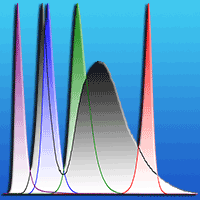
Variation in growth, photosynthesis and water-soluble polysaccharide of Cyclocarya paliurus under different light regimes
iForest - Biogeosciences and Forestry, Volume 10, Issue 2, Pages 468-474 (2017)
doi: https://doi.org/10.3832/ifor2185-010
Published: Apr 04, 2017 - Copyright © 2017 SISEF
Short Communications
Abstract
As a highly valued and multiple function tree species, Cyclocarya paliurus is planted and managed for timber production and medical use. Responses of growth, photosynthesis and phytochemical accumulation to light environment are useful informations to determine suitable habitat conditions for the cultivation of C. paliurus. A split-plot design with five light quality and three light intensity levels was adopted to compare the variations in plant growth, photosynthesis and water-soluble polysaccharide yield in C. paliurus leaves. Both light intensity and quality treatments significantly affected total biomass, photosynthetic rate and water-soluble polysaccharide yield in C. paliurus leaves. Treatments under red light and blue light with 1000 μmol m-2 s-1 achieved the highest values of biomass growth, photosynthetic rate, specific dry leaf mass per area and accumulation of water-soluble polysaccharide. These results indicate that red light and blue light with higher light intensity level were effective for increasing plant growth, photosynthesis and production of water-soluble polysaccharide in C. paliurus leaves. Manipulating light conditions might be an effective means to improve biomass and achieve higher water-soluble polysaccharide yield in C. paliurus plantations.
Keywords
Cyclocarya paliurus, Environmental Factor, Biomass Production, Phytochemicals, Photosynthesis
Introduction
Cyclocarya paliurus (Batal) Iljinskaja belongs to the Juglandaceae family and is widely distributed in mountainous regions of sub-tropical China ([10]). Leaves of this plant are traditionally used in China as a medicine or nutraceutical tea because of its unique taste ([1], [11]). Many studies have demonstrated that C. paliurus has a variety of bioactivities, including hypoglycemic activity ([20]), antihypertensive activity ([33]), anti-HIV-1 ([39]), antioxidant activity ([34]), and anticancer ([35]). However, most studies on C. paliurus were focused on plant compounds (including triterpenoids, flavonoids, steroids and saponins) and the extract activities whereas less attention was paid to the silvics of the species ([5], [6]).
Light intensity and quality are important environmental factors for plant growth and development ([38]). Specifically, changes in light quality strongly affect plant morphological, physiological, and biochemical parameters due to the spectral properties of tissue pigments ([9]). However, the responses of plants to light quality are species specific ([3]). For example, Ouyang et al. ([26]) reported that Cistanche deserticola cultured under blue light achieved higher biomass than those under red light. Yan et al. ([37]) demonstrated that red light improved salidroside production and root growth of Rhodiola sachalinensis. However, Johkan et al. ([18]) reported that green light was effective in promoting photosynthesis and plant growth of Lactuca sativa. Thus, it is necessary to determine the optimum light conditions for better growth of C. paliurus.
Polysaccharides are very common natural polymers in plants, animals and microorganisms ([32], [4]). Recently, polysaccharides from plants have attracted more and more attention due to their extensive biological activities, such as hypoglycemic activity ([31]), free-radical-scavenging activity ([4]), anticancer activity ([35]), and improvement of immunomodulation activity ([16]). Due to its biological activities, polysaccharide from C. paliurus leaves have become a focal point for research and development. The structure and antioxidant activities of polysaccharide as well as sulfated polysaccharides from C. paliurus leaves had been investigated by Xie et al. ([34], [36]). Fu et al. ([12]) also reported the seasonal and genotypic variation of leaf polysaccharide accumulation in C. paliurus, whereas knowledge of the environmental effects on polysaccharide accumulation in C. paliurus is limited.
The aims of this study were to quantify and compare the influences of varying light quality with different light intensities on plant growth, photosynthetic capacity, and water-soluble polysaccharide accumulation in C. paliurus leaves. Findings from the study are needed to better understand the responses of C. paliurus growth to differential light environment, and to provide a theoretical basis for standardized cultivation of C. paliurus plants.
