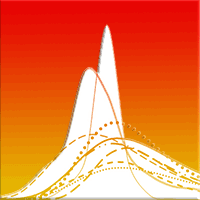
Nine-year monitoring of cambial seasonality and cell production in Norway spruce
iForest - Biogeosciences and Forestry, Volume 9, Issue 3, Pages 375-382 (2016)
doi: https://doi.org/10.3832/ifor1771-008
Published: Jan 16, 2016 - Copyright © 2016 SISEF
Research Articles
Abstract
We analyzed the relationship between weather conditions and year-to-year (1981-1989) variation in the seasonal dynamics of cambial cell production (CCP) in Norway spruce in a monoculture forest area in the Czech Republic. We found that the timing of CCP greatly varied among the studied years. The onset of CCP occurred at the beginning of May and was strongly correlated with the April mean temperature. CCP ceased by the end of August. The timing of the cessation of CCP was more variable among trees and among years than its onset. The amount of precipitation positively influenced the duration of CCP and the average rate of cell production positively correlated to the minimum temperature in January-April, as well as the maximum temperature during the growing period. Our results show that the timing and the rate of CCP of xylem cells are influenced by temperature and precipitation. However, weather-xylem growth relations of spruce from temperate forests under climatic conditions are complex, since trees are known to respond less strongly to climatic average variation than influences of extreme conditions.
Keywords
Picea abies, Temperature, Precipitation, Wood Formation, Xylem Increment, Light Microscopy
Introduction
Norway spruce (Picea abies [L.] Karst.) is one of the most widespread coniferous species in Europe ([55]) and has a considerable commercial importance. Changing environmental conditions might lead to a potential decrease in growth and yield of this species ([6]). Over the last years, several studies have been conducted on the xylogenesis of Norway spruce with the aim of investigating the plasticity of the species in terms of cell production ([10], [34], [3], [53], [5]), mostly in relation with the extreme environmental conditions predicted by future climate change scenarios ([25]).
Previous studies on Norway spruce have demonstrated that the annual wood formation period lasts from three to seven months, varying from April until October depending on site conditions ([50], [9]). It has been reported that the temperature is a crucial factor for cambial activity and cell development at the beginning of the growing season, while other factors prevail later ([19]). Gričar et al. ([20]) specified that mean temperature in April was strongly correlated with the onset of Cambial Cell Production (CCP). Similarly, Körner ([27]) pointed out that temperature primarily triggers phenological phases in this species. Furthermore, according to many studies, both daily tree growth and the duration of CCP in conifers are influenced by the minimum temperature, rather than the maximum ([46], [11], [50], [30]). As regards with high elevation sites, Norway spruce responds positively to the mean temperature in summer months, while the response is negative at lower elevations ([29]). Nevertheless, an increase in mean summer temperature is usually associated with a decrease in precipitation, which is considered to be quite a limiting factor in case of lower altitudes ([6]). In addition to temperature, water availability was also found to affect the seasonal dynamics of wood formation and radial cell dimensions of tracheids in Norway spruce ([23], [26]). Gričar et al. ([20]) reported that the phenology of CCP is highly variable and plastic among years, since Norway spruce has the ability to adjust CCP in order to function optimally in local environmental conditions. However, weather-xylem growth relations of Norway spruce growing under average climatic conditions in temperate forests are not easily detected, since trees respond less strongly to climatic variation than to extreme conditions ([34]).
In the Czech Republic, Norway spruce covers approximately 45% of the land ([40]) and is the most important commercial softwood timber. In this study, 9-year observations of the seasonal dynamics of CCP in Norway spruce growing in the Czech Republic in the 1980s (from 1981 to 1989), provides a long-term dataset of its radial growth patterns thirty years ago. Datasets of long-term monitoring of xylogenesis in trees from locations at different altitudes and latitudes are of great importance as they represent valuable information on the weather-growth relationships required to verify the existence of common climatic factors for xylogenesis ([51]).
Considering the limitations of this study (i.e., tree selection, sampling method) but also the uniqueness of tracking the timing of the phenological events as they occurred 30 years ago, we defined the aim of this study as: (1) to examine year-to-year variation in the seasonal dynamics of CCP in Norway spruce; and (2) to investigate the relationship between the timing of CCP (onset, duration and end) and local weather conditions during that period.
