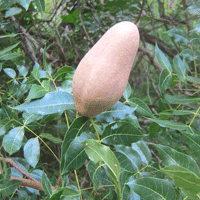
Nursery practices increase seedling performance on nutrient-poor soils in Swietenia humilis
iForest - Biogeosciences and Forestry, Volume 8, Issue 4, Pages 552-557 (2014)
doi: https://doi.org/10.3832/ifor1179-007
Published: Dec 09, 2014 - Copyright © 2014 SISEF
Technical Reports
Abstract
Swietenia humilis is a valued tree species for its high-quality wood, among other commercial interests. Attempts to plant the species in southwest Mexico have often failed due to poor quality seedlings combined with low soil fertility and dry environments. Nursery top-pruning and fertilization are practices previously reported to improve seedling quality, and facilitate rapid establishment under poor site conditions. In the present study, the effects of three top-pruning intensities (0%, 25%, and 50%), and two fertilizer regimes (traditional and exponential) on several S. humilis seedling morphological and physiological indices were tested in the nursery, and a quality test trial on nutrient-poor soils was conducted. Significant interactions between the above two treatments were not detected. Top pruning at 25% and 50% intensity did not improve S. humilis seedling quality. However, exponential nutrient supply exhibited favorable effects on seedling growth. Results of the quality test trial revealed exponential fertilization promoted satisfactory seedling performance when low nutrient availability was a limiting factor.
Keywords
Caobilla, Seedling Quality, Top Pruning, Exponential Fertilization
Introduction
Caobilla (Swietenia humilis Zucc., Meliaceae) is a small to medium-sized tree (15-20 m tall) native to the Mesoamerican Pacific tropical dry forest zones, and considered a valuable hardwood in Mexican Pacific coastal lowlands for the local forest industry ([16]). After decades of commercial over-exploitation, natural populations have been substantially reduced to scattered and small isolated populations. The species was therefore listed under the multilateral treaty CITES (Convention on International Trade in Endangered Species of Wild Fauna and Flora), and classified as vulnerable under the IUCN (International Union for Conservation of Nature) Red List.
Currently, the interest in establishing S. humilis for environmental and commercial purposes has increased in the dry tropics of Mexico and Central America. However, reforestation activities have been limited by low seedling survival and growth rates, largely because nurseries do not produce high-quality stock of container seedlings for outplanting ([8]).
Current practices in many forest nurseries involve container seedlings planted in local forest soil and little or no fertilizer application. Regardless of the production system, seedling germplasm quality along with cultural practices serve a major role in promoting successful morphological and physiological characteristics, resulting in satisfactory plant performance at the plantation site ([26], [30], [40], [14], [36]).
Drought and high temperatures in the dry tropics can limit nutrient availability, which often severely affects reforestation ([17]). However, success can be achieved by establishing seedling plantations during favorable seasons, using rapidly acclimating plant material. Villar-Salvador et al. ([36]) reported seedlings with high growth rates due to high photosynthetic performance and increased capacity to remobilize nutrients were well-suited to the above conditions; and such attributes were favored in large seedlings under high nutrient concentrations. As a consequence, cultivation practices that enhance these traits might increase S. humilis seedling quality.
Top-pruning seedlings in the nursery is an uncommon practice; nevertheless, Cetina et al. ([4], [5], [6]) provided evidence in Pinus greggii that the approach can increase seedling growth and photosynthetic capacity, resulting in favorable effects on seedling field performance. However, Wilson ([38]) demonstrated success was dependent on pruning intensity and removal as a consequence of the apical dominance effect ([38]).
Seedling fertilization is a common nursery practice, which serves to enhance growth and photosynthetic capacity. Reserves can be accumulated through fertilization when nutrient availability exceeds seedling requirements ([7], [34], [31]), with the benefit of later remobilization when nutrient availability becomes a limiting factor. Different fertilization regimes, including exponential fertilization have been established to promote these conditions ([34], [31], [12]).
