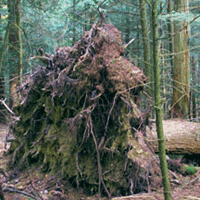
Soil microorganisms at the windthrow plots: the effect of post-disturbance management and the time since disturbance
iForest - Biogeosciences and Forestry, Volume 10, Issue 2, Pages 515-521 (2017)
doi: https://doi.org/10.3832/ifor2304-010
Published: Apr 20, 2017 - Copyright © 2017 SISEF
Research Articles
Abstract
Wind disturbance is a major natural driver of forest dynamics in a large part of Europe and can affect soil properties in different ways and for different time. The present study focuses on the effects of post-disturbance management of windthrow plots in the Tatra Mountains, Slovakia, on soil microorganisms ten years after the disturbance. Their comparison with the microbial characteristics at a new windthrow plot caused by strong wind in 2014 was also carried out. Three research plots differing in the way of their management after the windstorm in 2004 (EXT, salvage plot; FIR, salvage plot affected by fire; NEX, unsalvaged plot) and the plot destroyed by strong wind in May 2014 (REX) were used for study. Ten soil samples were taken from the mineral A-horizon (depth: 0-10 cm) at each plot in autumn 2014. In soil samples, soil chemical and microbial characteristics (microbial biomass C, basal and substrate-induced respiration, N-mineralisation, catalase activity, richness and diversity of soil microbial functional groups based on the Biolog approach) were determined. Ten years after the disturbance we still observed significant differences in microbial characteristics between FIR and the other plots, with higher microbial activity at the FIR, while no significant differences were found among the other plots. The results indicate that at a higher altitude the effect of fire on soil microorganisms is more distinct than removing or not removing of fallen trees and persists even over a decade.
Keywords
Forest Soil, Soil Microorganisms, Windthrow, Fire, Postdisturbance Management
Introduction
Recently, there is evidence of increasing intensity and frequency of extreme climate events such as extreme heat or cold, heavy precipitation or strong wind in most land regions of the world. Due to the ongoing climate change, this increase is expected to continue in the future. Climate change may considerably affect terrestrial ecosystems because of natural disturbances such as flooding, fire, windthrow, bark beetle damage, spread of pathogens etc. Wind disturbance is the main natural driver of forest dynamics in Central and Northern Europe ([33]). Windthrows can affect soil properties and nutrient fluxes of forest ecosystems mainly by removing the canopy and provoking the death of roots ([42]). Trees and forest stands are known to influence soil and its properties in various ways, e.g., through above- and belowground input of organic sources (often differing in their quantity and quality), as well as water and nutrient uptake by plant roots, differences in microclimate, stemflow, pit-and-mound formation after windthrow ([38], [36]). Changes in the herb layer (damage by fallen trees, invasion of non-indigenous species etc.) and regeneration of trees after the disturbance may also modify the soil ([3]). Therefore, canopy loss due to natural disturbances (windthrow) or management (clear-cutting) may distinctly influence site conditions and consequently change soil properties ([29], [11]), mainly in the top soil horizons.
Changes in water and temperature regimes, nutrient leaching, chemical properties and specific processes such as perturbation caused by uprooting, erosion, etc. have been primarily studied up to now ([30], [38], [23]). Changed environmental conditions on the affected areas are expected to result in changed microhabitat conditions for living organisms ([3], [38], [5], [23]) and, consequently, to be reflected in different responses of soil organisms. However, information about soil biota on windthrow-affected areas especially in long-term is scarce ([41], [4], [39]).
In November 2004, over 12.000 ha of forest stands in the Tatra Mountains (Slovakia, Central Europe) were broken or uprooted by strong wind. After this event, monitoring of changes in soil properties started at the long-term research plots with different post-disturbance management (salvage cutting, no interference) established by the Research Station of the Tatra National Park. Ten years later, in May 2014, the forest stand which served as a reference plot until that time, was destroyed by another strong wind event. Windthrow areas are usually salvaged; harvesting of disturbed stands is practiced for the sanitary and economic reasons and to prevent fires whose number increases as a result of extremely hot and dry periods associated with the ongoing climate change. However, in other areas (e.g., protected areas and nature reserves) the fallen trees are retained on site ([24]). As the environmental conditions at plots with different post-disturbance regime differ, changes in plant cover and soil properties are expected. Shortly after the disturbance, changes in microbial biomass and/ or activity are often observed ([37], [27]). Also in the Tatra Mts. we found changes in the soil microbial activity and biomass during the first years after the disturbance ([8]).
