]) using K2SO4 (0.5 M) and NaHCO3 (0.5 M) as extracting solution in MBC, MBN and MBP, respectively. Briefly, weighed portions of moist soil were put into a desiccator containing wet filter paper and alcohol-free liquid CHCl3. The desiccator was evacuated on a water pump until the CHCl3 had boiled vigorously for 5 min. The desiccator containing CHCl3 was kept at 25 °C until the fumigated soil was required for chemical analysis. Control, non-fumigated soil was treated similarly, except that the desiccator contained no CHCl3 and was not evacuated.
Microbial biomass C was measured by extracting the fumigated soil immediately following CHCl3 removal by shaking for 30 min with 0.5 M K2SO4 at a solution:soil ratio of 4:1. After filtration through a Whatman no. 42 filter paper, the filtrate was analyzed for organic C using dichromate digestion. Microbial biomass C was calculated as follows: MBC = Ec · (2.64), where Ec is the different between organic C extracted by 0.5 M K2SO4 from fumigate and non-fumigated soil ([33]) and also MBN was calculated from the difference between the amount of inorganic N extracted by 0.5 M K2SO4 from fresh soil fumigated with CHCl3 and the amount extracted from unfumigated soil by dividing by 0.54 ([2], [12]). MBP was calculated from the difference between the amount of inorganic P extracted by 0.5 M NaHCO3, (pH 8.5) from fresh soil fumigated with CHCl3 and the amount extracted from unfumigated soil by dividing by 0.4 ([7]).
Soil respiration
The CO2 emission from each soil sample was estimated after incubation for 3 days at 25 °C (55% water content) in a closed system. CO2 was trapped in a NaOH solution, which was then titrated with HCl ([2]).
Statistical analyses
To compare the three gap-size classes, 5 artificial gaps (replicates) per size class were established. Two-way ANOVA was performed to evaluate the effects of gap size and within-gap core position. Data were transformed where necessary to meet assumptions. One-way ANOVA followed by the least significant difference (LSD) test were performed among the three closed-canopy controls to exclude possible differences among them. To test the difference between the gaps and the closed-canopy, a second one-way ANOVA followed by the Dunnett’s test (bilateral alternative, P < 0.05) was applied to differences among the closed-canopy (reference mean) and both center and edge cores for each gap size.
Pearson’s correlation was performed to examine the relationships within physico-chemical or biological variables and the gap size, this latter simply scaled in three increasing levels. Significant levels were accepted at P<0.05 and P<0.01. All the analyses were performed with the software SPSS® version 20.0 (IBM, Armonk, NY, USA).
Results
Soil physico-chemical characteristics
Forest gaps significantly affected some properties of the soil (Tab. 1). For the physical characteristics, gap size affected the clay (P = 0.011 - Tab. 1) and sand (P = 0.018 - Tab. 1) proportion, with the medium size showing the lowest values for sand and the highest for clay (Tab. 2). Moreover, independently form the gap size, the sand percentage was significantly lower in the center than the gap-edge (position effect, P = 0.022 - Tab. 1) the opposite for the clay (position effect, P = 0.044 - Tab. 1). The gap size affected the bulk density as well (P = 0.008 - Tab. 1), increasing from the smaller to the larger size (Tab. 2). For the chemical properties, no significant differences occurred between the different gap sizes, whereas a significant gradient (within-gap-position effect) was observed for SOC (P = 0.034), total N (P = 0.028) and the pH (P = 0.048) with the SOC and total N increasing from the center to the edge, the opposite for the pH.
Tab. 1 - F and P values of ANOVA (GLM) for the effects of gap-size and within gap core position for gaps cores only on soil physico-chemical variables. (SOC): Soil organic carbon; (Total N): Total Nitrogen; (P): Phosphorus; (BD): Bulk Density.
