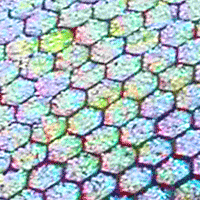
Size and age: intrinsic confounding factors affecting the responses to a water deficit in black spruce seedlings
iForest - Biogeosciences and Forestry, Volume 8, Issue 4, Pages 401-409 (2014)
doi: https://doi.org/10.3832/ifor1412-007
Published: Dec 09, 2014 - Copyright © 2014 SISEF
Research Articles
Abstract
The resistance to stress of seedlings during the initial phases after planting is fundamental for assuring fast establishment and long-term survival of artificial regeneration. Although needing less storage space and handling during their production and planting, small seedlings are considered to be less efficient in terms of water uptake and more sensitive to a water deficit than bigger seedlings. The responses to a water deficit produced by a suspension of irrigation for 14 days were assessed in black spruce (Picea mariana [Mill.] BSP) seedlings of different sizes, with height ranging between 13 and 71 cm. Seedlings growing in containers with cavity volumes of 25, 50, 110, 350 cm3 were tested. During the treatment, the seedlings attained Ψpd of between -1.71 and -2.28 MPa, indicating that a severe water stress was reached. Smaller seedlings exhibited similar or higher water potential and gas exchanges than bigger seedlings both during and after the treatment. Although root biomass was higher in bigger seedlings, the growth rates of roots were similar between seedling sizes and were not affected by the water stress. The initial hypothesis that small seedlings are more sensitive to water stress was rejected. The delayed stomatal closure and higher CO2 assimilation rate of smaller seedlings during the treatment could be attributed to a lower shoot:root ratio and greater ability of roots to sustain the evaporative needs of needles, which could attain higher performances in carbon assimilation. The potential effects of confounding factors such as age and pre-treatment preventing to identify the main factor affecting drought tolerance in black spruce seedlings were discussed.
Keywords
Artificial Regeneration, Boreal Forest, Containerized Plants, Planting, Water Stress
Introduction
Quick and successful post-cutting regeneration ensures a continuous and sustained productivity of the stands as well as the ecological functions and ecosystem services of the forest to be maintained over time. Forest management activities thus have to guarantee an appropriate regeneration of harvested stands. In Canada, between 60 and 70% of the harvested area (mainly conifer stands) is regenerated by artificial means, mainly by planting and seeding to efficiently achieve the desired density and species composition of the future stands ([35]). The huge surface involved has extended the planting period during summer in all boreal regions ([44], [36]). In these ecosystems, seedlings planted in late spring benefit from the fresh moist soil conditions due to still low temperatures and abundant water released with snowmelt ([31], [40]). However, in summer soils lose water at a higher rate than that supplied by precipitation, and soil water content has been observed to decrease down to 20% during the driest and hottest summer days even at the higher latitudes ([3]). The seedlings newly outplanted under these unfavorable conditions are potentially exposed to water stress and non-optimal temperatures ([43]).
Artificial regeneration is a useful but expensive component of forest management, with 80% of the plantation costs being directly related to the seedlings ([23]). However, seedling survival and growth rates under transient or persistent unfavorable environmental conditions can substantially affect the success and price of the procedure. There can also be important logistic and economic constraints, mainly when the artificial regeneration has to be established in remote stands far from the seedling production areas. In the last decade, there has been increasing interest in the use of smaller and younger seedlings ([30], [17]). Such a strategy has evident financial advantages during the production and planting process. In the nursery, smaller seedlings require less storage space and handling, and a shorter period of growth; in the field, transport and planting times and costs are reduced. In coniferous reforestation areas of Sweden, smaller seedlings have shown higher survival rates than bigger ones because of less pine weevil (Hylobius abietis L.) damage ([30], [8]). Competition with the vegetation for light and soil resources can be an important constraint for small seedlings, which show reduced shoot growth during the initial phases after planting ([11], [23]). However, the competitive disadvantage of small seedlings has only minimal effects at higher latitudes, where the vegetation is mainly composed of ericaceous shrubs and lichen mat ([17]). Small seedlings could require special precautions during the preparation and planting in the field, as they are expected to dry out faster than seedlings of conventional sizes because of their smaller root system ([30], [23]). Thus, the water availability in the site represents a crucial factor for the success of the regeneration.
Reduced growth is generally observed in seedlings of boreal species during the 2-3 years following planting. Seedlings have roots enclosed in a small rootball that reduce or inhibit the ability of plants to respond to the water requirements for evapotranspiration ([12]). Seedlings of bigger sizes may be considered better adapted to avoid water stress and complete the establishment phase more rapidly due to their higher root biomass and growth, and consequently an increased root-soil contact. Moreover, in respect to small seedlings, the soil volume in the rootball of bigger seedlings may represent a richer reservoir of water available for the plants ([6], [25]) Accordingly, even if economically advantageous, seedlings of small sizes could be less efficient in terms of water and nutrient uptake, which would make them unsuitable in stands with soils that may have low moisture content during the growing season.