Materials and methods
Plant material and growth conditions
Seeds of C. paliurus were collected from Tonggu (30° 73′ N, 116° 47′ E), Jiangxi province, China in late October 2014 and were subjected to chemical scarification, exogenous gibberellin A3 (GA3) treatments, and stratification treatments in early January 2015, according to the method proposed by Fang et al. ([10]). After a 3 month stratification treatment, the germinated seeds were transplanted into plastic pots (8.5 cm inner-diameter, 10 cm height, with holes in the bottom, one seedling per pot) filled with a substrate mixture of perlite: fowl manure: peat: soil (2: 2: 4: 2, v/v/v/v). The substrate was a loam with pH 6.44, organic matter content of 73.3 g kg-1, total N content of 72.35 g kg-1, total P content of 2.19 g kg-1, and total K content of 9.55 g kg-1. Eight weeks later, plants were moved into climate chamber and then exposed to LED lamps (Guangdong Philips Lamp Co., China).
A split-plot randomized design was used to establish three light intensity levels and five light quality treatments. Three light intensity treatments were subjected to three intensity regimes: L1 (500 ± 30 μmol m-2 s-1), L2 (750 ± 30 μmol m-2 s-1), and L3 (1000 ± 30 μmol m-2 s-1), respectively. The light intensity of LED lamps in each treatment was measured by LI-6400® system (Li-Cor, Lincoln, NE, USA). Five light quality treatments were WL (white light), BL (blue light), RL (red light), GL (green light) and PL (purple light), respectively. Spectral features of the LED lamps were recorded by means of a NIR-VIS spectrometer (Ocean Optics, USA) and reported in Fig. 1. Each treatment contains 5 replications and 8 plants per replication (plastic pot). All treatments were kept at 25 ± 2 °C and 60% relative humidity (RH) during the day, 22 ± 2 °C and 70% RH at night with a 12 h dark/light photoperiod. The plants were kept well watered once every two days until the end of the experiment.
Fig. 1 - Relative spectral irradiance of the LED lamps used. (WL): white light; (BL): blue light; (RL): red light; (GL): green light; (PL): purple light.
Growth and biomass assessment
After the growth of 5 months in the chamber, growth and biomass assessments of the plants were conducted on October 20, 2015. Intact C. paliurus seedlings in each treatment (5 seedlings) were harvested and separated into shoots and roots for biomass and water-soluble polysaccharide analysis. The leaf area (LA) (cm2) of the third and fourth fully-expanded leaves from the top of the shoots was measured at the same time with an area meter (Li-Cor Model 3100®). Biomass samples were dried (70 °C, 48 h) to constant weight and weighed. The total dry mass of each was calculated as the sum of leaf, stem, and root dry weights. The specific leaf mass (SLM) was calculated by dividing dry leaf weight by corresponding leaf area (LA - [29]).
Measurement of photosynthetic parameters
Fully developed leaves from the top of the shoots were randomly selected for gas exchange measurements, using a LI-6400® portable photosynthesis system (LiCor Inc., USA) with a standard leaf chamber equipped with a 6400-02B LED light source (LI-6400). Measurements were conducted at an air concentration of 21% O2, 380 µmol mol-1 CO2, 1000 μmol m-2 s-1 photosynthetically active radiation (PAR), 50% relative humidity and a temperature of 25 ± 2 °C. Photosynthetic rate (Pn) and stomatal conductance (gs) were recorded.
Extraction and measurement of water-soluble polysaccharide
Extraction of polysaccharide in C. paliurus leaves was carried out as described previously by Fu et al. ([12]) with slight modifications. Each sample (0.5 g) of leaves was extracted with 30 ml of 70% ethanol at 70 °C for 60 min to remove most pigments, small molecular sugars and impurities. The insoluble residues were separated, dried and then extracted twice with 20 ml distilled water at 100 °C for 75 min. The extracts were filtered and the filtrate was centrifuged at 5000 ×g for 15 min. Finally, the supernatant was combined for measurement.