After a disturbance, environmental conditions at the windthrow plots are gradually changing with the formation of a new forest through the ongoing plant succession and trees regeneration. Such changes can be reflected in the responses of soil microbiota to varying degrees at the plots. The objective of this study was to assess the responses of soil microorganisms to wind disturbance 10-years after the event and find whether different post-disturbance management regimes on the windthrow area are reflected in different responses of soil microbiota after a decade. At the same time, the newly disturbed forest (a control plot formerly) would add useful information on windthrow effects directly after a disturbance event and on possible differences in soil microbial responses between “new” and “old” windthrow plots. Understanding the microbial processes in the long-term and their responses to different management regimes may help in the choice of appropriate forest practices regarding the potential nutrient availability for later tree growth, since soil microbiota is the key player in nutrient cycles and nutrient accessibility for plants.
Materials and methods
Site description
The study was performed at the research plots established in 2005 in the windthrow area of the Tatra National Park situated at an altitude of 1040-1260 m a.s.l. with SE-S aspect and slope of 5-10%. The dominant soil type is Dystric Cambisol formed mostly from moraine material. Four research plots with area of 100 ha each and with different management regime were surveyed ([7]):
- REX - former reference plot established in an intact forest stand and destroyed by strong wind in May 2014. Canopy was reduced to approx. 15%. At the time of soil sampling, most of fallen trees were not removed from the plot yet. Until the disturbance the plot was covered by a >120-year-old forest stand of Norway spruce (Picea abies Karst., 80%) with an admixture of larch (Larix decidua Mill., 20%) and served as a control plot unaffected by wind. Vaccinium myrtillus, Avenella flexuosa, Oxalis acetosella and several mosses are the most widespread understory plants at this plot. During the last 10 years, the herb layer has not significantly changed.
- EXT - plot with salvage logging, fallen trees were removed from the plot after the windthrow in 2004. At the plot the vegetation composition differs significantly from the former one. Since 2007 Calamagrostis villosa and Chamaenerion angustifolium have become the most common species; the plot was reforested by Picea abies, Pinus cembra, Larix decidua, Fagus sylvatica and Acer pseudoplatanus.
- FIR - fire plot, fallen trees were extracted from the plot; however, in summer 2005 the plot was destroyed by a wildfire and the surface organic layer was mostly burnt. Since 2007 C. angustifolium and Rubus ideaus became dominant and since 2010 also C. villosa and C. arundinacea appeared more frequently; the plot was reforested with the same species as EXT.
- NEX - not extracted, windthrow plot without any intervention, left to spontaneous succession; C. villosa and A. flexuosa dominated the first year after the windstorm, C. angustifolium became dominant in 2011 and R. idaeus in 2012. Intensive natural regeneration of trees (Picea abies, Sorbus aucuparia, Salix caprea and Betula pendula) is ongoing at the plot.
Soil sampling and analyses
In autumn 2014, soil samples were taken in each plot at 10 m intervals along a 90 m long transect from the depth of 0-10 cm (excluding the surface organic layer). Herb layer (cover percentage and number of species) was recorded around the sampling places.
Immediately after bringing samples to the laboratory, each sample was divided into two parts. One part was intended for microbial analyses, as well as moisture and dry mass assessment, and was stored in a refrigerator at 4 °C until the analysis. The other part was air-dried and used for measurements of soil chemical properties.
Moisture and dry weight were determined gravimetrically by oven-drying fresh soil at 105 °C for 24 hours. Soil acidity was measured potentiometrically in 1 M KCl suspension (20 g soil plus 50 ml KCl solution) after 24 h. For the determination of total carbon (C) and nitrogen (N) concentration MACRO Elemental Analyzer® (CNS Version, Elementar Gmbh, Langenselbold, Germany) was used, employing the dry combustion method.