The aim of this paper was to assess the response to a severe water deficit of seedlings of different sizes growing under controlled conditions simulating extreme post-planting conditions. The hypothesis that the small size makes seedlings more sensitive to water stress was tested. Such relationships have been in part analyzed previously, but comparisons were made only for seedlings of big sizes and, to our knowledge, no study is available on the responses to water stress of small seedlings (i.e., those produced in cavities with volume of less than 50 cm3 - [28], [22]). In this study, black spruce (Picea mariana (Mill.) BSP) was used as study species because of its transcontinental distribution and utilization in the boreal forest of northern North-America and its well-known responses to water stress ([16], [21], [37]).
Material and Methods
Experimental design
The investigation was conducted on 2- and 3-year-old black spruce seedlings of different sizes and named C25, C50, C110 and C350, according to the cavity volume of the containers in which they were grown (Tab. 1). The containers for C50, C110 and C350 represented the sizes most used for boreal species. Diameter, height and biomass of seedlings increased while root density decreased with the cavity volume of the container. The container for C25 was specifically designed in 2006 at the Université du Québec à Chicoutimi (Canada) and was characterized by small cavities arranged in a honeycomb pattern (Fig. 1). All containers were produced by IPL Inc. (Saint Damien, Quebec, Canada). Before the experiment, the seedlings spent the first year in plastic tunnels, and were then transferred to an open field for their second (C25 and C50) and third (C110 and C350) growing season. In summer of the second year, C25 were again transferred into tunnels for two weeks in order to receive a short-day treatment with a photoperiod of 8 h. This additional treatment was applied to C25 according to the criteria required by the Ministère des Ressources Naturelles ([34]) for enabling seedlings to withstand the summer environmental conditions. The plant material homogeneity was verified by measuring the morphological characteristics of 20 seedlings per size randomly sampled before the experiment (Tab. 1).
Tab. 1 - Characteristics of the seedlings (mean ± standard deviation, n=20) and their growing containers (C25, C50, C110, C350 - see also Fig. 1). (DW): dry weight.
Item | Characteristics | C25 | C50 | C110 | C350 |
---|---|---|---|---|---|
Containers | Number of cavities | 113 | 67 | 45 | 25 |
Cavity depth (cm) | 7.5 | 8 | 12 | 12.5 | |
Cavity volume (cm3) | 25 | 50 | 110 | 350 | |
Seedlings | Seedling age (yrs) | 2 | 2 | 3 | 3 |
Seedling height (cm) | 12.82 ± 1.04 | 20.28 ± 2.53 | 31.81 ± 3.77 | 71.32 ± 4.38 | |
Diameter at collar (mm) | 1.9 ± 0.16 | 2.54 ± 0.20 | 3.71 ± 0.71 | 8.18 ± 0.68 | |
height:diameter ratio (cm/mm) | 6.80 ± 0.85 | 8.01 ± 1.10 | 8.80 ± 1.57 | 8.76 ± 0.84 | |
Needles (DW g) | 0.23 ± 0.05 | 0.53 ± 0.11 | 1.27 ± 0.34 | 7.02 ± 1.70 | |
Stem (DW g) | 0.19 ± 0.05 | 0.39 ± 0.09 | 0.95 ± 0.28 | 9.40 ± 1.59 | |
Roots (DW g) | 0.22 ± 0.06 | 0.49 ± 0.12 | 0.93 ± 0.35 | 6.67 ± 1.89 | |
Shoot:root ratio | 1.90 ± 0.31 | 1.91 ± 0.35 | 2.56 ± 0.63 | 2.61 ± 0.70 | |
Root density (cm3 cm-3) | 2.56 ± 1.40 | 6.60 ± 2.45 | 4.24 ± 1.00 | 7.00 ± 3.63 |
On 24 July 2007, the seedlings were transplanted in 10 raised beds (240 cm × 120 cm × 14 cm) arranged in 5 rows (blocks) and filled with sand (<1% in loam). Therefore, the spacing between the seedlings was 10 × 12 cm, which assured no interaction or competition between the developing roots of seedlings. The raised beds were located in a plastic tunnel equipped with a forced ventilation set at 25 °C. Eighty seedlings were planted along 10 rows per raised bed, and each row received two seedlings per size according to a random pattern. A row of C50 was planted along the perimeter of the raised bed to minimize the edge effect. Each block involved two raised beds randomly representing the control and the treatment. The raised beds were fertilized twice with 10 g of NPK (20-20-20) during two weeks of acclimatization.