Water-soluble polysaccharide content was measured using the phenol-sulphuric acid colorimetric method ([7]), using glucose as a standard, with absorbance measured at 490 nm. Concentration of water-soluble polysaccharide was quantitatively determined by the calibration curve. Water-soluble polysaccharide yield per plant was calculated by multiplying the water-soluble polysaccharide content by the leaves biomass per plant.
Statistical analysis
Data are reported as the mean ± standard deviation (SD), and all tests were performed using the SPSS® 16.0 statistical software package (SPSS, Chicago, IL, USA). A two-way ANOVA model with light quality and light intensity as the main fixed factors plus a light quality × light intensity interaction term, followed by Tukey’s multiple-range test, was performed for biomass accumulation, photosynthesis parameters, and leaf characteristics as well as the water-soluble polysaccharide yields. The data were tested for normality (Shapiro-Wilk normality test) before analysis of variance. All statistical analyses were performed at a 95 % confidence level.
Results
Variation in growth and biomass production
Two-way ANOVA showed that both light quality and light intensity treatments, as well as the interaction between them significantly affected the biomass production and allocation of C. paliurus (Tab. 1). The total biomass per seedling varied among light intensity treatments with the following order L3 > L2 > L1. This trend persisted across the growth of leaves, stem, and root (Tab. 2).
Tab. 1 - Summary of significance levels (Two-way ANOVA) for the effects of light quality, light intensity and their interaction on biomass production, water-soluble polysaccharide content and water-soluble polysaccharide yield in Cyclocarya paliurus leaves.
Source | Biomass (g) | Polisaccharide | |||||
---|---|---|---|---|---|---|---|
Root | Stem | Leaf | Total | Content (mg g-1) |
Yield (mg plant-1) |
||
Light quality (A) | df | 4 | 4 | 4 | 4 | 4 | 4 |
MS | 30.887 | 64.049 | 135.883 | 69.486 | 34.464 | 72.059 | |
Prob | < 0.001 | < 0.001 | < 0.001 | < 0.001 | 0.001 | < 0.001 | |
Light intensity (B) | df | 2 | 2 | 2 | 2 | 2 | 2 |
MS | 98.818 | 204.066 | 177.098 | 224.107 | 41.079 | 323.053 | |
Prob | 0.001 | < 0.001 | <0.001 | 0.001 | <0.001 | < 0.001 | |
Interaction (A×B) | df | 8 | 8 | 8 | 8 | 8 | 8 |
MS | 5.474 | 5.123 | 5.160 | 6.899 | 12.170 | 9.818 | |
Prob | < 0.001 | < 0.001 | < 0.001 | < 0.001 | <0.001 | < 0.001 |
Tab. 2 - Tukey’s multiple-range test of biomass, water-soluble polysaccharide content and water-soluble polysaccharide yield of Cyclocarya paliurus after a Two-way ANOVA. L1, L2, and L3 represent three irradiance levels of 500 ± 30 umol m-2 s-1, 750 ± 30 umol m-2 s-1, and 1000 ± 30 umol m-2 s-1, respectively. (WL): white light; (BL): blue light; (RL): red light; (GL): green light; (PL): purple light.
Treatment | Level | Biomass (g) | Polysaccharide | ||||
---|---|---|---|---|---|---|---|
Root | Stem | Leaf | Total | Content (mg g-1) |
Yield (mg plant-1) |
||
Light quality | RL | 2.65 a | 1.42 a | 1.27 c | 5.34 a | 34.27 ab | 44.52 c |
GL | 1.73 b | 0.89 c | 0.83 d | 3.45 b | 36.75 a | 29.29 d | |
BL | 2.67 a | 1.24 ab | 1.60 b | 5.51 a | 34.86 ab | 57.55 a | |
PL | 0.50 c | 0.51 d | 0.96 d | 1.97 c | 32.78 b | 32.01 d | |
WL | 1.78 b | 1.20 b | 2.12 a | 5.10 a | 23.51 c | 49.49 b | |
Light intensity | L1 | 0.76 c | 0.59 c | 0.83 b | 2.18 c | 27.98 c | 23.22 c |
L2 | 1.64 b | 0.98 b | 1.58 a | 4.21 b | 32.11 b | 50.73 b | |
L3 | 3.19 a | 1.58 a | 1.66 a | 6.43 a | 37.81 a | 62.76 a |
Across three light intensity levels, the highest value of total biomass was achieved at BL, RL and WL treatments (Tab. 2). However, the highest value of leaf biomass was achieved at WL treatment. Compared to WL treatment, leaf biomass of RL, GL, BL, and PL decreased by 40.1%, 60.8%, 24.5%, and 54.7%, respectively. Also, the five light quality treatments produced different biomass allocation among the seedlings parts. The highest ratios of leaf to total biomass were observed in treatments PL (48.7%) and WL (41.6%), whereas the greatest ratios of root and stem were achieved in treatment RL (76.2%), GL (75.9%) and BL (70.9%), respectively (Tab. 2).