Variable | Stats | Gap size | Within Gap Position |
Gap size × W-G Position |
---|---|---|---|---|
(df = 2) | (df = 1) | (df = 2) | ||
SOC (%) | F | 1.589 | 5.1 | 0.174 |
P | 0.227 | 0.034 | 0.841 | |
Total N (%) | F | 1.822 | 5.451 | 0.268 |
P | 0.183 | 0.028 | 0.767 | |
P (mg kg-1) | F | 1.902 | 0.157 | 0.441 |
P | 0.171 | 0.695 | 0.648 | |
Clay (%) | F | 5.41 | 4.519 | 0.249 |
P | 0.011 | 0.044 | 0.782 | |
Silt (%) | F | 2.741 | 0.371 | 0.177 |
P | 0.085 | 0.548 | 0.839 | |
Sand (%) | F | 4.804 | 5.98 | 0.724 |
P | 0.018 | 0.022 | 0.495 | |
pH | F | 0.591 | 4.275 | 0.64 |
P | 0.562 | 0.048 | 0.536 | |
BD (g m-3) | F | 5.934 | 2.617 | 0.686 |
P | 0.008 | 0.119 | 0.513 | |
Moisture content (%) | F | 0.325 | 0.051 | 2.335 |
P | 0.726 | 0.823 | 0.118 |
Tab. 2 - Physico-chemical variables for two within-gap position and three gap-size classes, and the adjacent closed canopy. Values are the mean (± standard deviation) of 5 replicates, 15 replicates for the closed-canopy only. The letters a, b, and c indicate significant differences between all positions (LSD, P<0.05); the letters x and y indicate significant differences between within-gap positions and closed canopy (Dunnet’s test, bilateral alternative, P<0.05). (SOC): Soil organic carbon; (Total N): Total Nitrogen; (P): Phosphorus; (BD): Bulk density.
Variable | Small | Medium | Large | Closed canopy |
|||
---|---|---|---|---|---|---|---|
gap-center | gap-edge | gap-center | gap-edge | gap-center | gap-edge | ||
SOC (%) | 5.32 ± 1.47 | 6.41 ± 1.56 b | 3.86 ± 1.42 ax | 5.52 ± 0.48 b | 4.81 ± 0.97 | 5.74 ± 2.06 b | 5.98 ± 1.36 y |
Total N (%) | 0.45 ± 0.13 | 0.55 ± 0.12 b | 0.33 ± 0.12 ax | 0.47 ± 0.04 | 0.43 ± 0.08 | 0.49 ± 0.18 b | 0.51 ± 0.12 y |
P (mg kg-1) | 5.76 ± 1.95 | 4.92 ± 0.67 | 4.95 ± 2.72 | 4.24 ± 0.47 | 6.00 ± 2.96 | 6.68 ± 1.85 | 5.34 ± 2.54 |
Clay (%) | 20.90 ± 4.92 | 15.50 ± 3.48 a | 25.10 ± 3.09 b | 22.80 ± 3.57 b | 19.10 ± 8.08 | 15.40 ± 4.57 a | 19.90 ± 5.83 |
Silt (%) | 10.50 ± 1.37 | 11.10 ± 2.99 a | 7.55 ± 2.08 b | 8.80 ± 3.61 | 9.92 ± 3.22 | 9.80 ± 1.16 | 10.20 ± 3.34 |
Sand (%) | 68.50 ± 3.64 b | 73.30 ± 1.91 a | 67.30 ± 2.12 b | 68.40 ± 2.77 b | 70.90 ± 5.27 | 74.80 ± 4.70 a | 69.70 ± 4.38 |
pH | 6.83 ± 0.21 | 6.72 ± 0.40 | 7.09 ± 0.27 a | 6.72 ± 0.23 b | 6.85 ± 0.26 | 6.76 ± 0.21 | 6.87 ± 0.27 |
BD (g m-3) | 1.28 ± 0.17 b | 1.27 ± 0.20 b | 1.53 ± 0.12 | 1.29 ± 0.23 b | 1.69 ± 0.32 ax | 1.54 ± 0.23 | 1.33 ± 0.18 y |
Moisture (%) | 43.70 ± 9.95 | 38.60 ± 9.39 | 32.80 ± 6.98 | 43.20 ± 4.93 | 41.40 ± 13.4 | 38.30 ± 4.02 | 34.90 ± 5.90 |
The closed-canopy did not show any significant difference with gaps for all properties, with the only exception of the lower SOC and total N concentrations for the medium sized and the higher BD for the large sized gaps, both measured at the center of the gap. Correlation highlighted the relationship among those variables affected by within-gap-position effect. Moreover, gap size correlated positively only with BD (Tab. S1 in Supplementary material).
