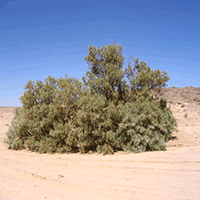
Effect of salt and drought on growth, physiological and biochemical responses of two Tamarix species
iForest - Biogeosciences and Forestry, Volume 8, Issue 6, Pages 772-779 (2015)
doi: https://doi.org/10.3832/ifor1233-007
Published: Mar 25, 2015 - Copyright © 2015 SISEF
Research Articles
Abstract
Tamarix trees are considered of particular interest for afforestation and reforestation of degraded areas prone to salinity and drought. In this study, a comparison of the performance and physiological responses of two species of Tamarix grown in saline and dried soils was carried out. Stem cuttings of T. aphylla and T. jordanis were collected from a location in the Negev desert and the plantlets obtained were subjected to four different soil treatments under semi-controlled conditions for 14 days. The treatments were: fresh water (C); salt (S: 150 mM of NaCl); drought (D: 50% field capacity); and a combined stress (DS: 150 mM of NaCl + 50% FC). Results showed a higher tolerance to salt stress of T. jordanis as compared with T. aphylla. The maintenance of high amount of cell carbohydrates, the high capacity of carbon assimilation and the active growth were considered as markers of salt tolerance in Tamarix spp. T. aphylla showed better performances in terms of growth and biomass production than T. jordanis in dry conditions. The high accumulation of sugars found in the leaves of T. aphylla under mild drought is considered a mechanism of acclimatization. The combined stress (salt+drought) lowered the performance of plants as compared to salt and drought stress applied alone. The possible role of the accumulation of proline observed in the leaves of both species under stressful conditions is discussed.
Keywords
Introduction
Afforestation and reforestation activities have recently received high attention as measures for carbon sequestration and mitigation of climate change ([49]). However, such activities may be complex in degraded lands where soil exhibit harsh conditions due to water shortage and salt accumulation, especially in vulnerable Mediterranean areas. The co-occurrence of the decrease in precipitation, the salinization of groundwater, and the overpopulation compelled many Mediterranean countries to look out for additional water resources (reclaimed waste water, desalinized water, etc.) in order to meet the growing demand and maintain high productivity of plants. Therefore, to face the problem of land degradation, there are increasing prospects for the use of salt and drought tolerant species showing high carbon sequestration rate and requiring minimal management practices and low production costs.
Tamarix is currently regarded as one of the species suitable to combat land degradation and mitigate climate change. This genus consists of halophytic shrubs and trees native to an area spanning from southern Europe and North Africa through the Middle East and south Asia to China and Japan ([39]). Tamarix plants are of particular interest for their fast growth, easy vegetative propagation and acclimatability to a wide range of contrasting environmental conditions ([6]). Tamarix shows various protective mechanisms allowing its survival and growth in harsh environments, including the presence of salt glands on leaves ([46], [31], [30]), which play an important role in regulating ionic balance ([38]) and maintaining/stabilizing osmotic and turgor pressure under high salinity ([13]). Indeed, such glands may excrete the salt excess which accumulates in the tissue through transpiration ([40], [41]). Previous studies have also investigated the biochemical responses of Tamarix spp. under different levels of salt stress ([42], [22], [23]). Tamarix jordanis Boiss has been reported to contain proline analogues that can reduce the negative effects of NaCl on the RuBisCO activity ([42]). In addition, proline has been reported to accumulate in roots of Tamarix tetragyna Ehrenb with increasing salinity up to 480 mM ([3]).
The ability of Tamarix to closely regulate photosynthesis and leaf conductance during drought increases its survivability and competitiveness in arid and semiarid rangelands ([34]). According to Frasier & Johnsen ([15]), Tamarix spp. are well adapted for survival in arid and semi-arid climates, and may tolerate even dramatic changes in soil moisture after establishment, provided that groundwater is available. Tamarix has a deep, extensive root system very efficient in accessing limited water supplies, and has higher water-use efficiency than native riparian ([51]). While the role of metabolites such as soluble sugars (sucrose, glucose and fructose) in conferring drought tolerance is well documented for many plants ([9], [44], [18]), little is known about the accumulation of osmolytes and their role in drought tolerance of Tamarix spp.
Approximately 54 species of Tamarix spp. have been described worldwide ([5]), which may differ in their tolerance to salt and drought stress. However, few studies compared their performance under different harsh conditions. Thus, understanding how Tamarix spp. respond to the combined effect of drought and salt stresses may contribute to the selection of the best material to be used for biomass production and conservation purposes in arid zones. This study aims to evaluate the effects of salinity, drought stress and their combination on growth rate, biomass and gas exchange of two Tamarix species, as well as on their carbohydrate and proline content.