Variation in photosynthesis and leaf characteristics
The seedlings grown under blue light and red light treatments had significantly higher photosynthetic rate (Pn) and stomatal conductance (gs) values than other light quality treatments (Fig. 2A, Fig. 2B). In the 15 treatments, the highest Pn value was detected in treatments R3 (7.09 μmol m-2 s-1) and B3 (6.89 μmol m-2 s-1), whereas the lowest value was observed in treatment P1 (0.79 μmol m-2 s-1). Two-way ANOVA showed that both light quality and light intensity treatments, as well as the interaction between them significantly affected the Pn and gs of C. paliurus (Tab. 3). Meanwhile, there was a significant decrease in Pn and gs under all light qualities over the range of light intensities from 1000 μmol m-2 s-1 (L3) to 500 μmol m-2 s-1 (L1 - Fig. 2A, Fig. 2B).
Fig. 2 - Variation in photosynthetic rate (Pn) (A), stomatal conductance (gs) (B), leaf area (LA) (C), and specific leaf mass per area (SLM) (D) of Cyclocarea paliurus under different light quality and intensity treatments (mean ± SD). Different lower case letters indicate significant differences between various treatments within a component after Tukey’s test (p < 0.05). (WL): white light; (BL): blue light; (RL): red light; (GL): green light; (PL): purple light. L1, L2, and L3 represent three irradiance levels of 500 ± 30 umol m-2 s-1, 750 ± 30 umol m-2 s-1, and 1000 ± 30 umol m-2 s-1, respectively.
Tab. 3 - Summary of significance levels (Two-way ANOVA) for the effects of light quality, light intensity and their interaction on photosynthetic rate (Pn), stomatal conductance (gs), leaf area (LA), and specific leaf mass per area (SLM) in Cyclocarya paliurus. (df): degrees of freedom; (MS): mean squares.
Source | Pn (μmol m-2 s-1) |
gs (mmol m-2 s-1) |
LA (cm2) |
SLM (g m-2) |
|
---|---|---|---|---|---|
Light quality (A) | df | 4 | 4 | 4 | 4 |
MS | 1505.678 | 913.713 | 291.358 | 130.176 | |
Prob | < 0.001 | < 0.001 | < 0.001 | < 0.001 | |
Light intensity (B) | df | 2 | 2 | 2 | 2 |
MS | 4680.091 | 2838.874 | 234.919 | 546.538 | |
Prob | <0.001 | < 0.001 | <0.001 | <0.001 | |
Interaction (A×B) | df | 8 | 8 | 8 | 8 |
MS | 156.876 | 95.179 | 12.882 | 19.190 | |
Prob | < 0.001 | < 0.001 | < 0.001 | < 0.001 |
Leaf characteristics such as leaf area (LA) and specific dry leaf mass per area (SLM) of C. paliurus were found to be significantly different under various light quality and intensity treatments (Fig. 2C, Fig. 2D). Moreover, a significant interaction of light quality and intensity was observed in LA and SLM of C. paliurus (Tab. 3). Light intensity of 500 μmol m-2 s-1 (L1) resulted in the highest LA, and LA was significantly higher in PL treatment than that in other light quality treatments (Fig. 2C). Variation trend in SLM of C. paliurus was consistent with that of Pn in leaves. In the 15 treatments, the highest SLM values were detected in treatments B3 (35.69 g m-2) and R3 (34.51 g m-2), whereas the lowest was observed in treatment P1 (3.53 g m-2 - Fig. 2D).