Materials and methods
Study site
The research was conducted in a study site managed by the Institute of Forest Ecology of the Mendel University in Brno (Czech Republic). The plot was located in the monoculture forest area Drahanska vrchovina, 30 km north of Brno (49° 29′ 31″ N; 16° 43′ 30″ E, 600-660 m a.s.l.). The soil type was modal oligotrophic Cambisol, while the bedrock consisted of intrusive rock acid granodiorite of the Brno Massive ([24]). It is considered to be a moderate climatic region ([44]), with average annual air temperature 7.6 °C and average annual precipitation of 717 mm ([22]).
According to weather data obtained from the Protivanov weather station (Czech Hydrometeorological Institute), which was located approximately 10 km away from the research site, the coldest temperature in the examined years (1981-1989) was usually reached in the period December-February, with a minimum temperature of -8.6 to -22.7 °C (Fig. 1). The warmest period was June-August, with a maximum temperature of 21.9 to 25.6°C. Annual precipitation ranged from 486 to 793 mm, whereby the wettest months were recorded during the growing season (Tab. 1). In 1983 and 1989 the highest annual mean temperature (7.1 and 7.2 °C, respectively) coincided with the lowest annual precipitation amount (486.4 and 499.4 mm, respectively).
Fig. 1 - Climate diagram of the study area for the period 1981-1989. Lines represent the mean monthly temperature, monthly maximum and monthly minimum temperature, while bars represent the total monthly precipitation (Protivanov weather station, Czech Republic).
Tab. 1 - Annual mean air temperature (T) parameters (°C), annual amplitude (K) and annual cumulative precipitation (P) during 1981-1989. The corresponding month is in parenthesis. (*): Calculation based on monthly maximum and minimum temperature values.
Year | Annual mean T (°C) | Annual maximum T* (°C) | Annual minimum T* (°C) | Warmest month T (°C) | Coldest month T (°C) | Annual amplitude T (K) | Annual P (mm) | Highest P month (mm) | Lowest P month (mm) |
---|---|---|---|---|---|---|---|---|---|
1981 | 6.0 | 12.0 | -0.8 | (8) 22.2 | (1) -12.7 | 13.4 | 793.3 | (10) 103.4 | (2) 26.9 |
1982 | 6.5 | 12.3 | 0.8 | (6) 21.9 | (1) -13.9 | 11.5 | 525.5 | (8) 113.9 | (2) 0.7 |
1983 | 7.1 | 14.3 | 0.0 | (7) 26.6 | (2) -11.2 | 14.3 | 486.4 | (6) 70.9 | (7) 9.2 |
1984 | 5.6 | 12.6 | 0.5 | (7) 23.8 | (2) -9.6 | 12.1 | 645.4 | (5) 125.1 | (8) 22.9 |
1985 | 5.0 | 12.6 | -2.4 | (8) 22.1 | (2) -19.0 | 15.0 | 849.1 | (9) 190.2 | (11) 16.3 |
1986 | 5.8 | 12.4 | -1.4 | (6) 22.0 | (2) -15.9 | 13.7 | 682.0 | (6) 121.1 | (2) 21.4 |
1987 | 5.3 | 12.2 | -2.2 | (7) 21.9 | (1) -22.7 | 14.4 | 743.1 | (6) 158.5 | (2) 26.6 |
1988 | 6.4 | 12.4 | 0.1 | (7) 25.6 | (12) -11.0 | 12.3 | 613.3 | (8) 119.9 | (4) 10.4 |
1989 | 7.2 | 13.6 | 0.9 | (8) 23.6 | (12) -8.6 | 12.7 | 496.4 | (6) 106.9 | (1) 8.6 |
Drought indices enable monitoring drought severity, duration and frequency by simplifying complex environmental interactions and quantifying weather extreme values ([58]). The Standardized Precipitation Index (SPI) developed by McKee et al. ([36]) was chosen as a well-tested and widely-accepted index of drought severity ([56]). The SPI is based on the fact that every factor involved in the water resources system (soil moisture content, groundwater, snowpack, river discharges and reservoir storages) eventually depends mostly on the deficit of the precipitation rate over different time scales ([36], [60]). Many studies have correlated the SPI with other indices, such as the Palmer drought severity index (PDSI - [31], [45]) or the Standardized Precipitation-Evapotranspiration Index (SPEI), derived from SPI by including temperature data for detecting potential evapotranspiration (PET) influence on drought ([60], [17], [41]). Vicente-Serrano et al. ([60]) reports that the inclusion of PET to calculate the SPEI affects the index only when PET differs from average conditions, for example, under global warming scenarios.