Material and methods
Plant material and culture conditions
Stem cuttings of two species of Tamarix, T. aphylla (L.) Karst and T. jordanis Boiss, were collected from a plantation located in the Negev Desert (southern Israel - 31° 30′ 56.01″ N, 34° 26′ 59.62″ E). Average annual rainfall at the sampling site was less than 100 mm yr-1 and summer average temperature between 32 and 39 °C. One year old stem cuttings (diameter: 7-9 mm, length: 20 cm) were planted in pots of 24 cm in diameter with drain holes filled with sandy soil. After rooting of cuttings, plants showing homogeneous development and size were selected, transplanted and acclimatized in a greenhouse for 14 days. Each species was then submitted to four different treatments, each including seven replicates, totaling 112 plants (28 individuals per species per treatment). The first treatment was irrigated with fresh water (C), the second with saline water 150 mM (S), the third (D) was irrigated to 50% of field capacity (FC), and the fourth with saline water 150 mM to 50% FC (DS). Pots were covered with plastic film to minimize evaporation. Four pots with no plants were used as a control to monitor the evaporative loss from the soil surface. Plants were then grown in a greenhouse for 14 days. All the plants received periodically the same quantity of nutrients. Water content was monitored gravimetrically every night by weighing the pots, and water level (measured as weight) was adjusted relative to the FC level needed. Electrical conductivity (EC) of the drained liquid phase was measured daily in the morning after irrigation, using a conductimeter (HI9811, Hanna instruments INC, USA) equipped with an electrode probe (HI1285, Hanna instruments INC, USA). Salt concentration in the soil was assessed by collecting a soil core at 20 cm depth, and then measuring the electrical conductivity of the saturated soil extract ECe. Electrical conductivity was expressed as ds m-1, ranging for both species from 0.9 to 1 ds m-1 for treatment C, 1-1.33 ds m-1 for treatment D 14.8-15.6 ds m-1 for treatment S and 15-18 ds m-1 for treatment DS.
Growth measurements
Plant growth was monitored at the beginning, at the midpoint and at the end of the experiment by measuring the increment in plant height. At the end of the experiment, plants were divided into leaves, stems and roots and their respective fresh weight (FW) was determined. Dry weight (DW) was obtained by oven drying at 70 °C until a constant weight was reached.
Gas exchange measurements
Net assimilation of CO2 (A
), stomatal conductance (g
s) and transpiration rate (E
) were measured at the beginning, midway and at the end of the experiment using a portable photosynthesis system (LI-6400) equipped with a conifer chamber. The intrinsic water use efficiency (WUE
) was obtained as the ratio between net assimilation and stomatal conductance (A
/g
s).
All measurements were made in the morning from 9:30 to 13:00 h, on a green twig of about 5 cm2 selected in the middle part of the plant. Measurement conditions were as follows: 1800 µmol m-2 s-1 photosynthetically active radiation (PAR), 400 µmol mol-1 ambient CO2 concentration, and 28 ± 2 °C leaf temperature. Data were automatically recorded after stabilization of the photosynthesis rate.
Carbohydrates analysis
Fresh leaf tissue was finely ground using liquid nitrogen, and 40 mg of ground tissue were extracted with 1.5 ml of 80% ethanol and 20% buffer (Hepes 100 mM, pH 7.1, MgCl2 10 mM) at 80 °C for 45 min. After cooling and centrifugation at 13 000 g for 5 min, the supernatant was collected for quantification of soluble sugars, while the pellet was used for the determination of the starch content. Soluble sugars were measured using enzymatic assays as described by Moscatello et al. ([33]). Sugar determination assays were performed using an Anthos plate reader (Anthos Labtec Instruments, Wals, Austria) in dual-wavelength mode (340-405 nm). The endpoint determination for all sugars (glucose, fructose and sucrose) was based on the reduction of NAD+ to NADH. Glucose was determined by first converting to glucose-6-phosphate in the presence of 100 mM Hepes (KOH - pH 7.1), 10 mM MgCl2, 1 mM DTT, 0.02% BSA, ATP 100 mM, NAD+ 80 mM and hexokinase 0.5 U, and then measuring its reduction of NAD+ in the presence of glucose-6-phosphate dehydrogenase 0.3 U. Absorbance by NADH was read at 340 nm. Fructose was phosphorylated as for glucose, converted to glucose-6-phosphate with phosphoglucoisomerase 0.3 U, and then assayed as for glucose. Sucrose was hydrolyzed with invertase 50 U, and glucose and fructose released by hydrolysis were assayed as described above. The pellet was washed three times with sodium acetate 50 mM pH 4.6, autoclaved with 1 ml sodium acetate for one hour at 120 °C, and then hydrolyzed by adding 70 U amyloglucosidase and 4 U α-amylase. The obtained suspension was incubated at 50 °C for one hour with regular shaking. Following centrifugation at 13 000 g, the glucose released in the supernatant was used for starch quantification as in the aforementioned assay.