Variation in water-soluble polysaccharide content and yield per plant
The highest water-soluble polysaccharide contents were observed in P3 (44.58 mg g-1) and R3 (43.69 mg g-1) treatments, whereas the lowest contents were found in P1 (23.31 mg g-1) and W1 (19.09 mg g-1) treatments (Fig. 3). Two-way ANOVA showed that both light quality and light intensity treatments, as well as the interaction between them significantly affected water-soluble polysaccharide content of C. paliurus leaves (Tab. 1). The water-soluble polysaccharide content in leaves varied among light intensity treatments with the following order L3 > L2 > L1 (Tab. 2).
Fig. 3 - Variation in water-soluble polysaccharide content in Cyclocarea paliurus leaves under different light quality and intensity treatments (mean ± SD). Different lower case letters indicate significant differences between various treatments within a component after Tukey’s test (p<0.05). (WL): white light; (BL): blue light; (RL): red light; (GL): green light; (PL): purple light. L1, L2, and L3 represent three irradiance levels of 500 ± 30 μmol m-2 s-1, 750 ± 30 μmol m-2 s-1, and 1000 ± 30 μmol m-2 s-1, respectively.
Based on the leaf biomass and water-soluble polysaccharide content, the integrated effect of light quality and light intensity on the accumulation of water-soluble polysaccharide in leaves per plant was significant (p < 0.05 - Fig. 4). The greatest accumulation of water-soluble polysaccharide in the leaves per plant was achieved in treatment B3 (77.86 mg plant-1), followed by treatment R3 (70.95 mg plant-1), whereas the lowest was found in treatment P1 (11.18 mg plant-1). Compared to treatment B3, water-soluble polysaccharide accumulation in other treatments was decreased by 8.9-85.6 %. Moreover, a two-way ANOVA showed that light intensity and light quality treatments as well as their interactions significantly affected water-soluble polysaccharide accumulation of C. paliurus (Tab. 1).
Fig. 4 - Variation in water-soluble polysaccharide yield of Cyclocarya paliurus leaves in different light quality and intensity treatments (mean ± SD). Different lower case letters indicate significant differences between various treatments within a component (p < 0.05 by Tukey’s test). (WL): white light; (BL): blue light; (RL): red light; (GL): green light; (PL): purple light. Number 1, 2, and 3 represent three irradiance levels of 500 ± 30 μmol m-2 s-1, 750 ± 30 μmol m-2 s-1, and 1000 ± 30 μmol m-2 s-1, respectively.
Discussion
Variation in plant growth and photosynthesis
It is generally recognized that light intensity and light quality play an important role in plant growth, photosynthetic capacity, as well as various aspects of physiology ([24], [21]). Typically, optimal light irradiance is central to the productivity of plants, as excessive high or low light intensity often impacts photosynthesis, and then severely limit plant growth ([22]). The present study demonstrated that biomass production of C. paliurus was much lower at light intensity levels of L1 (500 μmol m-2 s-1) and L2 (750 μmol m-2 s-1) than at L3 (1000 μmol m-2 s-1 - Tab. 2), indicating that C. paliurus is a heliophyte. The observed growth response of C. paliurus to light intensity was similar to that of many tree species, such as Rauvolfia species and Camptotheca acuminata, which were reported to grown better under higher light irradiance ([2], [22]).
In higher plants, the regulation and perception of the light changes are controlled by a system of photoreceptors, including cryptochromes (blue/UV-A light receptors, 340-520 nm), phytochromes (red/far-red receptors, >520 nm) and phototropins (phot1 and phot2 - [21]). Thus, varying light wavelength produces different growth responses in plants. The percentage absorption of red or blue light by plant leaves is about 90% ([30]), consequently plant development is strongly influenced by red or blue light ([23]). This was supported by data in our study, i.e., there were significantly higher values of total biomass under BL and RL treatments. Similar results were observed in studies of other trees such as C. deserticola ([26]) and C. acuminata ([21]).