Top-pruning and exponential fertilization effects in the nursery have not been documented in S. humilis, although improvement in seedling quality has been reported for many other forest species ([4], [19], [37]). In this study, we examined top-pruning and fertilization effects on S. humilis seedlings by respectively evaluating various morphological and physiological indices in the nursery, and a quality test trial on nutrient-poor soils.
Material and methods
The study was conducted under greenhouse conditions in the Nursery of the Forestry Graduate School of Colegio de Postgraduados in Montecillo, Texcoco, Mexico (19° 19′ N, 98° 53′ W; 2250 m a.s.l.). Greenhouse conditions were maintained at an average 30/15 °C (day/night), a 16 h (day/night) photoperiod, and 70-85% relative humidity.
Seeds were collected from healthy S. humilis individuals in a tropical dry forest in the Tierra Caliente, Guerrero, Mexico. In August 2012, 1000 seeds were field collected, scarified, and placed in running water for 24 h to sow directly into Jiffy-7 Forestry® compressed peat pellets. One seed per pellet was sown. Germination and emergence was observed during the last week of August 2012. Seven weeks later on 19 October 2012, 384 seedlings of approximately the same height (9-10 cm) were selected and organized into groups of 16 seedlings, each group corresponding to an experimental unit. Six treatment combinations with four replicates per treatment resulting in a 3×2 factorial arrangement were randomly assigned to each of the 24 experimental units in a completely randomized design.
The two factors tested were top-pruning (TP), with three levels (no-pruning - NP, TP 25%, and TP 50% intensity), and fertilization, with two levels (traditional fertilization - TF, and exponential fertilization - EXF). The six treatment combinations were labeled as T1 (NP + TF), T2 (TP 25% + TF), T3 (TP 50% + TF), T4 (NP + EXF), T5 (TP 25% + EXF), and T6 (TP 50% + EXF). Depending on the effects, NP and TF levels, and their combinations (NP + TF), were considered controls.
Treatments were initiated on 22 October 2012, when nutrient reserves in the seedling endosperm were largely exhausted. Top-pruning (TP) was conducted once, when fertilization was first applied, by removing the stem portion between the second node and the apex (including foliage). Fertilization was further supplied from 22 October to 28 December 2012. The fertilizer amount supplied was designed for a 10-week period, and based on nitrogen (N) concentration estimates in seedling foliage.
TF treatment followed the PRONARE-CONAFOR fertilization program for tropical species using Peters® fertilizers ([1]), which were adjusted to commercial formulations based on seedling developmental stage. Under the TF fertilization regime, seedlings were treated with Peters Professional® Seedling Starter™ (9 N: 45 P2O5:15 K2O) at concentrations of 25 mg N l-1 for a total of six applications, i.e., three weeks, two applications per week. Seedlings were administered eight applications of Peters Professional® General Purpose™ (20 N: 10 P2O5: 20 K2O) at concentrations of 50 mg N l-1 with the same frequency over the following four weeks. During the final three weeks, a total of six treatments, two per week of Peters Professional® Conifer Finisher™ (4 N: 25 P2O5: 35 K2O) were applied at a 50 mg N l-1 concentration.
The EXF treatment was designed to supply nutrients exponentially, increasing the fertilizer addition levels through time. Calculations were derived from an exponential function described by Timmer ([34] - eqn. 1):
where t
is the number of fertilizer applications during the nursery stage, N
T is the fertilizer amount applied on a specific day t, N
(t-1) is the cumulative fertilizer treatment amount at a specific time (including the previous application), and N
S is the initial N content in newly germinated seedlings (7.12 mg N seedling-1). A random sample of 30 newly germinated seedlings was analyzed to determine N
S.