Soil microbial biomass C was estimated according to Islam & Weil ([18]) using the microwave-irradiation procedure. C concentration in the extract was quantified by oxidation with K2Cr2O7/H2SO4 and titrimetrically by (NH4)2Fe(SO4)2. Basal soil respiration was measured by estimating the amount of CO2 released from fresh soil after 24 h incubation at 21 °C and absorbed in 0.05 M NaOH. The amount of carbonate was determined by titration with 0.05 M HCl after the precipitation of carbonates by 0.5 M BaCl2. For the determination of substrate-induced respiration, soil samples were amended by 1% (w/w) glucose and CO2 evolved was measured as described above after 4.5 h ([1]). N-mineralisation was determined by anaerobic incubation according to Kandeler ([19]). Catalase activity was measured 10 min after 3% H2O2 was added to fresh soil sample based on the volume of discharged oxygen, according to the method of Khaziev ([20]). For the study of soil microbial community structure, BIOLOG EcoPlates ([17]) were used. Inocula were prepared by resuspending fresh soil in 0.9% NaCl, the supernatant was diluted 1:10.000, and 150 μl of extract were incubated in microtitration plates at 27 °C for 6 days. Absorbance at 590 nm was recorded every 12 h using the Sunrise Microplate Reader® (Tecan, Salzburg, Austria). Absorbance values were blanked against the control well. Metabolic activity was calculated as the area below the time-absorbance curve, and was used as a measure of abundance of the respective functional group. The richness of the soil microbial community was assessed as the number of substrates with non-zero response. For the estimation of diversity, the Hill’s diversity index (Div) was calculated ([13] - eqn. 1):
where pi is the frequency (relative abundance) of the i-th functional group.
Data evaluation
All results were expressed as dry mass. To account for the effect of the available resources on the microbial community, microbial carbon and basal respiration were also recalculated per unit of total organic carbon (Cmic/C, resp. Resp/C). Metabolic quotient (qCO2) was determined based on the microbial respiration and microbial biomass carbon (Resp/Cmic).
All statistical treatments of data were done using the statistical package SAS/ STAT® ([32]). Differences in soil physico-chemical properties among plots were tested using one-way analysis of variance (proc ANOVA). As biological soil properties may potentially be influenced by soil moisture, covariance analysis was used to test the effects of plot, taking moisture as a continuous covariate (proc GLM). Pairwise differences between plot means were tested by the Tukey-Kramer test.
Because microbial richness and diversity do not completely explain how the composition of functional groups of microorganisms is related to different management, a multivariate analysis was performed. We chose a direct gradient analysis (redundancy analysis - RDA), which allows to determine the environmental variables that best explain the changes of the frequency distributions of microbial functional groups with different management. RDA is based on a linear approximation of the species’ response to environmental gradients (appropriate for a short gradient length) and yields constrained ordination axes reflecting the direction of maximum variability within the dataset which can be explained by the assessed environmental factors ([34]). The significance of environmental variables and RDA axes was tested using Monte Carlo permutation test (9999 runs). Unrestricted permutations were employed, since the abundance of microorganisms and the composition of microbial communities generally change at very short distances, so that we did not expect spatial dependence at the scale used in this study. Significant environmental variables were identified by forward selection. The software package CANOCO ([34]) was used for the calculations.
Results
Ten years after the disturbance, significant differences in some soil properties between plots were still evident (Tab. 1). Among soil physico-chemical properties, distinct differences were found in soil acidity and especially C/N ratio. The REX plot exhibited the highest acidity (pH-KCl 3.19) whereas at the other plots the average values did not drop below pH-KCl 3.49 (Fig. 1). The lowest C/N ratio was found at the EXT plot, while the other plots exhibited higher C/N ratio (average C/N about 19). Neither C nor N concentration differed significantly between plots due to a high intra-plot variability.
Tab. 1 - Analysis of variance and covariance of soil properties (significance of F-tests). (SWC): soil water content; (Cmic): microbial biomass carbon; (Resp): basal respiration; (SIR): substrate-induced respiration; (Nmin): N-mineralisation; (Catal): Catalase activity; (Richn): richness of soil microbial functional groups; (Div): diversity of soil microbial functional groups; (Cmic/C and Resp/C): microbial biomass carbon- and basal respiration-to-total carbon ratio, respectively; (qCO2): metabolic quotient; (df): degrees of freedom; (*): p<0.05; (**): p<0.01; (***): p<0.001; (ns): non-significant (P > 0.05).