Overall, the experiment lasted 25 days. The seedlings were irrigated every two days to maintain a volumetric soil water content of between 6 and 8%, which was evaluated daily in each container by using a TDR probe (model MP-917, ESI Environmental Sensors Inc., Victoria, BC, Canada). Treated seedlings were submitted to a water stress beginning on 6 August during which irrigation was withheld for 14 days. After that period, irrigation was restored at the same frequency as the control, with measurements being maintained for the successive 11 days. Maximum and minimum air temperature and relative humidity were measured daily using two digital hygrometer-thermometer recorders (Fisher Scientific, Pittsburgh, PA, USA) located close to the seedlings.
Data collection
Physiological measurements were taken during and after the treatment for a total of 9 sampling days and consisted of pre-dawn (Ψ
pd) and midday (Ψ
md) leaf water potential, stomatal conductance to water vapour (g
s) and net photosynthesis (P
n). On each sampling day, 80 seedlings were randomly selected for data collection, 40 during pre-dawn and 40 during midday measurements. Ψ
was measured with a pressure chamber (PMS Instruments, Corvalis, OR, USA). A
n and g
s were measured on the current-year needles of the apical arrow from 9:00 to 11:00 under saturating irradiance conditions (1200 µmol m-2 s-1) using a portable photosynthesis system LI-6400 (LI-COR, Inc., Lincoln, NB, USA) equipped with a conifers-adapted chamber of 0.25 l. Measurements were expressed according to the needle dry mass, which was assessed after drying for 48 h at 65 °C. After the measurements of gas exchange, Ψ
md was assessed on the same seedlings, the root system was collected, sealed in a plastic bag, and stored in the dark at 4 °C. The roots were carefully cleaned in water, dried for 48 h at 65 °C and weighed. Root density was determined on fresh samples with the method of volume displacement ([14]).
Statistical analyses
Data were analyzed as split-split-plot with 5 replicate blocks, where each block contained control and treated seedlings. Overall, 720 seedlings were measured (4 sizes × 2 water regimes × 5 blocks × 9 sampling days × 2 periods of measurements). The variables related to physiological measurements were compared with analysis of variance (three-way ANOVA in a block-split-plit-plot design) and Restricted Maximum Likelihood Method REML procedure of the JMP® statistical software (version 10, SAS Institute Inc., Cary, NC, USA) by using seedling size as a categorical variable. The homogeneity of variance was graphically verified on residual plots of the linear models ([33]). The SLICE procedure was used to analyze the interaction treatment × day, while the differences between seedling sizes were tested by orthogonal contrasts ([26]).
Analysis of covariance (ANCOVA) was used to assess the relationships between gas exchange and water potential, and to compare data of root biomass growth among seedling sizes. The models were applied on transformed data to respect the assumption of linearity. Goodness-of-fit of each model involved the significance of F-values, the proportion of variation accounted for (R2) and the distribution of residuals.
Results
Growth conditions
During the overall period of monitoring, minimum and maximum temperatures were on average 13.6 and 30.6 °C, respectively (Fig. 2). When treatment was carried out, from day 0 to 14, the temperature was lower, 12.5 and 29.8 °C for minimum and maximum, respectively. During the day, minimum relative humidity ranged between 17 and 64%, and attained a maximum of 78-97.5% at night. A rapid decrease in soil water content was observed two days after the suspension of irrigation in treated seedlings, which was followed by a gradual water loss reaching 1.4% of volumetric soil water content on day 7 (Fig. 2). After that date, soil water content remained stable until restoration of the irrigation. Two days of irrigations (day 14 and 16) were required for treated seedlings to attain soil water content values of 8.7%, similar to those measured in the control.
Fig. 2 - Environmental conditions and water content measured in black spruce seedlings submitted to water stress. Grey area represents the treatment period. Error bars indicate 95% confidence interval (n=5).
Water potential
The control seedlings of all sizes maintained a similar and constant water potential during the experiment, with average Ψ
pd and Ψ
md of -0.47 and -0.90 MPa, respectively (Fig. 3). The treatment modified both Ψ
pd and Ψ
md in seedlings of all sizes, with differences being statistically significant from day 7 for Ψ
md and 9 for Ψ
pd (Tab. 2). The differences between control and treated seedlings remained significant until day 14. At the end of the treatment period, Ψ
pd and Ψ
md attained values of -2.2 and -2.39 MPa, respectively. The significant interactions between size and treatment (T × S and T × S × D in Tab. 2) indicated that the responses of water potential to stress was related to seedling size. Contrasts revealed that smaller seedlings (C25 and C50) had different Ψ
pd and Ψ
md than bigger seedlings (C110 et C350 - Tab. 3). The differences between C25 and C50 and between C110 and C350 were occasionally significant. Differences in Ψ
pd among seedlings sizes were larger than the differences in Ψ
md (Fig. 3). No difference in water potential between control and treated seedlings was detected on days 21 and 25, after restoration of the irrigation (Fig. 3 and Tab. 2). No seedlings was observed to die during or after the treatment.