Soil microbial biomass C, N and P
As shown in Tab. 3, forest gaps had no influence on the biological properties of the soil (P>0.05). MBP marginally missed the significance for the variance of within gap position (P = 0.061 - Tab. 3) with values at the center always lower than at the edge, significant only for the medium sized gap (Tab. 4); MBN/total N marginally missed the significance for the gap size × within gap position interaction (P = 0.087) with values at the gap center higher than those at the edge except for the medium sized gap. No differences occurred also between the three controls, so that they were pooled and treated as one. Although not significantly, there was a general trend for the gaps to have MBC, MBP, MBN concentrations and their ratios higher than the closed-canopy plots. MBC directly correlated with MBP and its own ratio to MBN and SOC. In the microbial biomass, the C:N ratio increased with decreasing N:P and N:total N ratios (Tab. S1, Supplementary material). Between physico-chemical and biological variables, it is worth to note the direct relationship between MBC and silt percentage. Nitrogen in microbial biomass (MBN) increased with increasing N and SOC concentrations into the soil, whereas an inverse relationship did occur with soil pH and BD (Tab. S1).
Tab. 3 - F and P values of ANOVA (GLM) for the effects of gap-size and within gap core position for gaps cores only on soil biological variables. (MBC): Microbial Biomass Carbon; (MBP): Microbial Biomass Phosphorus; (MBN): Microbial Biomass Nitrogen.
Variable | Stats | Gap size | Within Gap Position |
Gap size × W-G Position |
---|---|---|---|---|
(df = 2) | (df = 1) | (df = 2) | ||
MBC (mg kg-1) | F | 0.091 | 0.968 | 0.574 |
P | 0.914 | 0.335 | 0.571 | |
MBP (mg kg-1) | F | 0.176 | 3.853 | 0.912 |
P | 0.839 | 0.061 | 0.415 | |
MBN (mg kg-1) | F | 1.802 | 1.226 | 1.301 |
P | 0.189 | 0.281 | 0.292 | |
MBC/MBN | F | 2.364 | 0.341 | 0.583 |
P | 0.121 | 0.566 | 0.567 | |
MBC/MBP | F | 0.241 | 2.008 | 0.474 |
P | 0.787 | 0.169 | 0.628 | |
MBN/MBP | F | 1.012 | 0.418 | 0.858 |
P | 0.379 | 0.524 | 0.436 | |
MBC/SOC% | F | 0.366 | 0.235 | 0.067 |
P | 0.698 | 0.633 | 0.935 | |
MBN/Total N | F | 2.116 | 0.29 | 2.749 |
P | 0.146 | 0.596 | 0.087 | |
MBP/P | F | 0.045 | 2.436 | 1.164 |
P | 0.956 | 0.134 | 0.333 | |
Respiration (mg CO2-C g-1 soil d-1) |
F | 0.847 | 0.201 | 1.118 |
P | 0.441 | 0.658 | 0.343 |
Tab. 4 - Biological variables for two within-gap position and three gap-size classes, and the adjacent closed canopy. Values are the mean (± standard deviation) of 5 replicates, 15 replicates for the closed-canopy only. The letters a, b, and c indicate significant differences between all positions (LSD, P<0.05); the letters x and y indicate significant differences between within-gap positions and closed canopy (Dunnet’s test, bilateral alternative, P<0.05). (MBC): Microbial Biomass Carbon; (MBP): Microbial Biomass Phosphorus; (MBN): Microbial Biomass Nitrogen; (SOC): Soil Organic Carbon; (Total N): Total Nitrogen; (P): Phosphorus.