Source of variation | df | SWC | pH-KCl | C | N | C/N |
---|---|---|---|---|---|---|
Plot | 3 | ns | * | ns | ns | *** |
Error | 36 | - | - | - | - | - |
Source of variation | df | Cmic | Resp | SIR | Nmin | Catal |
Plot | 3 | ns | ** | ns | *** | ns |
Moisture | 1 | *** | *** | *** | *** | *** |
Error | 35 | - | - | - | - | - |
Source of variation | df | Richn | Div | Cmic/C | Resp/C | qCO2 |
Plot | 3 | *** | ns | ns | * | * |
Moisture | 1 | *** | ns | ns | ns | ns |
Error | 35 | - | - | - | - | - |
Fig. 1 - Comparison of means of the soil reaction (a), carbon (b) and nitrogen (c) concentration, and C/N ratio (d). Homogeneous groups resulting from Tukey-Kramer test are indicated by the same letter. (REX ): plot disturbed by wind in 2014; (EXT): plot disturbed by wind in 2004, salvaged; (FIR): plot disturbed by wind in 2004, salvaged, damaged by fire; (NEX): plot disturbed by wind in 2004, unsalvaged.
The differences in microbial characteristics between plots are not consistent (Tab. 1). Among the measured characteristics, only basal respiration, N-mineralisation and richness of microbial functional groups differed significantly between plots. Generally, the FIR plot exhibited highest microbial activity (Fig. 2 and Fig. 3), while no significant differences were found in microbial activity among the other plots. According to the results of ANOVA, microbial biomass did not differ between plots. However, Tukey-Kramer test indicates differences in Cmic between REX and EXT plots, with higher microbial biomass at the REX plot (Fig. 2). While the diversity of soil microbial functional groups did not differ between plots, the richness of functional groups was significantly lower at the REX plot in comparison to the others (Fig. 3). Among the other microbial indices, qCO2 and Resp/C showed significant differences among different plot management, revealing a higher substrate utilisation at all “old” windthrow plots (Fig. S1 in Supplementary material).
Fig. 2 - Comparison of means of the microbial biomass carbon (a), basal respiration (b), substrate-induced respiration (c) and N-mineralisation (d). Homogeneous groups resulting from Tukey-Kramer test are indicated by the same letter. (REX ): plot disturbed by wind in 2014; (EXT): plot disturbed by wind in 2004, salvaged; (FIR): plot disturbed by wind in 2004, salvaged, damaged by fire; (NEX): plot disturbed by wind in 2004, unsalvaged.
Fig. 3 - Comparison of means of the catalase activity (a), richness (b) and diversity (c) of soil microbial functional groups. Homogeneous groups resulting from Tukey-Kramer test are indicated by the same letter. (REX ): plot disturbed by wind in 2014; (EXT): plot disturbed by wind in 2004, salvaged; (FIR): plot disturbed by wind in 2004, salvaged, damaged by fire; (NEX): plot disturbed by wind in 2004, unsalvaged.
There was neither an unambiguous trend in sample scores of the first two RDA axes, nor a clear association of any management regime with environmental variables (Fig. 4). Nevertheless, RDA scores were distributed according approximately to the naturalness of the plots: most FIR samples (i.e., samples from the most heavily damaged plot) had negative scores on the RDA 1 axis, whereas most REX samples were concentrated in the upper right corner of the graph. The community structure based on the utilisation of different carbon source showed differences especially between the REX and FIR plot. At the FIR plot higher utilisation of L-arginine, L-asparagine, N-acetyl-D-glucosamine, glycyl-L-glutamic acid and putrescine, and lower utilisation of D-glucosaminic acid was observed in comparison to the REX plot (Tab. S1 in Supplementary material).
Fig. 4 - Redundancy analysis of soil microbial data: sample positions. (REX ): plot disturbed by wind in 2014; (EXT): plot disturbed by wind in 2004, salvaged; (FIR): plot disturbed by wind in 2004, salvaged, damaged by fire; (NEX): plot disturbed by wind in 2004, unsalvaged.