Fig. 3 - Physiological responses of black spruce seedlings of different sizes submitted to a water stress treatment. (Ψ
pd): predawn leaf water potential; (Ψ
md): midday leaf water potential; (P
n): net photosynthesis; (g
s): stomatal conductance to water vapour. C25, C50, C110 and C350 represent the different seedling sizes according to the cavity volume of the containers in which they were grown. Grey area represents the treatment period.
Tab. 2 - ANOVA comparisons performed on the physiological measurements collected from black spruce seedlings of different sizes (S) submitted to a water stress treatment (T). (Ψ
pd): predawn leaf water potential; (Ψ
md): midday leaf water potential; (P
n): net photosynthesis; (g
s): stomatal conductance to water vapour. (D0-D25): days since the beginning of the treatment; (ns): not significant.
Source of variation |
ndf | ddf |
Ψ
pd
|
Ψ
md
|
P
n
|
g
s
|
||||
---|---|---|---|---|---|---|---|---|---|---|
F-ratio | p | F-ratio | p | F-ratio | p | F-ratio | p | |||
Treatment (T) | 1 | 4 | 8.36 | <0.05 | 23.28 | <0.01 | 14.61 | <0.05 | 50.53 | <0.01 |
Day (D) | 8 | 64 | 17.25 | <0.0001 | 23.14 | <0.0001 | 28.05 | <0.0001 | 64.99 | <0.0001 |
T × D | 8 | 64 | 11.54 | <0.0001 | 20.31 | <0.0001 | 8.96 | <0.0001 | 9.67 | <0.0001 |
Between T at D0 | 1 | 64 | 0.01 | ns | 0.32 | ns | 0.26 | ns | 0.01 | ns |
Between T at D2 | 1 | 64 | 0.03 | ns | 0.05 | ns | 0.00 | ns | 1.07 | ns |
Between T at D4 | 1 | 64 | 0.06 | ns | 3.77 | ns | 2.08 | ns | 9.03 | <0.01 |
Between T at D7 | 1 | 64 | 2.24 | ns | 17.11 | <0.001 | 25.26 | <0.0001 | 58.93 | <0.0001 |
Between T at D9 | 1 | 64 | 8.05 | <0.05 | 10.09 | <0.01 | 4.02 | ns | 54.46 | <0.0001 |
Between T at D11 | 1 | 64 | 25.78 | <0.0001 | 36.94 | <0.0001 | 33.92 | <0.0001 | 19.20 | <0.0001 |
Between T at D14 | 1 | 64 | 55.21 | <0.0001 | 124.02 | <0.0001 | 34.85 | <0.0001 | 34.46 | <0.0001 |
Between T at D21 | 1 | 64 | 0.01 | ns | 0.24 | ns | 0.26 | ns | 1.70 | ns |
Between T at D25 | 1 | 64 | 0.07 | ns | 1.08 | ns | 0.14 | ns | 3.65 | ns |
Size (S) | 3 | 215 | 1.80 | ns | 8.29 | <0.0001 | 148.06 | <0.0001 | 198.94 | <0.0001 |
T × S | 3 | 215 | 14.92 | <0.0001 | 3.66 | <0.05 | 1.63 | ns | 8.93 | <0.0001 |
S × D | 24 | 215 | 3.02 | <0.0001 | 1.14 | ns | 5.26 | <0.0001 | 7.20 | <0.0001 |
T × S × D | 24 | 215 | 2.79 | <0.0001 | 2.20 | <0.01 | 1.17 | ns | 1.86 | <0.0001 |
Tab. 3 - P-values of the orthogonal contrasts calculated for the days with significant effect of the treatment according to the ANOVA shown in Tab. 2. C25, C50, C110 and C350 represent the different seedling sizes according to the cavity volume of the containers in which they were grown. (ns): not significant.