Variable | Small | Medium | Large | Closed canopy |
|||
---|---|---|---|---|---|---|---|
gap-center | gap-edge | gap-center | gap-edge | gap-center | gap-edge | ||
MBC (mg kg-1) | 405.60 ± 256.1 | 371.70 ± 169.6 | 294.50 ± 138.8 | 463.70 ± 271.8 | 370.30 ± 180.4 | 466.40 ± 237.6 | 295.50 ± 191.4 |
MBP (mg kg-1) | 5.20 ± 3.18 | 5.63 ± 2.33 | 3.87 ± 1.80 a | 8.85 ± 4.47 b | 5.21 ± 2.08 | 7.74 ± 5.47 | 1.94 ± 0.61 |
MBN (mg kg-1) | 102.90 ± 47.0 | 106.40 ± 81.9 | 51.70 ± 24.8 | 116.30 ± 39.1 | 63.20 ± 47.2 | 58.50 ± 38.4 | 83.90 ± 55.9 |
MBC/MBN | 2.87 ± 1.70 | 1.94 ± 0.98 | 6.69 ± 4.07 | 4.82 ± 4.84 | 4.71 ± 2.74 | 6.03 ± 4.16 | 1.80 ± 0.80 |
MBC/MBP | 110.70 ± 74.2 | 66.48 ± 21.6 | 90.30 ± 57.6 | 54.90 ± 25.5 | 83.60 ± 66.2 | 82.30 ± 44.7 | 77.70 ± 51.9 |
MBN/MBP | 44.10 ± 40.2 | 21.50 ± 20.2 | 18.60 ± 17.9 | 23.60 ± 21.5 | 17.70 ± 21.2 | 17.60 ± 22.1 | 26.30 ± 28.8 |
MBC/SOC | 72.30 ± 33.9 | 65.90 ± 37.8 | 79.80 ± 42.0 | 67.00 ± 40.3 | 59.80 ± 24.7 | 59.20 ± 27.4 | 49.40 ± 32.4 |
MBN/Total N | 260.90 ± 138.1 a | 135.10 ± 107.8 | 151.70 ± 44.3 | 239.70 ± 67.6 | 130.20 ± 105.4 | 109.50 ± 81.22 b | 168.90 ± 105.8 |
MBP/P | 0.90 ± 0.43 | 1.13 ± 0.36 | 0.63 ± 0.36 | 1.39 ± 0.38 | 0.96 ± 0.48 | 0.93 ± 0.89 | 0.93 ± 0.61 |
Respiration (mg CO2-C g-1 soil d-1) |
0.10 ± 0.06 | 0.10 ± 0.04 | 0.09 ± 0.05 | 0.13 ± 0.03 | 0.14 ± 0.03 | 0.11 ± 0.04 | 0.09 ± 0.05 |
Discussion
This work highlighted that changes in the size of gap six years after logging may affects soil physico-chemical characteristics rather than the microbial biomass and soil respiration. This outcome is particularly evident for the medium-sized gaps in this study (131-175 m2). Moreover, some of the soil chemical characteristics such as SOC and total N showed a significant decrease in the center of gaps, independently from their size. Studies on the correlation between canopy gap size and BD have provided inconsistent results; correlation was found positive in broad-leaved Pinus koraiensis forests ([38]) or absent in old-growth Tsuga canadiensis forests ([32]). The lack of correlation of BD with the textural component of the soil made this result hard to fathom, but soil compaction due to the seedling recruitment ([20]) cannot be ruled out.
Markedly higher soil moisture levels in the artificial gaps compared to adjacent closed-canopy were found for Danish Fagus sylvatica forests ([28]) and Turkish oriental beech, oak, and chestnut stands ([30]). This can be related to the joint effects of an increased rainfall and reduced transpiration by plant uptake ([15], [20]).