Proline analysis
Free proline content was estimated following the method of Bates ([4]). Fresh leaves (0.5 g) were extracted in 3% sulphosalicylic acid, and the homogenates obtained were centrifuged at 10 000 g for 10 min. A 2 ml of the supernatant was reacted with 2 ml of acid ninhydrin reagent and 2 ml of glacial acetic acid in a test tube for one hour at 100 °C and the reaction was terminated in an ice bath. The reaction mixture was extracted with 4 ml of toluene and mixed vigorously with a vortex mixture for 15-20 s. The chromophore containing toluene was aspirated from the aqueous phase, warmed to room temperature and the absorbance measured at 510 nm using toluene as blank. Proline concentration was calculated from a standard curve using 0-100 µg l-1 proline (Sigma®).
Greenhouse conditions
Temperature and humidity inside the greenhouse were monitored daily throughout the period of the experiment. The mean temperature ranged between 22.02 and 27.32 °C, while mean relative humidity ranged between 37.8 and 73.9%. The observed lowest values of relative humidity were attained only around the 7th day of the experiment (37.8%).
Experimental design and statistical analysis
A completely randomized design was used. Due to destructive nature of the analyses carried out, seven replicates of plant height increment data and four replicates for all other measurements were used. Means were submitted to the analysis of variance and tested using a least significant difference (LSD) test (α = 0.05). When a significant difference (P ≤ 0.05) was detected, mean values were compared using the Duncan’s multiple range test. Factorial analysis was used to test for inter-specific differences induced by the treatment, and repeated measures ANOVA to test for the time effect. All statistical analyses and computations were carried out using the software package STATISTICA® for Windows version 7.0.61 (StatSoft, Tulsa, OK, USA).
Results
Growth measurements
At the end of the experiment, T. aphylla and T. jordanis showed different growth responses depending the applied stress. Salt had a highly negative effect on plant height increment of T. aphylla, which was reduced by 27.3%, while it did not show any significant effect on T. jordanis (Tab. 1). The shoot dry weight of both species was not negatively affected by salt stress in the short period of the experiment, yet shoot dry weight of T. jordanis under salt stress was even higher than the control (Tab. 1). Similarly, roots of both species were not affected by salt stress.
Tab. 1 - Height increment, shoot, root weight and root/shoot ratio of T. aphylla and T. jordanis plants exposed to stress. Treatments: (C) Control; (S) salt (150 mM); (D) drought (50% FC); (DS) drought + salt (150 mM + 50% FC). Different letters indicate statistically different means (P ≤ 0.05) at the 14th day after the Duncan’s multiple range test. Data of plant height increment are means of seven replicates ± standard error. Data of shoots dry mass, root dry mass and root/shoot ratio are means of four replicates ± standard error.
Species | Treatment | Plant height increment (cm/14 days) |
Shoots dry mass (g plant-1) |
Root dry mass (g plant-1) |
Root/shoot ratio |
---|---|---|---|---|---|
T. aphylla | C | 0.73 ± 0.06a | 41.45 ± 4.37a | 20.37 ± 2.55 a | 0.49 ± 0.02b |
S | 0.53 ± 0.05b | 42.95 ± 5.62a | 21.38 ± 2.35a | 0.50 ± 0.03b | |
D | 0.52 ± 0.05b | 33.65 ± 2.94ab | 20.92 ± 1.50a | 0.63 ± 0.03ab | |
DS | 0.11 ± 0.01c | 26.84 ± 4.45b | 21.06 ± 3.68a | 0.85 ± 0.17a | |
T. jordanis | C | 1.10 ± 0.16a | 66.00 ± 7.74a | 30.19 ± 2.13a | 0.47 ± 0.04b |
S | 1.12 ± 0.25a | 77.10 ± 2.46a | 29.20 ± 4.47a | 0.45 ± 0.05b | |
D | 0.49 ± 0.08b | 44.49 ± 4.57b | 35.85 ± 1.22a | 0.84 ± 0.10a | |
DS | 0.26 ± 0.05b | 44.96 ± 4.78b | 26.98 ± 1.35a | 0.61 ± 0.05b |
Contrastingly, drought (50% FC) showed an opposite impact on Tamarix spp., determining a severe reduction of plant height increment by 55% in T. jordanis vs. only 28.7% in T. aphylla. Reduction of shoot dry weight observed in the drought treatment (50% FC) was also severe for T. jordanis, (32.5%), and non significant for T. aphylla (19% - Tab. 1). Consequently, a higher root/ shoot ratio was recorded in T. jordanis, while non-significant changes of root/shoot ratio were detected in T. aphylla (Tab. 1).