Contrasts | Day |
Ψ
pd
|
Ψ
md
|
P
n
|
g
s
|
---|---|---|---|---|---|
(C25, C50) vs. (C110, C350) | 4 | ns | <0.01 | <0.0001 | <0.0001 |
7 | <0.01 | <0.001 | <0.0001 | <0.01 | |
9 | <0.0001 | ns | <0.0001 | <0.0001 | |
11 | <0.0001 | <0.01 | ns | ns | |
14 | <0.0001 | <0.01 | <0.05 | ns | |
C25 vs. C50 | 4 | ns | ns | ns | ns |
7 | ns | ns | ns | ns | |
9 | <0.05 | ns | ns | ns | |
11 | ns | ns | ns | ns | |
14 | ns | ns | ns | ns | |
C110 vs. C350 | 4 | ns | <0.001 | <0.0001 | ns |
7 | <0.01 | ns | <0.01 | ns | |
9 | <0.01 | ns | <0.01 | <0.05 | |
11 | <0.0001 | ns | ns | ns | |
14 | ns | ns | ns | ns |
Gas exchange
P
n and g
s of control seedlings were generally higher in C25, while C350 showed markedly lower but more stable values during the monitoring period (Fig. 3). The treatment significantly affected gas exchange (Tab. 2). P
n differed between control and treated seedlings on days 7, 11 and 14, with the timings of response to water stress being related to the seedling size (significant interaction S × D in Tab. 2). On day 9, the smaller seedlings (C25 and C50) still showed higher P
n than bigger seedlings (C110 and C350). Similarly, C350 had the lowest P
n until day 9 (Tab. 3). Eleven days after the suspension of irrigation, seedlings of all sizes attained similar P
n values (on average 0.02 µmol CO2 g-1 s-1), but markedly lower than those measured on the control (0.08 µmol CO2 g-1 s-1). At the end of water stress, on day 14, P
n was statistically higher on smaller (C25 and C50) than on bigger seedlings (C110 and C350) (Tab. 3).
The effect of treatment on gas exchange was evident particularly for g
s, with differences between control and treated seedlings appearing 4 days after the suspension of irrigation (Fig. 3 and Tab. 2). On day 14, g
s was on average 0.79 and 0.07 mmol g-1 s-1 in control and treated seedlings, respectively. Smaller seedlings (C25 and C50) maintained higher g
s than bigger seedlings (C110 and C350) on days 4-9. In general, C350 exhibited the lowest values of g
s in both control and treated seedlings (Tab. 3). The response in g
s of seedlings to the treatment was related to their size, as confirmed by the significant interaction T × S (Tab. 2).
The relationships between P
n and g
s and Ψ
pd were correctly represented by the ANCOVA models, as revealed by the significant F-values and the high R2, which ranged between 0.70 and 0.72 (Tab. 4). The residuals were homogeneously distributed around zero, and showed no pattern, except a slight heteroscedasticity for P
n. The general trend, which is represented in Fig. 4 by applying the inverse transformations on the model predictions for clarity, revealed a slow growth of the curves at low Ψ
pd, followed by higher growth rates, producing an exponential pattern, mainly in g
s. Gas exchanges were positively correlated with water potential according to a non-linear pattern, and were related to seedling size, as also confirmed by the ANCOVA models (Tab. 4). For all seedling sizes, lower Ψ
pd corresponded to decreases in P
n and g
s (Fig. 4). However, for the same Ψ
pd, smaller seedlings exhibited higher P
n and g
s. The interaction Ψ
pd × seedling size was significant for P
n, which indicated that seedlings of different sizes produced different slopes of the curves.
Tab. 4 - Results of the ANCOVA models performed between P
n and g
s and Ψ
pd measured on black spruce seedlings of different sizes. The analysis was performed on transformed data to respect the assumption of linearity. (ns): not significant.
Dependent variable |
Source of variation |
Regressors | Model | ||||
---|---|---|---|---|---|---|---|
Type III SS | F-value | P | F-value | P | R2 | ||
P
n
|
Ψ
pd
|
0.034 | 81.11 | <0.0001 | 21.73 | <0.0001 | 0.70 |
seedling size | 0.013 | 10.42 | <0.0001 | ||||
Ψ pd × seedling size |
0.005 | 3.91 | <0.05 | ||||
g
s
|
Ψ
pd
|
6.887 | 112.94 | <0.0001 | 23.57 | <0.0001 | 0.72 |
seedling size | 0.629 | 3.44 | <0.05 | ||||
Ψ pd × seedling size |
0.134 | 0.74 | ns |
Fig. 4 - Relationships between P
n and g
s and Ψ
pd measured in black spruce seedlings of different sizes. The analysis was performed on transformed data to respect the assumption of linearity. (Ψ
pd): predawn leaf water potential; (P
n): net photosynthesis; (g
s): stomatal conductance to water vapour. C25, C50, C110 and C350 represent the different seedling sizes according to the cavity volume of the containers in which they were grown.
Root biomass
At the beginning of the treatment, root biomass ranged between 194 to 7820 mg (Fig. 5). During the experiment, the roots grew significantly, as detected by the significant effect of day (Tab. 5). Root biomass was clearly related to seedling size, with bigger seedlings exhibiting higher root weights. Although the regression of C25 had a steeper slope in treated seedlings, the interaction D × T was not statistically significant (p>0.05), which indicated that root growth rates were similar between seedling sizes. No effect of water stress was observed on the growth in root biomass (Tab. 5).