The openness of the canopy induced a significant decrease of soil pH at the edge of the gap, independently from its size, but no difference occurred in comparison to the closed canopy. Zhang & Zhao ([38]) found no significant difference for soil pH between canopy gaps and closed-canopy sites in broad-leaved Pinus koraiensis forests. Kooch et al. ([14]) reported a higher amount of pH for the large gaps in comparison to the medium gaps. Interestingly, the inverse pattern occurred for SOC and total N, resulting in a significative negative correlation with soil pH. Many factors could affect soil pH, such as salt base saturation, soil redox state, soil water content, and CO2 partial pressure in soils. The increase of soil respiration with the gap opening, though not observed in this study with the only exception for the large gaps, increases HCO32- concentration resulting in higher pH ([25]).
These findings are partially compatible with those of Ritter & Bjørnlund ([27]) who indicated that gap had no influence on the amount of organic material of the soil in Fagus sylvatica stands. In fact, in this study the canopy openness affected anyhow the amount of SOC and N available into the soil in the central area of the gap, particularly for the medium size gaps. The lack of significance for the smaller and larger gaps might be related to the small number of soil samples in comparison to the high soil heterogeneity. The lack of noticeable changes among gaps in this study could be also attributed to resistance of forests to small scale disturbances ([27]).
Regarding the soil biological variables, the slight changes observed among gaps, although not statistically significant, may be attributed to vegetation type ([26]), changes in the microclimatic properties of the soil ([5]), and gaps age. Microbial biomass changes according to soil temperature, moisture, and depth of soil ([26]). In addition to microclimate conditions, variations of soil biological characteristics may also be related to soil compaction and reduction in woody residuals ([16]). In fact, discrepancy in the quantity and quality of leaf and root litters can be an essential factor affecting the microbial biomass of the soil ([36]). The significant direct correlations observed between nitrogen concentration in microbial biomass (MBN) and N and SOC concentrations into the soil highlighted this essential factor, though independent form the size of gaps. In this study, microbial biomass markedly increased from closed-canopy to gaps (Tab. 4). Micro-environmental conditions that benefit microbial activity, including temperature of air and soil, solar radiation and soil moisture, have been found more favorable in gaps than in adjacent closed-canopy stands, so that gaps could have higher rates of decomposition than the closed forest ([32], [1]). Although soil respiration is a valuable indicator to study decomposition status, it is variable in nature and changes in response to moisture, available resources, and temperature ([5]). In this study, soil microbial biomass in terms of MBC, MBN, MBP and their ratios were higher in gaps than in closed canopy highlighting the higher nutrient concentrations in the gaps, though their values were lower at the center than at the edge. Moreover, the ratio of MBC to soil organic matter (MBC/SOC) is used to compare the quality of soil with different organic material concentrations ([9]). The higher MBC/SOC ratios observed in gaps and in particular at the center highlighted the higher availability of organic matter provided by the litter of herb-tree layer plants in this position. Likewise, MBN/total N and MBP/available P indicate the availability of N and P. A decrease in MBN with increasing gap size (Tab. 4) might be related to the influence of soil temperature and moisture on decreasing microbial biomass and decomposition of organic matter ([20]). According to our results, correlations observed between some of the physico-chemical and biological investigated variables highlighted the occurrence of a gradient from the edge to the center of the gap responsive to the openness of the canopy, and such gradient is independent from the size of gap.
Conclusions
This research examined the influence of artificial gaps created under single- tree selection in oriental beech stand on some soil properties and its comparison with undisturbed adjacent stands. According to results, it can be concluded that there are relative similar conditions in terms of many soil properties among gaps and adjacent closed-canopy stands six years after logging, though canopy openness triggered a reduction in carbon and nitrogen availability at the center of the gaps, independently from their size. Therefore, if aimed at preserving an uneven aged structure along with soil quality in temperate broadleaved deciduous forest as the oriental beech stands in the Hyrcanian region, single-tree selection practice for harvesting trees can be recommended as sustainable forest management type.
Acknowledgements
We would like to express our gratitude to Lorestan University, Lorestan, Iran to provide us with financial supports.