Discussion
Analysis of soil data showed that ten years after the disturbance differences in several soil characteristics still persist between plots with different post-disturbance management. However, among soil physico-chemical properties only C/N ratio distinctly differed between plots. Lower C/N ratio occurred at the plots where fallen trees were extracted and thus an intensive succession of plants took place. On the other hand, higher C/N ratio was found at the plots with persisting aboveground tree biomass. Surprisingly, the C/N ratio at the FIR plot did not differ significantly from the REX and NEX plots, despite the fact that tree remains were extracted and similar herb layer succession was ongoing as on the EXT plot.
Environmental change after the disturbance affected soil microorganisms directly and/or indirectly. In our earlier study ([9]), we pointed on the differences in microbial activity at the disturbed plot in comparison to the intact forest one year after the event. FIR plot exhibited the highest microbial activity, due to the reduction in thickness of the surface organic layer, the enrichment of the A-horizon by mineral nutrients released by burning, and the dissolved organic matter from the unburnt remains. Contrastingly, the response of soil microorganisms to different management on EXT and NEX plots generally did not differ, except for slower basal respiration and N-mineralisation on the EXT plot. Later, the responses of soil microbiota to forest disturbances differed as a function of the time since disturbance. As forests recover after the disturbance, post-disturbance changes in soil microbial characteristics can diminish over time. Indeed, a significant positive relationship between time since forest harvesting and microbial biomass has been reported ([14]). Hedo et al. ([12]) found that 17 years after fire there were no differences in microbiological soil properties and enzyme activities of soil between burned and thinned, burned and unthinned, and mature plots, because total vegetation recovery normalises post-fire soil microbial parameters; thus wildfire and post-fire silvicultural treatments are not significant factors affecting soil properties after 17 years. The effect of disturbance on soil microbial communities can persist until aboveground vegetation re-grows ([10]). During the course of vegetation succession, changes in environmental conditions are expected to continue because of gradual changes in plant cover, but their extent and direction are difficult to predict. Rumbaitis Del Rio ([31]) showed that understory vegetation composition and diversity in blowdown areas stabilized during the 5 years following the blowdown as compared with control areas, but vegetation cover continued to increase. Lang et al. ([26]) found that 25 years after the disturbance, tree basal area, sapling density, shrub layer density and seedling cover converged between unsalvaged and salvaged areas; on the other hand, salvaged forest displayed significantly higher herb richness and cover, and a greater dominance of understory species benefiting from disturbance. In this study, ten years after the disturbance, still the FIR plot significantly differed from the others, showing a higher microbial activity. However, unlike the first year after the disturbance ([9]), differences between NEX and FIR seem to vanish as no significant differences were found in microbial characteristics (except N-mineralisation) between these plots. Fire usually leads to a decrease of soil biomass and activity because of the extremely high surface temperature during the burning ([5]), making the conditions for soil microbiota unfavourable. However, the impact of fire on soil microbial biomass and activity is significantly influenced by fire severity, intensity and duration, soil moisture and also soil depth ([5], [15]). It was shown that intense fire with short duration does not affect more than few centimetres below the soil surface. Moreover, burnt aboveground biomass often offers a remarkable increase in the availability of nutrients ([5]); thus their release into the mineral horizons could improve conditions for soil microorganisms, resulting in a higher microbial activity and biomass at the FIR plot and persisting over a decade. Also herbs can play an important role in post-disturbance trend. According to a literature review ([5]), when plants promptly recolonise the burnt area, the pre-fire level of most soil properties can be recovered and even enhanced. At the FIR plot the vegetation cover reached 100% already 2 years after fire, with Chamaerion angustifolium as the dominant species ([16]).