The r
factor is the relative nutrient addition rate to increase N
S to the final (N
T + N
S) level, obtained by the following equation (eqn. 2):
where N
T is the amount of fertilizer applied on a specific day t
. Due to limited information on the species nutritional attributes, N
T (27.78 mg N seedling-1) was determined from the N amount applied per seedling using the TF scheme. As in TF, fertilizer was applied twice per week, therefore A
= 20. The doses obtained (mg N seedling-1) for each application date were multiplied by 32. This value was used as a constant considering that for each liter of nutrient solution, 32 seedlings are fertilized. The specific N concentrations used are shown in Fig. 1. For all EXF fertilizer treatments, Peters Professional® General Purpose™ (20 N: 20 P2O5: 20 K2O) was applied to seedlings.
Fig. 1 - Concentration of N per liter of nutrient solution provided in each application of the exponential fertilization regime.
Fertilizer solution pH and electrical conductivity were adjusted following Landis ([24]). Fertilizer residue on seedling leaves was removed with water after each application. Additionally, flushing irrigation was conducted weekly. Treatment fertilizer applications at different concentrations, i.e., TF and EXF were the only nutrient sources, as distilled water was used throughout the experiment.
Three weeks after the final fertilization (21 January 2013), a random sample of 16 seedlings per treatment (four from each replicate) was selected to examine shoot height, root-collar diameter, net photosynthetic rate, and chlorophyll content. Twelve of the above 16 seedlings per treatment were further analyzed for leaf area, shoot and root dry weight, and nitrogen (N), phosphorus (P), and potassium (K) concentrations.
Shoot height (cm) and root-collar diameter (mm) were respectively measured with a graduated ruler and a Mitutoyo digital caliper. The sturdiness index (SI
) was calculated from the ratio between height and root-collar diameter ([20]). Net photosynthetic rate (NPR, µmol m-2 s-1) was measured between 09:00 and 12:00 a.m. with a CIRAS -2 (PP Systems, Haverhill, MA, USA), and chlorophyll content (CC, SPAD units) was indirectly determined by a Minolta SPAD 502 Plus portable chlorophyll meter (Spectum Technologies Inc., Plainfield, IL, USA). These last two measures were derived from the same leaves previously selected based on (apparent) similar physiological age. Leaf area (LA, cm2) was determined with an area meter (3100 Biosciences Li-Cor Biosciences, Lincoln, NE, USA).
Shoot and root dry weight were obtained by oven-drying seedlings at 70 °C for 72 h. Dry weight (g) of aboveground components (shoots + leaves), and roots with shoot-heights and root-collar diameters were combined to estimate the Dickson quality index (DQI
) based on the following equation ([20] - eqn. 3):
Finally, N, P, and K concentrations were determined from tissue samples (three per treatment) integrated over the entire seedling by the micro-Kjeldahl method, using photocolorimetry by reduction with Molibdo-Vanadate and flame emission spectrometry, respectively. Total dry biomass and nutrient concentrations were used to calculate N, P, and K content.
Seedling quality, e.g., growth, development, and establishment at nutrient-poor sites was examined by conducting a quality test trial in February 2013. A 30-cm deep planting-bed with red volcanic rock substrate (tezontle) was constructed to establish low or no nutrient availability. Ten randomly selected seedlings per treatment (a total of 60 seedlings) were sown in rows on the planting bed following a complete randomized design.
The quality test trial ended in May 2013. During the four month period, seedlings were periodically watered with distilled water, which was used to prevent any source of external nutrient supply. Seedling height (cm) and stem diameter (mm) were measured at the beginning and end of the test, and monthly relative growth rates were estimated based on the following equation ([27] - eqn. 4):
where X
1 and X
2 are the variables measured at the first and second assessment time, respectively, and Δt
is the time interval between the two measurements.
Comparisons of the factor effect and its interactions on morphological and physiological quality indices were performed through analysis of variance (ANOVA, α = 0.05). Significant differences among treatment means were analyzed using the post-hoc Fisher’s LSD test (α = 0.05). Logarithmic and square root transformations of raw data were conducted when assumptions of normality and homoscedasticity were violated.