Fig. 5 - Root biomass of black spruce seedlings of different sizes submitted to a water stress treatment. Vertical axis is scaled at common logarithm. Grey area represents the treatment period.
Tab. 5 - ANCOVA comparisons performed on root biomass of black spruce seedlings of different sizes submitted to a water stress treatment. The analysis was performed on log-transformed data to respect the assumption of linearity. (ns): not significant.
Source of variation | ndf | ddf | F-ratio | p |
---|---|---|---|---|
Treatment (T) | 1 | 4 | 0.66 | ns |
Day (D) | 8 | 64 | 15.83 | <0.0001 |
D × T | 8 | 64 | 1.80 | ns |
Size (S) | 3 | 215 | 1237.71 | <0.0001 |
T × S | 3 | 215 | 1.51 | ns |
S × D | 24 | 215 | 1.20 | ns |
T × S × D | 24 | 215 | 0.71 | ns |
Discussion
This experiment investigated some physiological responses of seedlings of different sizes to a water stress produced under controlled environmental conditions. Before the resumption of irrigation on day 14, treated seedlings attained Ψ
pd of between -1.71 and -2.28 MPa, indicating a severe water stress for black spruce ([7], [1]). In general, both during and after the treatment, water potential and gas exchanges in C25, the seedlings of smallest size, were similar or higher than those measured in bigger seedlings (C50, C110 and C350). These results could in part be related by the short-day treatment that C25 seedlings received before the experiment. Reductions in Ψ
pd corresponded to declines in P
n and g
s in all seedlings, but for the same Ψ
pd, smaller seedlings exhibited higher values of gas exchanges. No effect of the water stress was observed on the biomass of roots, which, although different in terms of absolute value between small and big seedlings, increased at the same rate in all seedling sizes. As a result, the initial hypothesis that small seedlings are more sensitive to a water stress was definitely rejected.
The stomatal conductance to water vapor of needles showed a higher sensitivity to water potential, with a clear reduction observed at Ψ
pd of approximately -1.0 MPa, 4 days after the suspension of the irrigation. These results are in agreement with previous studies and confirmed that Ψ
pd of -1.0 MPa are sufficient to produce responses in black spruce ([1], [46]). By reducing stomatal conductance to prevent water loss, seedlings substantially reduce their ability to assimilate carbon ([46]). However, photosynthesis remained unaffected, or only slightly affected, by the treatment until day 11, when Ψ
pd ranged between -2 and -1.5 MPa. The high values of P
n at Ψ
pd of -1.0 MPa may thus suggest that the reduction in efficiency of water translocation or the effects of hydraulic failure through cavitation in 2- and 3-year-old seedlings could occur only when Ψ
pd reaches -1.5 MPa. One week after the resumption of irrigation, water potential and gas exchanges measured in treated individuals had attained values similar to the control. At this point, seedlings of all sizes could be considered to have completely recovered their optimal water balance and growth.
Growth conditions during the experiment
The experiment produced two contrasting water conditions during the treatment period, with volumetric soil water content decreasing quickly after the suspension of irrigation and reaching 1.4% within one week. This was related to the use of a sandy substrate that allowed a rapid water loss. Such characteristics only partially represent the conditions experienced by planted seedlings on typical post-cutting soils in black spruce stands, which are deep and rich in organic material ([38]). However, black spruce regenerates naturally from the aerial seed banks released by its semi-serotinous cones during the immediate postfire periods, within three years after fire, mainly on mineral soils with a thin or missing layer of burnt humus ([41]). Microsites with concave profiles or mosses are colonized more frequently by the seedlings, demonstrating that moisture is crucial for survival of black spruce regeneration ([47]). Moreover, the plastic tunnels used in this investigation were not equipped to maintain an ideal temperature and relative humidity for growth. Thus, during the day temperatures exceeding 30 °C were measured and relative humidity decreased to 20-40%. Such environmental conditions, if prolonged, may inhibit root growth or damage the plant tissues in species adapted to cold and relatively humid climates ([9], [29]). Overall, the environmental conditions tested in this experiment could be considered less favorable to seedling growth in terms of water availability than those commonly met in post-fire stands by natural regeneration or in post-cutting areas destined to artificial regeneration.