The combination of both stress (treatment DS) had the most pronounced effect on plant growth of both species. On the third day of experiment, symptoms of leaf yellowness and shedding were detected at the bottom part of plants and were more pronounced for T. aphylla than for T. jordanis. At the end of experiment, height increment of T. aphylla and T. jordanis plants was strongly influenced by the combined stress, with a significant decrease by 85% and 76.3%, respectively, as compared with control plants (Tab. 1). In the combined stress treatment (DS), shoot dry weight of T. aphylla and T. jordanis plants were significantly lower than control plants by 35.4% and 31.8%, respectively. Root dry weight was not affected by the combined stress in both species (Tab. 1). The root/ shoot ratio of T. aphylla plants grown under the combined stress was significantly higher (73.5%) than that of control plants, while no significant differences were observed in root/ shoot ratio between T. jordanis and control plants.
Gas exchange measurements
Absolute differences in the mean of carbon assimilation capacity (A
), stomatal conductance (g
s), and transpiration rate (E
) were observed between T. aphylla and T. jordanis plants. Generally, the highest values of A
, g
s and E
throughout the experiment were recorded for T. aphylla, with values higher by 21%, 43.7% and 25.2%, respectively, as compared with T. jordanis plants (Fig. 1). Salinity (S) and the combination of drought and salt stress (DS) did affect A
, g
s and E
in both species to a higher extent than drought alone (D).
Fig. 1 - Gas exchange measurements of T. aphylla (A, C, E) and T. jordanis (B, D, F) plants exposed to different stress treatments. (A
): assimilation rate (panels A-B); (g
s): stomatal conductance (panels C-D); (E
): transpiration rate (panels E-F); (C): Control; (S): salt (150 mM); (D): drought (50% FC); (DS): drought + salt (150 mM +50% FC). Data are means of four replicates. Different letters at the top of bars indicate statistically different means (P ≤ 0.05) after the Duncan’s multiple range test.
On the 2nd day, salt (150 mM) reduced significantly A
, g
s and E
relative to the control (p ≤ 0.05) by 46.9%, 70.3%, 55% in T. aphylla, and by 51.1%, 62.6%, 50% in T. jordanis, respectively. Afterwards, both species showed an improvement of such parameters on the 7th day and a full restoration on the 14th day as compared with untreated plants. However, a better improvement of these parameters was achieved by T. jordanis plants. Indeed, reductions of A
, g
s and E
in T. jordanis plants were no longer significantly different from controls on day 14, while T. aphylla plants showed a significant reduction of A
, gs and E
(by 14.7%, 36%, 23.3%, respectively - Fig. 1).
Drought (50% FC) effects on A
, gs and E
were minimal over the experiment in both species, as compared with salt stress. Only on the 7th day, water deficit (50% FC) determined some alterations of g
s and E
(Fig. 1C-F), while had no significant effect on A
(Fig. 1A-B).
Plants subjected to the combined salt and drought stress (DS) had the most affected values of gas exchange among all treatments and throughout the 14 days of the experiment (Fig. 1). Unlike plants exposed to the salt stress alone (treatment S), both species grown under the combined stress (DS) did not achieve any significant improvement during the experiment. At the end of the experiment, A
was significantly lower than control in T. aphylla (59.8%) and T. jordanis (43.7%, p ≤ 0.05 - Fig. 1A-B).
Water use efficiency
Factorial analysis revealed that the intrinsic water use efficiency (WUE = A/
gs) was generally higher in T. jordanis than in T. aphylla, regardless of the stress applied (p ≤ 0.05 - Fig. 2), due to its relatively low values of g
s (Fig. 1C-D). Overall, a certain increase in the ratio A
/g
s was recorded in all treatments for both Tamarix species, as compared with the control plants.
Fig. 2 - Water use efficiency (A
/g
s) of T. aphylla and T. jordanis plants under different stress treatments. (C): Control; (S): salt (150 mM); (D): drought (50% FC); (DS): drought + salt (150 mM + 50% FC). Data are means of four replicates. Different letters on the top of the error bars indicate statistically different means (P ≤ 0.05) after the Duncan’s multiple range test.
As for the salt stress treatment (S), WUE
increased during the experiment in both species. T. aphylla plants showed a significant increase (44.15%) in WUE
since the second day, while in T. jordanis WUE
increased only at the end of the experiment (Fig. 2).
Under drought stress (treatment D), T. aphylla plants showed some fluctuations of WUE
over the experiment, with an observed increase of 31.3%, while WUE
values in T. jordanis increased only in the last experimental stage (Fig. 2). Contrastingly, both species had the highest WUE
values in the combined treatment (Fig. 2), with higher increments in T. aphylla than in T. jordanis over the trial period (p ≤ 0.05).
Sugars
Leaf sugar content was affected by stress in both species, showing similar sugar trend responses with slight differences and a marked response in T. aphylla.