Statistical analyses were carried out using the package INFOSTAT© ([11]). Statistical analyses were not conducted on N, P, and K concentration and content. Instead, average values were reported for each composite sample. Graphical vector analysis ([33], [25]) was performed to interpret nutrient variation among treatments.
Additionally, monthly relative growth rates were analyzed by treatment and not factors, since the quality test was specifically designed to assess seedling performance as a function of nutritional reserves under low soil fertility conditions. Therefore, treatments better reflected the influence of plant nutrient status on seedling growth.
Results
Morphological and physiological quality indices
ANOVA results indicated the main factors, i.e., TP and fertilization regime had significant effects on most morphological and physiological variables analyzed (Tab. 1). However, the interaction between TP and fertilization was not significant for any parameters. In summary, seedlings under the NP and EXF treatments showed the highest values for all variables measured (Tab. 2).
Tab. 1 - ANOVA results for top pruning and fertilization regime effects on morphological and physiological variables related to seedling quality in Swietenia humilis.
Variables | Factors | df | F-value | P-value |
---|---|---|---|---|
Root-collar diameter | Top pruning | 2, 90 | 9.01 | 0.0003 |
Fertilization regime | 1, 90 | 1.32 | 0.2542 | |
Interaction | 2, 90 | 1.47 | 0.2362 | |
Sturdiness index | Top pruning | 2, 90 | 3.01 | 0.0542 |
Fertilization regime | 1, 90 | 5.25 | 0.0243 | |
Interaction | 1, 90 | 1.89 | 0.1572 | |
Shoot dry weight | Top pruning | 2, 66 | 5.93 | 0.0001 |
Fertilization regime | 1, 66 | 10.31 | 0.0176 | |
Interaction | 2, 66 | 0.01 | 0.99 | |
Root dry weight | Top pruning | 2, 66 | 10.01 | 0.0002 |
Fertilization regime | 1, 66 | 1.28 | 0.2614 | |
Interaction | 2, 66 | 0.02 | 0.9754 | |
Dickson”s quality index | Top pruning | 2, 66 | 6.75 | 0.0022 |
Fertilization regime | 1, 66 | 0.56 | 0.4572 | |
Interaction | 2, 66 | 0.06 | 0.9387 | |
Leaf area | Top pruning | 2, 66 | 7.71 | 0.001 |
Fertilization regime | 1, 66 | 33.78 | <0.0001 | |
Interaction | 2, 66 | 0.7 | 0.5025 | |
Chlorophyll content | Top pruning | 2, 90 | 0.24 | 0.791 |
Fertilization regime | 1, 90 | 26.34 | <0.0001 | |
Interaction | 2, 90 | 0.81 | 0.4493 | |
Net photosynthesis rate | Top pruning | 2, 90 | 40.88 | <0.0001 |
Fertilization regime | 2, 90 | 175.3 | <0.0001 | |
Interaction | 2, 90 | 0.59 | 0.5543 |
Tab. 2 - Average values (± SE) of morphological and physiological quality indexes assessed in Swietenia humilis seedlings in response to top pruning and fertilization treatments. Only means for factors having a significant effect after ANOVA are reported. Means with the same letter within each treatment are not significantly different (p>0.05) after Fischer’s LSD test. (NP): No-pruned seedlings; (TP): top-pruned seedlings; (TF): traditional fertilization; (EXF): exponential fertilization; (SI): sturdiness index; (DQI): Dickson quality index; (NPR): net photosynthesis rate; (CC): chlorophyll content.