Factors affecting drought tolerance
C25 had the lowest root density compared to seedlings of the bigger sizes, indicating that in proportion, a higher volume of soil was available for the roots of smaller seedlings, which have lower needle biomass and consequently need less water for the evapotranspiration process ([19], [18]). The lower shoot:root ratio of smaller seedlings could also explain their ability to sustain an appropriate water status of the tissues. Increasing shoot:root ratios indicate the occurrence of higher proportions of leaves in respect to roots, whose biomass could be insufficient or less adequate to satisfy the water requirements for maintaining gas exchanges and photosynthesis. According to Bernier et al. ([4]) the previous statement concerns bare-root seedlings and should be taken with caution in the case of containerized seedlings, which should experience no limiting factor. However, the container itself may represent a physical factor constraining root growth, as also deduced by the higher root density observed in C350 with respect to C25. It is well known that water absorption efficiency and seedling survival are negatively affected at high root densities ([2], [42]). Moreover, although the biomass of each compartment increased according to the seedling size, bigger seedlings invested more in producing needles than roots, thus modifying the proportion between roots and needles (compare the dry weight of needles and roots in Tab. 1).
The growth of new roots is a key factor for the successful establishment of seedlings after planting. The definitive establishment allows a sufficient rate of water and nutrient uptake to be sustained in the long term or during stressful events, which can occur early and frequently in boreal forests ([39], [44]). Root growth can be affected by water stress that inhibits division and differentiation of the cells at the apical meristem of roots ([20]). Root growth is also related to the availability of photosynthates for meristem development ([27]). Under similar environmental conditions and water potentials, the smaller seedlings demonstrated an ability to maintain higher rates of gas exchanges than the other seedlings. Delayed stomatal closures ensure the CO2 assimilation for completing the photosynthetic process even under unfavorable environmental conditions. Accordingly, the slope of the regression for C25 was steeper than those estimated for the other seedling sizes, suggesting higher growth rates in smaller seedlings. However, no statistical difference in root growth was observed during the treatment. Thus, it was concluded that root growth in smaller seedlings was comparable to that observed in the other seedling sizes.
Size vs. age effect
The slow growth of black spruce prevents sizes suitable for planting to be attained within short periods. As a result, in seedlings of this species the size is intrinsically dependent on age. Moreover, production of the experimental seedlings carefully respected the standard criteria adopted by the forest tree nurseries in Quebec to make the results representative and effective for practitioners. Thus, in this investigation, the factor age covariated with seedling size, as either 2- or 3-year-old seedlings were used for small or big seedlings, respectively. This prevented identification of the main factor triggering the physiological performances of seedlings. Bigger seedlings have a less branched root system and lower proportions of unsuberized fine roots, and could be less efficient in water and nutrient uptake ([24]). This different permeability of roots could also explain the higher concentration of several foliar nutrients observed in C25 compared to C50 ([45]). The higher metabolism and greater capacity for enhancing photosynthesis should allow smaller and younger plants to endure the stress ([5]). Although there is evidence that the effect may be ascribed mainly to seedling size rather than age ([32]), in this work the correlated factors size and age were not disentangled. As a result, the question whether the differences observed were connected to the physiological features related to seedling size or age remained unanswered.
Other effects
Before the experiment, C25, the smaller seedlings, were transferred to tunnels for two weeks to receive a short-day treatment. This was not provided for the seedlings of the other sizes. Such a treatment is contemplated by the protocol of forest tree nurseries of Quebec and currently used to induce growth cessation and bud set in seedlings, mainly for those planted in summer, which should be better enabled to withstand summer environmental conditions ([11]). Compared with seedlings grown under long-day conditions, there is agreement that this treatment increases root growth and drought and frost tolerance of seedlings ([10], [13], [31]). The short-day treatment is expected to promote photosynthesis, reduce respiration, and increase the proportion of carbon allocated to roots ([15]). Thus, the performance observed in C25 during the investigation could be in part attributed to the short-day treatment received before the experiment.
Another factor possibly affecting the results was related to the characteristics of containers. As shown in Fig. 1, the largest container had side slits, which were missing in the other containers. There is a potential for the side slits to have altered the morphology or post-planting growth of the roots, in addition to the intended effect of container size. Although this factor could not be excluded and should be taken into account, no marked effect was detected in the results.
Conclusions
Post-cutting regeneration is a critical period for successful and effective forest management, and is costly in the case of seeding or planting. The resistance to stress of seedlings during their first phases after planting is fundamental for avoiding a growth check and assuring fast establishment and long-term survival. There is increasing interest in seedlings of small sizes for artificial regeneration because they need less storage space and handling. However, the resistance of small seedlings to water deficit is questioned. In this work, the response to a severe water deficit produced by a suspension of irrigation for 14 days was assessed in black spruce seedlings of different sizes. Smaller seedlings exhibited similar or higher water potential and gas exchanges than bigger seedlings both during and after the treatment. The root growth of both small and big seedlings was not affected by the water stress. The initial hypothesis that small seedlings are more sensitive to a water stress was thus definitely rejected. The performances observed in smaller seedlings were attributed to higher ability of roots to sustain the evaporative needs of needles under water deficit and to maintain higher rates of carbon assimilation. However, the smaller seedlings were also necessarily younger, thus the question whether the differences observed were connected to their size or age remained unanswered.