Photosynthesis, one of the most important chemical processes in higher plants, is directly linked to production of plant biomass, however, photosynthesis of plants is very sensitive to light conditions ([22]). In this study, variation in biomass accumulation in C. paliurus grown under different light quality and intensity treatments were closely linked with photosynthetic rate (Pn). Red light and blue light of 1000 μmol m-2 s-1 achieved significantly higher Pn and gs values (Fig. 2), suggesting the photosynthetic rate of C. paliurus plants increased under red and blue light, which was consistent with previous reports in other plants such as C. acuminata and cucumber ([21], [14]). It has been reported that red light is related with a highly effective light absorption through chlorophyll accumulation for photosynthesis ([8]), while blue light may promote leaf stomatal opening by activating phototropin ([17]). However, chlorophyll contents and stomatal opening of C. paliurus leaves under varying light qualities need to be further studied, as we did not measure them in this study.
The plasticity in leaf morphological and physiological characteristics may be crucial to the success of plant to establish itself in a new environment. Low light intensity may lead to increase in leaf area and seedling height. These changes may maximize the capture of available light to meet the demand for leaf photosynthesis ([28]). This was supported by the changes of LA in different light quality and intensity treatments, as we observed the highest LA values achieved at light intensity of 500 μmol m-2 s-1, especially under white light and purple light (Fig. 2C). Meanwhile, the higher SLM of plant is often considered as an index related to higher leaf photosynthetic capacity and chemical defense ([27]). Similarly, we suggest that the higher SLM may protect C. paliurus leaves against photoinhibition under blue and red light treatments.
Variation in water-soluble polysaccharide accumulation
The content of phytochemicals is often induced by environmental factors, including light quality and intensity. For example, leaf camptothecin concentrations in C. acuminata display a significantly increase under blue light and 50% shading treatments ([21], [15]). Visible light had been reported to induce proanthocyanidin biosynthesis and affect their composition, whereas UV light specifically induced biosynthesis of flavonols ([19]). In our previous studies, flavonoids production in C. paliurus plantations had been demonstrated to significantly positively correlate with total solar radiation ([21]). In the present study, water-soluble polysaccharide content in C. paliurus leaves also followed the order L3 (1000 μmol m-2 s-1) > L2 (750 μmol m-2 s-1) > L1 (500 μmol m-2 s-1 - Tab. 2). These results support the carbon/nutrient balance theory, i.e., if light becomes limiting, the decline in photosynthesis may limit plant growth and accumulation of carbon-based phytochemicals ([5]).
The effects of light quality on phytochemical accumulation are more complex and often reported with mixed results ([13], [25]). In the present study, the highest water- soluble polysaccharide contents were observed at RL and BL treatments across the three light intensity levels, which may due to the higher percentage absorption of red or blue light by leaves of C. paliurus and higher photosynthetic rate (Fig. 2A) related with carbohydrate accumulation ([8]). The goal of silvicultral practices is to obtain higher water-soluble polysaccharide yield (equal to water-soluble polysaccharide content multiplied by leaf biomass). In the present study, treatment under RL and BL at 1000 μmol m-2 s-1 were the most effective way to induce the accumulation of water-soluble polysaccharide because it resulted in the highest leaf biomass with the passage of time (Tab. 2, Fig. 4). Overall, in order to achieve the highest water-soluble polysaccharide yield per area in C. paliurus plantations, it is important to manipulate growing conditions such as light intensity and light quality. However, high-yield production of water-soluble polysaccharide in C. paliurus through manipulating light conditions needs to be further confirmed with better designed large-scale field tests.
In conclusion, blue light and red light at 1000 μmol m-2 s-1 achieved the highest total biomass, photosynthetic rate and specific leaf dry mass per area in C. paliurus. Meanwhile, treatments under blue light and red light at 1000 μmol m-2 s-1 achieved the highest water-soluble polysaccharide yield per plant, due to the higher polysaccharide content and leaf biomass. These results indicate that manipulating light intensity and quality might be an effective means to obtain higher biomass and water-soluble polysaccharide yield in C. paliurus plantations.
Acknowlegements
We acknowledge financial support from the National Natural Science Foundation of China (No. 31470637), a project funded by the Priority Academic Program Development of Jiangsu Higher Education Institutions (PAPD) and the Doctorate Fellowship Foundation of Nanjing Forestry University.
WY and YL equally contributed to this work.