Under salt stress (S), a drastic reduction of glucose (51%) and fructose (41.5%) content was detected on the 2nd day in T. aphylla plants. Afterwards, glucose and fructose content recovered in the leaves of T. aphylla on the 7th day. Nonetheless, at the end of the experiment the content of both sugars resulted significantly lower in treated plants than in control plants. On the other hand, from the 2nd to the 14th day, the reduction in content of glucose and fructose was not significant for T. jordanis plants subjected to salt stress (150 mM - Fig. 3A-D).
Fig. 3 - Soluble sugar content (glucose: A-B, fructose: C-D; and sucrose: E-F) of T. aphylla and T. jordanis plants exposed to different stress treatments. (C): Control; (S): salt (150 mM); (D): drought (50% FC); (DS): drought + salt (150 mM + 50% FC). Data are means of four replicates. Different letters on the top of bars indicate statistically different means (P ≤ 0.05) after the Duncan’s multiple range test.
Salt stress treatment (S) did not affect sucrose content in both species (Fig. 3E-F), while caused a decline of starch content in both species. In T. aphylla, starch was highly affected by salt stress, decreasing sharply by 49.7% on the 2nd day, while T. jordanis showed a non significant reduction of starch content. However, a recovery in starch content in T. aphylla and T. jordanis was noticed in the following days (Fig. 4).
Fig. 4 - Starch content of T. aphylla and T. jordanis plants exposed to different stress treatments. (C): Control; (S): salt (150 mM); (D): drought (50% FC); (DS): drought + salt (150 mM + 50% FC). Data are means of four replicates. Different letters on the top of bars indicate statistically different means (P ≤ 0.05) after the Duncan’s multiple range test.
Under drought stress (treatment D), the substantial difference in soluble sugar content was evident only at the 7th day. Accumulation of all soluble sugars (glucose, fructose and sucrose) was significantly higher than the control only for T. aphylla leaves (Fig. 3). Drought (50% FC) provoked an initial significant decrease in starch content of T. jordanis leaves and did not influence starch level in T. aphylla leaves. However, a lower starch content was detected on the 7th day in both species (37% in T. aphylla and 44% in T. jordanis) as compared with control plants, partly balanced by an increase in soluble sugars in the leaves of both species. Afterwards, starch content fairly increased in both species under drought (D), equaling the control plant values (Fig. 4).
Combined stress (DS) initially induced a reduction in glucose and fructose in both species. Glucose was reduced by 56% in T. aphylla and by 46% in T. jordanis, while fructose was reduced by 44% in T. aphylla and by 31% in T. jordanis, as compared with the control plants (Fig. 3A-D). At the end of treatment, the combined stress treatment (DS) showed the same effect of the salt treatment (S) on glucose and fructose (Fig. 3A-D). Unlike hexose sugars, sucrose was stable throughout the whole experiment, being values for all treated plants not significantly different from those of the control plants (Fig. 3E-F). The combined stress significantly reduced the starch content relative to the control in both species, with an estimated reduction at the 2nd day between 49.7% and 61.3% in T. jordanis and T. aphylla, respectively. The last day of experiment, starch content of plants grown under combined stress (DS) was significantly lower than the control 64.2%, 74.2% in T. jordanis and T. aphylla, respectively (Fig. 4). The starch response under the combined stress was similar to that described for the salt stress treatment at the beginning, though at the end of the experiment clear differences from S and D treatments were observed (Fig. 4).
Proline
In general, T. aphylla accumulated larger quantity of proline than T. jordanis. Salt stress induced an accumulation of proline in the second day only in T. aphylla. Neither species showed any significant response in proline accumulation under water deficit. For both species proline accumulation was higher for plants under treatment DS (combined stress), with the free proline content increasing approximately 5-9 fold as compared with the control plants (Fig. 5).
Fig. 5 - Proline content of T. aphylla and T. jordanis plants exposed to different stress treatments. (C): Control; (S): salt (150 mM); (D): drought (50% FC); (DS): drought + salt (150 mM + 50% FC). Data are means of four replicates. Different letters on the top of bars indicate statistically different means (P ≤ 0.05) after the Duncan’s multiple range test.
Discussion
Tamarix plants are halophytes that can acclimate and grow under high salinity levels, surviving in areas where the groundwater concentration of dissolved solids reaches 15 000 ppm ([46], [7]). This tolerance to saline soil is achieved mainly by special salt excreting glands ([45], [26]).