Post-disturbance treatments may cause changes in soil properties. Fleischer ([7]) confirmed temporal changes in soil temperature and moisture on sites with different disturbance regimes. Peterson & Leach ([28]) found that salvaged areas had significantly greater variety of microsites, higher soil temperature and greater canopy openness on comparison to unsalvaged areas. Studies on soil microorganisms at plots cleared and not-cleared after windthrow are scarce ([25]). More studies are related to clear-cut and partially cut plots, which could be roughly compared with post-windthrow treatments. As shown by Holden & Treseder ([14]), while forest clear-cutting had a significant negative impact on soil microorganisms, partial harvesting did not significantly affect soil microbial biomass. Even ten years after the disturbance, we did not confirm any differences in microbial characteristics between the salvaged and unsalvaged plots, in spite of the fact that they differed in the above-ground biomass (dead biomass of fallen trees), herb layer cover and microclimate. Surprisingly, no significant differences were found also between the REX plot (new windthrow plot) on one side and the EXT and NEX plots (old windthrow plots) on the other side. The lack of differences indicate that at higher elevations, on soil with a low pH and a high carbon stock, the influence of mesoclimate on soil microorganisms is more important than the differences in environmental conditions (microclimate, herb layer etc.) caused by different post-windthrow management. As mentioned above, the REX plot served as a control plot until the disturbance in 2014. As the reference (control) plot became itself wind-damaged and no other plot fulfilling the criteria of reference plot was found, it was not possible to evaluate the impact of the “new” and “old” windthrow on soil microorganisms in contrast to intact forest.
Positive as well as negative effects of natural disturbances on belowground diversity were observed depending on the time since disturbance ([5], [2], [4], [39]). Meta-analysis of the disturbance effect on species richness performed by Thom & Seidl ([35]) showed that species richness increases by 35.6% on average for a high-severity disturbance event. At the windthrow plots of the Tatra Mts. only the REX plot differed significantly in the richness of soil microbial functional groups from the others. Community structure based on the utilisation of different carbon sources showed differences especially between the REX and FIR, i.e., the freshly disturbed plot and the most affected “old” plot, respectively. Even though there is no one-to-one relationship between the functional and taxonomical groups of microorganisms, this supports the suggestion that the composition of microbial community is driven by the plant community composition ([40]). Knelman et al. ([21]) highlighted an important role of plant colonization in succession by a clear connection between plant and bacterial communities in a post-fire landscape. Different plant species produce litter and exudates in different quality varying in their degree of stimulation for soil microorganisms ([10]). Since the windthrow, the aboveground input of herbaceous litter with higher N and lower lignin content increased and the deposition of acid spruce needles decreased at the disturbed plots in comparison to the newly formed windthrow plot in the Tatra Mts., which was also reflected in a lower soil C/N ratio. Moreover, the FIR plot was enriched by the ash from the burnt litter in comparison to the other plots, which could differentiate the microbial community structure at this plot from the others. The fact that the shifts in nutrient availability may drive microbial community succession was confirmed by Knelman et al. ([22]). As the aboveground standing plant biomass increases through time after disturbance, changes can be expected until the mature stage of forest is reached. However, information about the changes of soil microbial community structure at post-disturbance plots in long-term is scarce ([6]).
Conclusions
Extreme climate events leading to forest disturbances including windthrow and fire can be expected worldwide as a consequence of ongoing climate change. As they can affect also the environment of soil microorganisms after the disturbance, information about the changes in microbial community and time of its recovery can be very important as the soil microorganisms play the key role in nutrient cycles and their availability for plants. The present study showed that the absence of significant differences in microbial characteristics and a similarity of microbial community composition between the extracted and not-extracted plots persists ten years after the windthrow, despite the fact that a significant difference between plots in C/N ratio appeared as a consequence of the input of the organic matter of different quality. A beneficial effect of the reduction of thick surface organic layers after wildfire and the consequent release of nutrients persists over a decade and is reflected in still higher microbial activity at the burnt plot in comparison to the other windthrow plots. Microbial characteristics at the “new” windthrow plot did not differ from the plots with different management, except the burnt plot. These results suggest that at a high elevation the regional mesoclimate has a deeper effect on soil microorganisms than different post-disturbance management regime (removing or leaving fallen trees), in contrast to a wildfire, whose distinct effect lasts longer than a decade.
Acknowledgments
We thank V. Kriššák, T. Dvorská and Z. Brnáková for technical and laboratory assistance. This study was financed by the projects APVV-15-0176, VEGA 1/0710/17 and VEGA 1/0783/15.