References
CrossRef | Gscholar
CrossRef | Gscholar
Authors’ Info
Authors’ Affiliation
Yang Liu
Shengzuo Fang
Haifeng Ding
Mingming Zhou
Xulan Shang
College of Forestry, Nanjing Forestry University, Nanjing 210037 (P. R. China)
Shengzuo Fang
Xulan Shang
Co-Innovation Center for Sustainable Forestry in Southern China, Nanjing Forestry University, Nanjing 210037 (P. R. China)
Corresponding author
Paper Info
Citation
Yang W, Liu Y, Fang S, Ding H, Zhou M, Shang X (2017). Variation in growth, photosynthesis and water-soluble polysaccharide of Cyclocarya paliurus under different light regimes. iForest 10: 468-474. - doi: 10.3832/ifor2185-010
Academic Editor
Gianfranco Minotta
Paper history
Received: Aug 02, 2016
Accepted: Jan 19, 2017
First online: Apr 04, 2017
Publication Date: Apr 30, 2017
Publication Time: 2.50 months
Copyright Information
© SISEF - The Italian Society of Silviculture and Forest Ecology 2017
Open Access
This article is distributed under the terms of the Creative Commons Attribution-Non Commercial 4.0 International (https://creativecommons.org/licenses/by-nc/4.0/), which permits unrestricted use, distribution, and reproduction in any medium, provided you give appropriate credit to the original author(s) and the source, provide a link to the Creative Commons license, and indicate if changes were made.
Web Metrics
Breakdown by View Type
Article Usage
Total Article Views: 47728
(from publication date up to now)
Breakdown by View Type
HTML Page Views: 40304
Abstract Page Views: 2888
PDF Downloads: 3498
Citation/Reference Downloads: 23
XML Downloads: 1015
Web Metrics
Days since publication: 3009
Overall contacts: 47728
Avg. contacts per week: 111.03
Article Citations
Article citations are based on data periodically collected from the Clarivate Web of Science web site
(last update: Mar 2025)
Total number of cites (since 2017): 11
Average cites per year: 1.22
Publication Metrics
by Dimensions ©
Articles citing this article
List of the papers citing this article based on CrossRef Cited-by.
Related Contents
iForest Similar Articles
Research Articles
Links between phenology and ecophysiology in a European beech forest
vol. 8, pp. 438-447 (online: 15 December 2014)
Research Articles
Photosynthesis of three evergreen broad-leaved tree species, Castanopsis sieboldii, Quercus glauca, and Q. myrsinaefolia, under elevated ozone
vol. 11, pp. 360-366 (online: 04 May 2018)
Research Articles
The use of branch enclosures to assess direct and indirect effects of elevated CO2 on photosynthesis, respiration and isoprene emission of Populus alba leaves
vol. 1, pp. 49-54 (online: 28 February 2008)
Research Articles
Light acclimation of leaf gas exchange in two Tunisian cork oak populations from contrasting environmental conditions
vol. 8, pp. 700-706 (online: 08 January 2015)
Research Articles
Evergreen species response to Mediterranean climate stress factors
vol. 9, pp. 946-953 (online: 07 July 2016)
Research Articles
Oak sprouts grow better than seedlings under drought stress
vol. 9, pp. 529-535 (online: 17 March 2016)
Research Articles
Wintertime photosynthesis and spring recovery of Ilex aquifolium L.
vol. 12, pp. 389-396 (online: 31 July 2019)
Research Articles
Adjustment of photosynthetic carbon assimilation to higher growth irradiance in three-year-old seedlings of two Tunisian provenances of Cork Oak (Quercus suber L.)
vol. 10, pp. 618-624 (online: 17 May 2017)
Research Articles
Effects of planting density on the distribution of biomass in a douglas-fir plantation in southern Italy
vol. 8, pp. 368-376 (online: 09 September 2014)
Research Articles
Photosynthetic parameters of urban greening trees growing on paved land
vol. 12, pp. 403-410 (online: 13 August 2019)
iForest Database Search
Search By Author
Search By Keyword
Google Scholar Search
Citing Articles
Search By Author
Search By Keywords
PubMed Search
Search By Author
Search By Keyword