The results of this experiment suggested that the performance in terms of tolerance to water stress and maintenance of root growth during a water deficit was similar in seedlings of small and big size, even under temperatures warmer than those generally occurring in typical boreal forests dominated by black spruce. Moreover, delayed stomatal closures were observed to occur in smaller seedlings, which allowed higher rates of photosynthesis to be maintained than in bigger seedlings. Given the financial and logistic advantage of using smaller seedlings in artificial regeneration, their utilization should be seriously considered and tested in boreal stands experiencing occasional drought events or non-optimal soil moisture conditions.
References
Gscholar
Online | Gscholar
Gscholar
Gscholar
Gscholar
Gscholar
Gscholar
Gscholar
Gscholar
CrossRef | Gscholar
Authors’ Info
Authors’ Affiliation
Sergio Rossi
Daniel Lord
Département des Sciences Fondamentales, Université du Québec à Chicoutimi, 555 Boulevard de l’Université, Chicoutimi (QC), G7H2B1 (Canada)
Corresponding author
Paper Info
Citation
Walsh D, Rossi S, Lord D (2014). Size and age: intrinsic confounding factors affecting the responses to a water deficit in black spruce seedlings. iForest 8: 401-409. - doi: 10.3832/ifor1412-007
Academic Editor
Giustino Tonon
Paper history
Received: Jul 30, 2014
Accepted: Oct 17, 2014
First online: Dec 09, 2014
Publication Date: Aug 02, 2015
Publication Time: 1.77 months
Copyright Information
© SISEF - The Italian Society of Silviculture and Forest Ecology 2014
Open Access
This article is distributed under the terms of the Creative Commons Attribution-Non Commercial 4.0 International (https://creativecommons.org/licenses/by-nc/4.0/), which permits unrestricted use, distribution, and reproduction in any medium, provided you give appropriate credit to the original author(s) and the source, provide a link to the Creative Commons license, and indicate if changes were made.
Web Metrics
Breakdown by View Type
Article Usage
Total Article Views: 48229
(from publication date up to now)
Breakdown by View Type
HTML Page Views: 42085
Abstract Page Views: 2094
PDF Downloads: 2871
Citation/Reference Downloads: 21
XML Downloads: 1158
Web Metrics
Days since publication: 3635
Overall contacts: 48229
Avg. contacts per week: 92.88
Article Citations
Article citations are based on data periodically collected from the Clarivate Web of Science web site
(last update: Feb 2023)
Total number of cites (since 2015): 4
Average cites per year: 0.44
Publication Metrics
by Dimensions ©
Articles citing this article
List of the papers citing this article based on CrossRef Cited-by.
Related Contents
iForest Similar Articles
Research Articles
Conservation of Betula oycoviensis, an endangered rare taxon, using vegetative propagation methods
vol. 13, pp. 107-113 (online: 23 March 2020)
Research Articles
Linking nursery nutritional status and water availability post-planting under intense summer drought: the case of a South American Mediterranean tree species
vol. 9, pp. 758-765 (online: 03 June 2016)
Research Articles
Germination and seedling growth of holm oak (Quercus ilex L.): effects of provenance, temperature, and radicle pruning
vol. 7, pp. 103-109 (online: 18 December 2013)
Review Papers
Problems and solutions to cork oak (Quercus suber L.) regeneration: a review
vol. 16, pp. 10-22 (online: 09 January 2023)
Research Articles
Controlled-release fertilizers combined with Pseudomonas fluorescens rhizobacteria inoculum improve growth in Pinus halepensis seedlings
vol. 8, pp. 12-18 (online: 12 May 2014)
Research Articles
Forecasting the field performance of Austrian pine seedlings using morphological attributes
vol. 10, pp. 99-107 (online: 13 October 2016)
Research Articles
Use of alternative containers for promoting deep rooting of native forest species used for dryland restoration: the case of Acacia caven
vol. 10, pp. 776-782 (online: 02 September 2017)
Research Articles
Growth and physiological acclimation to shade in young plants of Adesmia bijuga Phil., a critically endangered species in central Chile
vol. 14, pp. 307-312 (online: 01 July 2021)
Research Articles
Effects of brassinosteroid application on seed germination of Norway spruce, Scots pine, Douglas fir and English oak
vol. 10, pp. 121-127 (online: 02 October 2016)
Research Articles
Substrates and nutrient addition rates affect morphology and physiology of Pinus leiophylla seedlings in the nursery stage
vol. 10, pp. 115-120 (online: 02 October 2016)
iForest Database Search
Search By Author
Search By Keyword
Google Scholar Search
Citing Articles
Search By Author
Search By Keywords
PubMed Search
Search By Author
Search By Keyword