In this study, submission of two different Tamarix species to salt stress (150 mM) induced notable changes on physiological responses and growth of both species relative to plants grown under control conditions. The yellowing and shedding of old leaves observed in both species during the experiment suggest that toxic ions could accumulate in the oldest leaves, preventing the death of younger ones. Such symptoms appeared earlier and more severely in T. aphylla, indicating its lower ability in partitioning toxic ions in the vacuoles, and therefore a lower salt tolerance as compared with T. jordanis. Storage of salt in old leaves probably occurs via the absorption of incoming ions from the xylem or retranslocation through the phloem from young to older leaves. This mechanism has been reported in many halophytes plants, and limit the transportation of salt to active younger leaves and reduce their toxic effect on such organs. The shedding of old leaves may then eliminate the excess of salt ([32], [2]). Likely, the initial decrement of gas exchange measures observed in this study for both species was generated by the salt osmotic stress, and was followed by a better acclimation and performances of T. jordanis than T. aphylla. The greater reduction of g
s observed under salt stress in T. aphylla (Fig. 1C-D) is consistent with the findings of Abbruzzese ([1]), who reported a higher control of stomatal conductance in T. aphylla under salt stress. Differences in the recovery of stomatal opening may also account for the higher salt tolerance observed in T. jordanis as compared with T. aphylla. Stomata closure in the latter species may contribute to limit the damage under salt stress, thus reducing the assimilation rate, which increased the intrinsic water use efficiency (A
/g
s), thereby maintaining a high biomass in the short period (Tab. 1). Contrastingly, T. jordanis showed a higher water use efficiency in absolute term.
The remarkable reduction of hexoses and starch in the leaves of T. aphylla under salt stress on the 2nd day (Fig. 3A-C, Fig. 4) was associated with a decrease in CO2 assimilation, though the recover of the starch content to the same level of control plants was observed afterward. This could be related to both a partial recover of photosynthesis and a reduction of plant sinks demands, slowing down the plant height increment (Tab. 1), and decreasing sugar export according to the source/sink equilibrium under salt stress (150 mM). The observed reduction of sugar content in T. aphylla confirm the results of Parida et al. ([37]) who reported that salinity decreased soluble sugars in the salt tolerant mangrove Aegiceras corniculatum L., as well as those of Gadallah ([17]) who observed a reduction of soluble sugars in Vicia faba L. On the contrary, the content of sugars in the leaves of T. jordanis was not significantly reduced under salt stress (Fig. 3B-D, Fig. 4). The maintenance of sugars in T. jordanis and the higher content of starch under control and salty conditions may have a protective role on membranes. Carbohydrates such as soluble sugars (glucose, fructose, sucrose) affects osmoregulation, osmoprotection, carbon storage, and radical scavenging ([36]). Consistently with many other halophytes species ([14]), T. jordanis growth was stimulated by salinity, while T. aphylla showed a growth reduction in the same experimental conditions (Tab. 1). Accordingly, Waisel ([45]) reported a reduced growth of saplings of T. aphylla under irrigation with 0.1M and 0.2 M of NaCl. Such growth depression is probably due to the osmotic and toxic effect of the NaCl solution ([45]), which accumulated at higher concentration in T. aphylla than in T. jordanis ([1]). Growth was also possibly reduced as a consequence of the increase in cost energy associated with salt pumping, increased respiration ([24]) and decreased photosynthetic rates ([20]).
In contrast to salt, drought (50% FC) never induced appreciable effects on carbon assimilation in any of the studied species in the short time (Fig. 1). The maintenance of optimal levels of photosynthesis under drought conditions may be related to the high efficiency of Tamarix twigs to excrete calcium carbonate in non-saline soils, thereby trapping the CO2 from the atmosphere. According to Waisel ([47]), a leaf chalk crust may have a conspicuous adaptive value in terms of optimization of photosynthesis by concentrating the atmospheric CO2. Despite its small surface area, T. aphylla showed a higher capacity of assimilation under control and drought stress (Fig. 1A-B). T. aphylla excrete more calcium than T. jordanis ([1]), therefore it may benefit from an extra source of carbon trapped by calcium, and exploit it for optimal assimilation during early morning ([47]).
In this study, the stomatal conductance of plants grown under drought conditions was substantially maintained in both species over the whole experiment, except for day 7 (Fig. 1C-D), when an increase in soluble sugars and a concomitant decrease of relative humidity (37.9%) was observed (Fig. 3A-F). Despite the short duration of the experiment carried out, the high content of soluble sugars and their increase mainly in T. aphylla suggest that soluble sugars accumulation is one of the possible mechanisms adopted by this species as a response to drought. Accumulation of soluble carbohydrates in leaves is common under water stress ([8]). Moreover, it has been reported that plants growing in dry habitats contain more soluble sugars than those living in moist habitats. lljin ([19]) reported values of sugar content (on a dry weight basis) ranging from 1.3% in herbaceous mesophytes to 6.9% in xerophytic trees and shrubs. In this study, an increase of sucrose and a simultaneous decrease of starch content in plants grown under drought stress was detected on the 7th day of the experiment (Fig. 3E-F, Fig. 4). It is also a common observation that water stress conditions induce a severe decrease in starch and simultaneously an accumulation of soluble sugars ([19], [43]). Such a shift in carbon partitioning may have an adaptive value, since it could contribute to osmotic adjustment and protection ([10], [28]).