The SPI is based on the long-term precipitation record (for an examined period for any location), which is fitted to a probability distribution transformed into a normal distribution such that the mean SPI for the location and the desired period is zero ([36], [38]). The SPI detects drought on multiple time-scales, usually computed over five running time intervals, i.e., 1, 3, 6, 9, and 12 months, but the index is also adjusted to the specific period chosen. Hence, the SPI is particularly suited to compare drought conditions between time periods and regional climatic conditions using only precipitation data. SPI was calculated using the free software package DrinC (Drought Indices Calculator - [57]). The SPIannual was produced based on water years (WY), starting from the previous October up to the current September (each WY included one growth period). Additionally, both SPIJan-Apr and SPIMay-Aug were also calculated and adjusted for two 4-month time intervals before and during the growth period (Fig. 2).
Fig. 2 - Drought events during the examined period according to SPIannual, SPIJan-Apr, SPIMay-Aug. Water years (WY) start from the previous year (October) to the current year (September). The classification of drought conditions according to SPI is reported in the right box.
Fig. 3 - Growing degree days (GDD) at the onset of cambial cell production (CCP) in Picea abies by years. Tb = 5 °C (Tb =base temperature). Columns represent mean values, bars represent standard deviations.
The potential relationship between weather conditions and the occurrence of phenological phases was investigated by calculating the Growing Degree Days (GDD
), a measure of heat accumulation ([37]). GDD
provided an estimation of the cumulative temperature (°D) connected with the early phenological phases. Daily GDD
was calculated for every tree and year in the examined period (1981-1989 - Fig. 3) as follows (eqn. 1, eqn. 2):
where T
max and T
min are the maximum and minimum daily air temperatures, respectively, and T
base is the base temperature above which CCP takes place after a certain amount of temperature has been accumulated. A threshold of 5 °C was chosen for T
base ([52], [21]). Negative values of GDD
daily were set to zero. GDD
daily values were then summed starting from the 1st day of the year (DOY 1, t
= 1) each year until the onset DOY of CCP (t
= n
) as follows (eqn. 2):
Differences in GDD
values among the years were tested using one-way repeated-measures ANOVA.
Sample collection and preparation
Tree selection, sample collection and slide preparation for microscope analysis were performed in 1981. From 1981 to 1989, six dominant and co-dominant Norway spruce trees were selected each year (54 trees in total). Microscopic observations of the intra-annual wood formation were carried out in 2014 on 49 trees (5 trees were excluded due to spoiled slides).
All trees were approximately 75 years old, with diameter at the breast height of about 21 cm and an average height of 23 m. Sampling was performed at 14-days intervals using a modified hammer-driven cylindrical punch (Doležal’s hammer punch - 10 mm in diameter) around the circumference of the stem, approximately 1.3 m above the ground. In order to avoid wound effects, sampling points on the stem were separated by at least 10 cm. Microcores of 1 cm in length were obtained, including the inner phloem, the cambium and at least two most recently formed xylem growth rings. Microcores were immediately immersed in formaldehyde-acetic acid-ethanol solution (FAA) for 24 hours ([62]), and then rinsed with distilled water, dehydrated in a graded series of ethanol, and finally sectioned using a Jung K® rotary microtome (Leica, Wetzlar, Germany). Sections 20-40 μm in thickness were stained in safranine and light green yellowish SF (triarylmethane dye) and mounted on permanent slides using Canadian Balsam ([23]). The permanent slides of cross sections of the sampled tissues were prepared at the end of each growing period and stored.
Observations of cross-sections and histometric analyses were performed in 2014 by a Leica DMLS® microscope (Leica Microsystems, Wetzlar, Germany) connected to a Leica DFC 280® digital camera (Leica Microsystems, Wetzlar, Germany). Image processing was carried out using the public-domain software package ImageJ ([1]).
Measurements and data processing
Cambial cells and currently formed xylem cells were counted along three radial files on transverse sections, and then averaged ([12]). For each tree, the following phenological phases of CCP were assessed and the relative DOY computed: (1) the onset of CCP was identified as an increased number of thin-walled cambial derivatives ([49]); (2) the cessation of CCP was identified as the time at which no new thin-walled cambial derivatives were observed adjacent to the cambium and the number of cambial cells was comparable to the number before its reactivation in spring; (3) the duration of the period of CCP was calculated in number of days; (4) the final tree-ring width was expressed as number of cells; (5) the average rate (final number of cell/duration of CCP) of xylem cells produced per day ([20]); and (6) maximum rate of xylem cells produced per day, which was calculated using a Gompertz function that indicated the maximum growth rate at the inflection point of the curve ([47]).
The number of xylem cells was fitted per tree and year (1981-1989) to the Gompertz function ([47], [32] - eqn. 3):
where y
is the weekly cumulative cells, t
is the day of year, A
is the upper asymptote, representing the maximum number of cells, B
is the place on the x-axis, estimating the beginning of cambial activity, and k
is the inflection point on the curve. The first derivatives of each Gompertz function were calculated to emphasize differences in the rate of production of xylem cells along the growing seasons.