Variables | Pruning | Fertilization | |||
---|---|---|---|---|---|
NP | TP 25% | TP 50% | TF | EXF | |
Diameter (cm) | 4.97 ± 0.11a | 4.88 ± 0.11a | 4.37 ± 0.11b | - | - |
SI (cm mm-1) | - | - | - | 2.20 ± 0.06a | 2.39 ± 0.06b |
Shoot dry weight (g) | 1.16 ± 0.06a | 0.99 ± 0.06b | 0.79 ± 0.06c | 0.90 ± 0.05a | 1.06 ± 0.05b |
Root dry weight (g) | 0.51 ± 0.03a | 0.41 ± 0.03b | 0.34 ± 0.03b | - | - |
DQI | 0.36 ± 0.02a | 0.31 ± 0.02a | 0.25 ± 0.02b | - | - |
Leaf area (cm2 ) | 73.56 ± 4.07a | 52.21 ± 4.07b | 54.07 ± 4.07b | 46.82 ± 3.33a | 73.28 ± 3.33b |
NPR (µmol m-2 s-1) |
3.54 ± 0.09a | 2.37 ± 0.09b | 2.69 ± 0.09c | 2.18 ± 0.08a | 3.55 ± 0.08b |
CC (SPAD units) | - | - | - | 31.83 ± 0.89a | 38.28 ± 0.89b |
Under the NP treatment, the most divergent morphological and physiological quality index response occurred for shoot and root dry weight, leaf area, and net photosynthetic rate (Tab. 2). NP seedlings accumulated 17% and 47% more shoot biomass than seedlings pruned at 25% and 50%, respectively. Similar results were obtained for root dry weight, where NP control seedlings accumulated 24% and 50% more root biomass compared with TP treatments at 25% and 50%, respectively. Additionally, NP seedlings showed the largest leaf area, 40% and 36% greater than 25% and 50% TP seedlings, respectively. Finally, photosynthetic rate of NP seedlings was 49% higher than 25% TP plants, and 32% higher compared with 50% TP plants.
Results of the fertilization effect showed seedlings under the EXF treatment regime produced 17% more biomass and had 50% more leaf area compared with TF treatment seedlings. Similarly, physiological variables, such as chlorophyll content (CC) and net photosynthetic rate (NPR) were significantly affected by EXF, evidenced by a respective 20% and 60% higher CC and NPR in seedlings compared with those grown under the TF regime (Tab. 2).
N, P, and K concentration and content
Differences in plant nutrient status were detected in response to treatments. Compared with the control treatment (T1), seedlings receiving treatments T4, T5, and T6 exhibited increased N concentration and content, respectively (126% and 157% for T4, 75% and 67% for T5, and 364% and 260% for T6). P concentration in T4 and T6 was respectively 53% and 74% higher than in T1. Similarly, P content in T4 and T6 treatments were respectively 57% and 21% higher than in T1. Finally, only T6 revealed higher K concentration (> 55%) and content (> 20%) than T1 (Tab. 3).
Tab. 3 - Concentration and content of N, P and K in response to pruning and fertilization treatments in Swietenia humilis seedlings in the nursery.
Treatment combination |
Concentration (mg g-1) | Content (mg plant-1) | ||||
---|---|---|---|---|---|---|
N | P | K | N | P | K | |
NP + TF (T1) | 8.1 | 3 | 12.2 | 12.7 | 4.7 | 19.13 |
TP 25% + TF (T2) | 8.1 | 2.4 | 11.4 | 10.56 | 3.13 | 14.87 |
TP 50% + TF (T3) | 9.1 | 2.7 | 11.6 | 9.49 | 2.81 | 12.1 |
NP + EXF (T4) | 18.3 | 4.6 | 11.3 | 32.6 | 8.19 | 20.13 |
TP 25% + EXF(T5) | 14.2 | 3.2 | 11.5 | 21.16 | 4.77 | 17.14 |
TP 50% + EXF (T6) | 37.6 | 4.7 | 18.9 | 45.68 | 5.71 | 22.96 |
Quality test trials in low nutrient substrate (Tezontle)
Seedling growth in the quality test trial revealed significant effects for height (F[5, 54] = 3.4, P < 0.01) and diameter (F[5, 54] = 4.91, P < 0.001), with T4 seedlings showing the highest relative growth rates (Tab. 4).