Despite the limited effect of drought on photosynthesis, growth of T. jordanis plants was severely limited, with height increment lower by 55% than that of control plants. This reduced growth may be due to other factors not analyzed in this study, such as hormones or precursors ([16], [35]). Contrastingly, the slight effect of drought on height increment and biomass of T. aphylla plants was probably due to the high and early increase of intrinsic water use efficiency in this species, allowing plants under stress conditions to produce a quantity of dry matter similar to control plants with less water consumption. Also, the increase in the root/shoot ratio observed in T. jordanis under drought conditions was probably due to a significant decrease in the shoot biomass (Tab. 1), that is considered an adaptive mechanism allowing to reduce the transpiration surface and to increase the water absorption from the soil ([21]).
Controversial results are reported in the literature about the combined effect of salt and drought stress. In this study, the presence of both constraints in the soil (salt 150 mM + drought 50% FC) showed the most severe impact on both species, as compared with water and salt stress applied separately. Symptoms of yellowing and defoliation in plants under such combined stress were much more evident than in leaves of plants grown under salt alone, appearing on the third day of the experiment. Such symptoms suggest a high accumulation of salt in older tissues to prevent the death of young leaves. Adverse effects on all measured parameters were reported. Photosynthesis, transpiration, stomatal conductance, sugars and starch content were significantly reduced in response to the combined stress. The combination of drought and salt stress induced effects similar to salt stress on the reduction of hexoses sugars. In addition, both species showed a marked decline of the starch content, which was lower than that of salt and drought applied alone. This confirms the positive interaction between salt and drought in the reduction of the starch content in the species analyzed. However, the ability of plants of both species to survive the whole experiment may be associated with a high production of proline content and with an increase of their water use efficiency. Proline may act as an osmoregulator, a ROS (reactive oxygen species) scavenger and a molecule chaperone reinforcing the structure of proteins, thereby protecting plant cells from damage caused by stress ([11], [27]). Moreover, it has been suggested that proline may represent a stock of nitrogen available for the plant after a period of suffering from water deficiency ([12]). In this study, a high proline content was observed in the two Tamarix species grown under the combined stress (Fig. 5). Likely, proline accumulation was induced by NaCl ion stress rather than drought, because plants grown under salt stress alone also showed an initial increase in proline content (Fig. 5). However, proline usually accumulates in the cytosol, which is only 5 to 10% of the cell volume ([29], [50]). Based on our results, the contribution of proline to the cell osmotic potential (0.028-0.031 MPa at the 7th day) was too low for the osmotic adjustement of the cytosol (0.2-0.4 MPa in osmotic potential). Therefore, we may hypothesize that proline acts mainly as osmoprotector and storage of nitrogen and carbon for future use under stressful conditions. These considerations are supported by Wikqiang et al. ([48]) and Koyro ([25]), who found that proline contributed to the protection of T. chinensis against high salinity in the soil by scavenging the ROS generated by salt stress, which may cause oxidative damage to membrane lipids, proteins and nucleic acids. Furthermore, the accumulation of proline was suggested to be related to a decrease or suppression of gene encoding for the proline dehydrogenase (PDH), or due to the expression of gene P5CS for the key enzyme regulating the proline synthesis via glutamate pathway under environmental stresses ([27]).
In this study, the severe reduction of plant growth observed in both Tamarix species grown under high salinity and drought could be attributed to more than one factor: hindrance in cell division and cell elongation, reduction in assimilation rate and increase in ion toxicity due to reduction of water availability. All the above factors may retard plant elongation, but also hinder dry matter accumulation causing a decrease in biomass production.
Conclusion
The two species analyzed in this study, Tamarix aphylla and T. jordanis, proved their ability to cope with salt and drought stress to a different extent, through common acclimatization mechanisms. T. aphylla showed better performances and growth than T. jordanis under drought stress, due to higher photosynthesis, sugar accumulation and water use efficiency. The maintenance of an adequate sugar content under salt stress reflects the important role of these osmolytes in cell protection of salt tolerant species like T. jordanis. From an agronomic and environmental perspective, the two species studied seems to acclimatize fairly well to a moderate salinity (150 mM) without any loss of biomass. In particular, T. jordanis seems to maintain a high efficiency in the carbon sequestration over a relatively short period of time. However, a reduction in biomass could be expected at moderate drought (50% FC), particularly in T. jordanis. In soils with high salinity (0.1-0.2 M of NaCl) and in the absence of irrigation, a short drought period might causes a severe damage to these trees.