References
Gscholar
Gscholar
Gscholar
Gscholar
Gscholar
Gscholar
Gscholar
Gscholar
Gscholar
Authors’ Info
Authors’ Affiliation
Peter Fleischer
Viliam Pichler
Marián Homolák
Richard Gere
Dušan Gömöry
Technical University in Zvolen, Faculty of Forestry, T. G. Masaryka 24, SK-960 53 Zvolen (Slovakia)
Research Station and Museum of the Tatra National Park, SK-059 60 Tatranská Lomnica (Slovakia)
Corresponding author
Paper Info
Citation
Gömöryová E, Fleischer P, Pichler V, Homolák M, Gere R, Gömöry D (2017). Soil microorganisms at the windthrow plots: the effect of post-disturbance management and the time since disturbance. iForest 10: 515-521. - doi: 10.3832/ifor2304-010
Academic Editor
Rupert Seidl
Paper history
Received: Dec 11, 2016
Accepted: Mar 17, 2017
First online: Apr 20, 2017
Publication Date: Apr 30, 2017
Publication Time: 1.13 months
Copyright Information
© SISEF - The Italian Society of Silviculture and Forest Ecology 2017
Open Access
This article is distributed under the terms of the Creative Commons Attribution-Non Commercial 4.0 International (https://creativecommons.org/licenses/by-nc/4.0/), which permits unrestricted use, distribution, and reproduction in any medium, provided you give appropriate credit to the original author(s) and the source, provide a link to the Creative Commons license, and indicate if changes were made.
Web Metrics
Breakdown by View Type
Article Usage
Total Article Views: 47606
(from publication date up to now)
Breakdown by View Type
HTML Page Views: 40379
Abstract Page Views: 2477
PDF Downloads: 3548
Citation/Reference Downloads: 22
XML Downloads: 1180
Web Metrics
Days since publication: 3008
Overall contacts: 47606
Avg. contacts per week: 110.79
Article Citations
Article citations are based on data periodically collected from the Clarivate Web of Science web site
(last update: Mar 2025)
Total number of cites (since 2017): 14
Average cites per year: 1.56
Publication Metrics
by Dimensions ©
Articles citing this article
List of the papers citing this article based on CrossRef Cited-by.
Related Contents
iForest Similar Articles
Research Articles
Effect of plant species on P cycle-related microorganisms associated with litter decomposition and P soil availability: implications for agroforestry management
vol. 9, pp. 294-302 (online: 05 October 2015)
Research Articles
Soil stoichiometry modulates effects of shrub encroachment on soil carbon concentration and stock in a subalpine grassland
vol. 13, pp. 65-72 (online: 07 February 2020)
Research Articles
Soil fauna communities and microbial activities response to litter and soil properties under degraded and restored forests of Hyrcania
vol. 14, pp. 490-498 (online: 11 November 2021)
Research Articles
Wood-soil interactions in soil bioengineering slope stabilization works
vol. 2, pp. 187-191 (online: 15 October 2009)
Research Articles
Soil respiration along an altitudinal gradient in a subalpine secondary forest in China
vol. 8, pp. 526-532 (online: 01 December 2014)
Research Articles
Impact of deforestation on the soil physical and chemical attributes, and humic fraction of organic matter in dry environments in Brazil
vol. 15, pp. 465-475 (online: 18 November 2022)
Research Articles
The manipulation of aboveground litter input affects soil CO2 efflux in a subtropical liquidambar forest in China
vol. 12, pp. 181-186 (online: 10 April 2019)
Research Articles
Spatial heterogeneity of soil respiration in a seasonal rainforest with complex terrain
vol. 6, pp. 65-72 (online: 07 February 2013)
Research Articles
Seasonal dynamics of soil respiration and nitrification in three subtropical plantations in southern China
vol. 9, pp. 813-821 (online: 29 May 2016)
Research Articles
Effects on soil characteristics by different management regimes with root sucker generated hybrid aspen (Populus tremula L. × P. tremuloides Michx.) on abandoned agricultural land
vol. 11, pp. 619-627 (online: 04 October 2018)
iForest Database Search
Search By Author
Search By Keyword
Google Scholar Search
Citing Articles
Search By Author
Search By Keywords
PubMed Search
Search By Author
Search By Keyword