Tab. 4 - Average relative growth rates in Swietenia humilis seedlings planted in low or null availability of nutrients. Means with the same letter within columns are not significantly different (p>0.05) after Fischer’s LSD test.
Treatment combination |
Relative growth rates | |
---|---|---|
Height (cm cm-1month-1) |
Diameter (mm mm-1month-1) |
|
NP + TF (T1) | 0.10 ± 0.01a | 0.05 ± 0.01ab |
TP 25% + TF (T2) | 0.09 ± 0.01ª | 0.04 ± 0.01ª |
TP 50% + TF (T3) | 0.12 ± 0.01ab | 0.08 ± 0.01bc |
NP + EXF (T4) | 0.15 ± 0.01b | 0.11 ± 0.01d |
TP 25% + EXF (T5) | 0.11 ± 0.01ª | 0.05 ± 0.01abc |
TP 50% + EXF (T6) | 0.14 ± 0.01b | 0.09 ± 0.01cd |
Discussion
Morphological and physiological quality indices
Our results showed TP at 25% or 50% did not improve the morphological or physiological quality of S. humilis seedlings, unless accompanied by EXF fertilization. Indeed, the NP treatment seedlings exhibited the highest value for all quality parameters examined (Tab. 2). The growth responses of TP seedlings, i.e., root-collar diameter, shoot dry weight, leaf area, and DQI
were likely affected by the stress imposed in shoot biomass loss.
Previous reports demonstrated that after defoliation, plants accumulated nonstructural carbohydrates for regrowth to compensate tissue loss ([23], [35]). Chapin et al. ([7]) showed the shoot partially and/or temporarily lost the capacity to produce photosynthates, as accumulation was largely dependent on resource allocation to storage. However, allocation of resources to storage directly competes with growth ([2]). Moreover, growth patterns in different forest species might differ as a consequence of differential biomass allocation to shoots and/or roots ([13]). These trade-offs might partly explain reduced growth in the pruned S. humilis seedlings observed in this study.
Cetina et al. ([4], [5]) suggested the morpho-physiological responses in P. greggi were associated with pruning, and might be beneficial to enhance seedling quality. The same pruning intensity tested in the present study (25% and 50%) was applied to P. greggi seedlings, resulting in increased dry biomass accumulation in shoots and roots, as well as in leaf area and the photosynthetic rate ([4], [5]). However, our findings suggested TP was not a suitable approach to enhance S. humilis seedling quality. First, this lack of congruency might be attributed to among species differences. Cline & Harrington ([9]) observed growth and branching patterns among temperate and tropical species differ. Moreover, Chapin et al. ([7]) suggested the benefits obtained by apical dominance removal can vary as a consequence of different species capacities to store the carbohydrates necessary to recover from pruning. Furthermore, seedling age might partially explain these inconsistencies. Seedlings used by Cetina et al. ([4]) were older than the seedlings investigated in our work. However, young seedlings have not yet developed an efficient root system. Therefore, seedlings cannot recover from the injuries caused by pruning through re-sprouting ([23]), unless the resources necessary to support regrowth are provided by fertilization.
Exponential nutrient loading applied in this study improved the morphological and physiological attributes of S. humilis seedlings, results inconsistent with pruning. Despite the lack of reports on this particular species, the favorable effects of exponential nutrient supply on growth, and other beneficial characteristics in the nursery have been examined by many authors ([29], [31], [32], [19], [12], [37]). Timmer ([34]) reported this technique has the advantage of supplying the nutrient amounts required at each stage of seedling exponential growth. Seedling responses expressed in morphological and physiological quality indices confirmed these effects (Tab. 2).