Acknowledgments
The authors are grateful to the Euro Mediterranean Center for Climate Change (CMCC) and the Italian Ministry of Environmental, Land and Sea (MATTM) for providing financial support. The authors further acknowledge Matilde Tamantini at the University of Tuscia and Giovanni De Simone at the Institute of Agro-Environmental and Forest Biology (IBAF/CNR) for the technical support. We would like to thank also Rindala Hakim and Inaam Darwish for editing the English version of this paper.
References
Gscholar
Gscholar
Online | Gscholar
CrossRef | Gscholar
Gscholar
Gscholar
Gscholar
Gscholar
Gscholar
Authors’ Info
Authors’ Affiliation
Riccardo Valentini
University of Tuscia, Department of Forest Environment and Resources (DISAFRI), v. S. Camillo de Lellis snc, I-01100 Viterbo (Italy).
Consiglio per la Ricerca in Agricoltura e l’Analisi dell’Economia Agraria, Forestry Research Center (CRA-SEL), v.le Santa Margherita 80, I-52100 Arezzo (Italy)
Alberto Battistelli
Institute of Agro-Environmental and Forest Biology (IBAF-CNR), v.le G. Marconi 2, I-05010 Porano, Terni (Italy)
Corresponding author
Paper Info
Citation
Dawalibi V, Monteverdi MC, Moscatello S, Battistelli A, Valentini R (2015). Effect of salt and drought on growth, physiological and biochemical responses of two Tamarix species. iForest 8: 772-779. - doi: 10.3832/ifor1233-007
Academic Editor
Silvano Fares
Paper history
Received: Jan 02, 2014
Accepted: Oct 16, 2014
First online: Mar 25, 2015
Publication Date: Dec 01, 2015
Publication Time: 5.33 months
Copyright Information
© SISEF - The Italian Society of Silviculture and Forest Ecology 2015
Open Access
This article is distributed under the terms of the Creative Commons Attribution-Non Commercial 4.0 International (https://creativecommons.org/licenses/by-nc/4.0/), which permits unrestricted use, distribution, and reproduction in any medium, provided you give appropriate credit to the original author(s) and the source, provide a link to the Creative Commons license, and indicate if changes were made.
Web Metrics
Breakdown by View Type
Article Usage
Total Article Views: 51953
(from publication date up to now)
Breakdown by View Type
HTML Page Views: 44287
Abstract Page Views: 2557
PDF Downloads: 3905
Citation/Reference Downloads: 26
XML Downloads: 1178
Web Metrics
Days since publication: 3541
Overall contacts: 51953
Avg. contacts per week: 102.70
Article Citations
Article citations are based on data periodically collected from the Clarivate Web of Science web site
(last update: Feb 2023)
Total number of cites (since 2015): 5
Average cites per year: 0.56
Publication Metrics
by Dimensions ©
Articles citing this article
List of the papers citing this article based on CrossRef Cited-by.
Related Contents
iForest Similar Articles
Research Articles
Salinity strongly drives the survival, growth, leaf demography, and nutrient partitioning in seedlings of Xylocarpus granatum J. König
vol. 10, pp. 851-856 (online: 26 October 2017)
Research Articles
Links between phenology and ecophysiology in a European beech forest
vol. 8, pp. 438-447 (online: 15 December 2014)
Research Articles
Drought tolerance in cork oak is associated with low leaf stomatal and hydraulic conductances
vol. 11, pp. 728-733 (online: 06 November 2018)
Research Articles
Magnolia grandiflora L. shows better responses to drought than Magnolia × soulangeana in urban environment
vol. 13, pp. 575-583 (online: 07 December 2020)
Research Articles
Assessment of cadmium tolerance and phytoextraction ability in young Populus deltoides L. and Populus × euramericana plants through morpho-anatomical and physiological responses to growth in cadmium enriched soil
vol. 10, pp. 635-644 (online: 01 June 2017)
Research Articles
Variability of tolerance of Wild cherry clones to PEG-induced osmotic stress in vitro
vol. 15, pp. 265-272 (online: 25 July 2022)
Research Articles
Effect of drought stress on some growth, morphological, physiological, and biochemical parameters of two different populations of Quercus brantii
vol. 11, pp. 212-220 (online: 01 March 2018)
Research Articles
Effects of mild drought on the morphology of sun and shade needles in 20-year-old Norway spruce trees
vol. 12, pp. 27-34 (online: 10 January 2019)
Review Papers
Indicators of drought effects in Pinus sylvestris: genetic analyses to corroborate the results of empirical methods
vol. 3, pp. 89-91 (online: 15 July 2010)
Short Communications
Preliminary indications for diverging heat and drought sensitivities in Norway spruce and Scots pine in Central Europe
vol. 13, pp. 89-91 (online: 01 March 2020)
iForest Database Search
Search By Author
Search By Keyword
Google Scholar Search
Citing Articles
Search By Author
Search By Keywords
PubMed Search
Search By Author
Search By Keyword