References
Gscholar
Gscholar
CrossRef | Gscholar
Gscholar
Authors’ Info
Authors’ Affiliation
Kambiz Abrari Vajari 0000-0002-7637-3984
Mohammad Feizian 0000-0002-0212-3712
Faculty of Agriculture and Natural Resource, Lorestan University, Khorramabad (Iran)
Department of Biotechnology and Life Science, University of Insubria, 21100 Varese (Italy)
Corresponding author
Paper Info
Citation
Amolikondori A, Abrari Vajari K, Feizian M, Di Iorio A (2020). Influences of forest gaps on soil physico-chemical and biological properties in an oriental beech (Fagus orientalis L.) stand of Hyrcanian forest, north of Iran. iForest 13: 124-129. - doi: 10.3832/ifor3205-013
Academic Editor
Giorgio Alberti
Paper history
Received: Jul 30, 2019
Accepted: Jan 25, 2020
First online: Apr 07, 2020
Publication Date: Apr 30, 2020
Publication Time: 2.43 months
Copyright Information
© SISEF - The Italian Society of Silviculture and Forest Ecology 2020
Open Access
This article is distributed under the terms of the Creative Commons Attribution-Non Commercial 4.0 International (https://creativecommons.org/licenses/by-nc/4.0/), which permits unrestricted use, distribution, and reproduction in any medium, provided you give appropriate credit to the original author(s) and the source, provide a link to the Creative Commons license, and indicate if changes were made.
Web Metrics
Breakdown by View Type
Article Usage
Total Article Views: 35793
(from publication date up to now)
Breakdown by View Type
HTML Page Views: 31686
Abstract Page Views: 1966
PDF Downloads: 1687
Citation/Reference Downloads: 4
XML Downloads: 450
Web Metrics
Days since publication: 1689
Overall contacts: 35793
Avg. contacts per week: 148.34
Article Citations
Article citations are based on data periodically collected from the Clarivate Web of Science web site
(last update: Feb 2023)
Total number of cites (since 2020): 6
Average cites per year: 1.50
Publication Metrics
by Dimensions ©
Articles citing this article
List of the papers citing this article based on CrossRef Cited-by.
Related Contents
iForest Similar Articles
Research Articles
Soil fauna communities and microbial activities response to litter and soil properties under degraded and restored forests of Hyrcania
vol. 14, pp. 490-498 (online: 11 November 2021)
Research Articles
Effects of altitudinal gradients on leaf area index, soil microbial biomass C and microbial activity in a temperate mixed forest ecosystem of Northwestern Turkey
vol. 10, pp. 334-340 (online: 15 December 2016)
Research Articles
Effects of tree species, stand age and land-use change on soil carbon and nitrogen stock rates in northwestern Turkey
vol. 9, pp. 165-170 (online: 18 June 2015)
Research Articles
Potential relationships of selected abiotic variables, chemical elements and stand characteristics with soil organic carbon in spruce and beech stands
vol. 14, pp. 320-328 (online: 09 July 2021)
Research Articles
Short-time effect of harvesting methods on soil respiration dynamics in a beech forest in southern Mediterranean Italy
vol. 10, pp. 645-651 (online: 20 June 2017)
Research Articles
Dynamics of humus forms and soil characteristics along a forest altitudinal gradient in Hyrcanian forest
vol. 14, pp. 26-33 (online: 10 January 2021)
Research Articles
Wood-soil interactions in soil bioengineering slope stabilization works
vol. 2, pp. 187-191 (online: 15 October 2009)
Research Articles
Spatial heterogeneity of soil respiration in a seasonal rainforest with complex terrain
vol. 6, pp. 65-72 (online: 07 February 2013)
Research Articles
Impact of deforestation on the soil physical and chemical attributes, and humic fraction of organic matter in dry environments in Brazil
vol. 15, pp. 465-475 (online: 18 November 2022)
Research Articles
Effect of size and surrounding forest vegetation on chemical properties of soil in forest gaps
vol. 8, pp. 67-72 (online: 04 June 2014)
iForest Database Search
Search By Author
Search By Keyword
Google Scholar Search
Citing Articles
Search By Author
Search By Keywords
PubMed Search
Search By Author
Search By Keyword