Enhanced seedling growth, i.e., leaf area and shoot biomass under the EXF treatment also suggested greater resource exploitation, i.e., light and CO2, resulting in increased NPR (Tab. 2). Cuesta et al. ([10]) reported higher photosynthetic rates in P. halepensis seedlings that exhibited greater leaf area. Villar-Salvador et al. ([36]) emphasized a large aerial biomass advantage in capturing the resources required to synthesize new tissues, which lead to a positive seedling growth feedback pathway ([23]). However, plant growth and photosynthetic capacity are dependent on plant nutrient status under greenhouse ([36]) or natural conditions ([39]). Field evidence demonstrated that both processes were limited by mineral nutrient availability ([28]). Furthermore, the rate of carbon gain is a function of the photosynthetic rate, respiration, and tissue loss, which typically exhibits interspecific and environmental variability ([2], [22], [10]).
N, P, and K concentration and content
In the present study, nutrient reserve amounts (N, P, and K) were higher in plants fertilized with the EXF treatment regime (Tab. 3), demonstrating the treatment’s effectiveness in promoting the storage of these resources. Kim et al. ([21]) reported plant nutrient reserves declined at the planting site as a consequence of reduced nutrient availability due to interspecific competition ([34]), or severe soil degradation ([17]). According to Tab. 3, the beneficial effects of exponential fertilization were clearly demonstrated compared with the traditional fertilization regime. Nevertheless, it is worth noting that TP also influenced seedling response to fertilization. Indeed, increased growth and N and P concentration and content were achieved under NP + EFX treatments (T4). However, T6 showed maximum concentration and content of all nutrients (50% TP + EXF), although a lower biomass amount was produced. López & Alvarado ([25]) indicated this type of effect resulted from toxicity; however, we determined growth was reduced by pruning, and not by nutrient excess (Fig. 2).
Fig. 2 - Nomograms for response of nitrogen (a), phosphorus (b), and potassium (c), to the application of different top pruning intensities and different fertilization regimes in Swietenia humilis seedlings in the nursery. Treatment combinations: (T1) no pruning + TF; (T2) pruning at 25% + TF; (T3) pruning at 50% + TF; (T4) no pruning + EXF; (T5) pruning at 25% + EXF; (T6) pruning at 50% + EXF.
Quality test trial n nutrient-limited soils
Results from the quality test in Tezontle (Tab. 4) confirmed seedling nutrient accumulation due to exponential fertilization facilitated growth on nutrient-limited soils. Heiskanen et al. ([18]) obtained similar results in Picea abies, where exponential seedling fertilization clearly allowed rapid seedling establishment on poor sites. However, Everett et al. ([15]) found a complete lack of congruency in results of a study on morphology and nutritional status of Pseudotsuga menziesii var. glauca seedlings.
Varied interpretations of species differential responses to exponential fertilization have been proposed. Burgess ([3]) attributed the limited response of some fast-growing species to fertilizer addition rates; at early developmental and growth stages, the fertilizer amounts might be insufficient to meet seedling requirements. However, toxic fertilizer addition rates might also generate detrimental effects ([12]).
Based on our short-term trial results, the doses applied (17 to 412 mg N l-1) using a complete formula of soluble fertilizer (20 N: 20 P2O5: 20 K2O) resulted in beneficial effects on accumulation reserves to support growth, when no external nutrient supply was available. However, we cannot exclude toxic effects due to exponential fertilization, particularly when the 50% TP + EXF treatment was administered. Indeed, exponential fertilization assumes a relationship between fertilizer dose and plant size ([34]), while in the above treatment, plant size, i.e., pruning reduced biomass.
Acknowledgments
We are grateful to Celi G. Calixto Valencia, Noé Bahena Tamayo† and Itzel Dinoran Galindez for their technical support.
References
Gscholar
Gscholar
Gscholar
Gscholar
Gscholar
Gscholar
CrossRef | Gscholar
Gscholar
Gscholar
Gscholar
Gscholar
CrossRef | Gscholar
Gscholar
CrossRef | Gscholar
Authors’ Info
Authors’ Affiliation
Colegio de Postgraduados, campus Montecillo, Km. 36.5 Carretera Federal México-Texcoco, 56230, Montecillo (México)
Corresponding author
Paper Info
Citation
Basave Villalobos E, Alcalá Cetina VM, López López MA, Aldrete A, Del Valle Paniagua DH (2014). Nursery practices increase seedling performance on nutrient-poor soils in Swietenia humilis. iForest 8: 552-557. - doi: 10.3832/ifor1179-007
Academic Editor
Alberto Santini
Paper history
Received: Nov 12, 2013
Accepted: Nov 28, 2014
First online: Dec 09, 2014
Publication Date: Aug 02, 2015
Publication Time: 0.37 months
Copyright Information
© SISEF - The Italian Society of Silviculture and Forest Ecology 2014
Open Access
This article is distributed under the terms of the Creative Commons Attribution-Non Commercial 4.0 International (https://creativecommons.org/licenses/by-nc/4.0/), which permits unrestricted use, distribution, and reproduction in any medium, provided you give appropriate credit to the original author(s) and the source, provide a link to the Creative Commons license, and indicate if changes were made.
Web Metrics
Breakdown by View Type
Article Usage
Total Article Views: 51889
(from publication date up to now)
Breakdown by View Type
HTML Page Views: 43999
Abstract Page Views: 3236
PDF Downloads: 3478
Citation/Reference Downloads: 29
XML Downloads: 1147
Web Metrics
Days since publication: 3812
Overall contacts: 51889
Avg. contacts per week: 95.28
Article Citations
Article citations are based on data periodically collected from the Clarivate Web of Science web site
(last update: Mar 2025)
Total number of cites (since 2015): 4
Average cites per year: 0.36
Publication Metrics
by Dimensions ©
Articles citing this article
List of the papers citing this article based on CrossRef Cited-by.
Related Contents
iForest Similar Articles
Research Articles
Combined pre-hardening and fall fertilization facilitates N storage and field performance of Pinus tabulaeformis seedlings
vol. 9, pp. 483-489 (online: 07 January 2016)
Research Articles
Conservation of Betula oycoviensis, an endangered rare taxon, using vegetative propagation methods
vol. 13, pp. 107-113 (online: 23 March 2020)
Research Articles
Substrates and nutrient addition rates affect morphology and physiology of Pinus leiophylla seedlings in the nursery stage
vol. 10, pp. 115-120 (online: 02 October 2016)
Research Articles
Germination and seedling growth of holm oak (Quercus ilex L.): effects of provenance, temperature, and radicle pruning
vol. 7, pp. 103-109 (online: 18 December 2013)
Research Articles
Linking nursery nutritional status and water availability post-planting under intense summer drought: the case of a South American Mediterranean tree species
vol. 9, pp. 758-765 (online: 03 June 2016)
Research Articles
Forecasting the field performance of Austrian pine seedlings using morphological attributes
vol. 10, pp. 99-107 (online: 13 October 2016)
Research Articles
Use of alternative containers for promoting deep rooting of native forest species used for dryland restoration: the case of Acacia caven
vol. 10, pp. 776-782 (online: 02 September 2017)
Research Articles
Controlled-release fertilizers combined with Pseudomonas fluorescens rhizobacteria inoculum improve growth in Pinus halepensis seedlings
vol. 8, pp. 12-18 (online: 12 May 2014)
Research Articles
Seedling quality and short-term field performance of three Amazonian forest species as affected by site conditions
vol. 17, pp. 80-89 (online: 21 March 2024)
Short Communications
Effect of four levels of shade on survival, morphology and chlorophyll fluorescence of Nothofagus alessandrii container-grown seedlings
vol. 8, pp. 638-641 (online: 08 January 2015)
iForest Database Search
Search By Author
Search By Keyword
Google Scholar Search
Citing Articles
Search By Author
Search By Keywords
PubMed Search
Search By Author
Search By Keyword