Pearson’s correlation coefficients were calculated to measure the strength of association between xylem and tree-ring width, the length of growth season, as well as the rate of cell division. Differences among years in cambial phenology and xylem increments (expressed in number of cells) were tested by individual one-way repeated measures ANOVA.
To analyze the weather-growth relationships, Pearson’s correlation coefficients between weather data (precipitation and temperature) and phenological data were calculated. One-way ANOVA was used for analysing cambium phenology and GDD values in comparison with dry/normal/wet conditions. Statistical analysis was carried out using Microsoft Excel® spreadsheets and the IBM SPSS Statistics® software package.
Results
Weather conditions
Weather conditions during the growing seasons differed among the nine study years. In general, annual mean temperature was inversely proportional to the annual amount of precipitation, and it was lower in the case of higher rainfall. In addition, the wettest months occurred during the growing season. SPIannual values were negative in 1983 and 1989, indicating dry periods, whereas 1985 was the wettest year. The 4-month interval SPIJan-Apr revealed dry conditions prevailing before the onset of CCP in 1983 and 1989 (WY 1982-1983 and 1988-1989, respectively) which turned into normal during summer (SPIMay-Aug). The SPIMay-Aug depicted the summer drought occurred in 1984. Other study years were considered as normal with regard to water availability.
Comparison of the growing degree days (GDD
) showed various amounts of accumulated heat units (°D) at the onset of CCP in the nine years (F = 2.66, p < 0.0076). The highest GDD
value was recorded in 1989 (195.10 ± 80.10 °D) and the lowest in 1985 (100.55 ± 26.40 °D). The highest variation in GDD
among the sampled trees was recorded in 1984 (coefficient of variation: 0.48) and the lowest in 1987 (coefficient of variation: 0.21).
Cambium phenology
The onset of CCP occurred in early May for most of the studied years and ceased by the end of August. CCP lasted longer in 1985 and was shortest in 1989. In the latter year, a delay of almost two weeks was observed compared with the other examined years. The onset, ending and duration of CCP significantly differed among the examined years (onset: F = 2.91, p = 0.0012; ending: F = 2.80, p = 0.0015; duration: F = 2.26, p = 0.04 - Fig. 4A). Although the widths of annual xylem increments did not differ in the study years (F = 0.841, p = 0.57), the average annual increments were widest in 1989 (51.56 ± 15.94 cells) and narrowest in 1981 (24.22 ± 7.81 cells - Fig. 4B).
Fig. 4 - (A): Onset (CP), cessation (ECP) and duration of cambial cell production (CCP) in Norway spruce from 1981-1989. Points represent mean values, bars are the standard deviation. (B): Final width of xylem growth rings expressed in number of cells. Columns represent mean values, bars represent standard deviations..
Similarly, the average rate of cell production also varied between 0.25 and 0.43 among the studied years. The maximum rate of cell production (inflection point of the curve) was reached in the period from 8 June to 6 July and was highest in 1983 and 1989, with 1.02 and 1.4 cells day-1, respectively (Fig. 5). However, the peak period of cell production differed, being in 1989 almost three weeks later than in 1983. In other examined years, the maximum production rate ranged from 0.24 (in 1982) to 0.60 cells day-1 (in 1988). Despite the summer drought in 1984 (SPIMay-Aug = -2.59), the maximum rate of cell production was 0.47 cells day-1.
Fig. 5 - First derivatives of Gompertz functions describing xylem growth ring formation in Norway spruce from 1981-1989. The peaks of the function represent the time of maximum rate of cell production (inflection point of the curve).
Weather-growth relationship
A vast number of possible weather-growth relationships were examined (Pearsons’ correlations) between phenological and weather data (temperature and precipitation of various timings). The strongest weather-growth correlations are reported in the Tab. 2. The onset of CCP strongly correlated with mean temperature in April. The average temperature of the CCP period strongly affected the duration and cessation of CCP. Additionally, the duration of CCP was highly controlled by precipitation during this period. The final number of xylem cells was negatively correlated with mean monthly maximum temperature of June. The maximum rate of produced cells positively correlated with the January-April minimum temperature, as well as with the maximum temperature during CCP. Finally, GDD
strongly correlated with the onset and cessation of CCP, the number and the maximum rate of produced cells.
Tab. 2 - Pearson’s correlations for the onset (CP), ending (ECP) and duration of cambial cell production, xylem ring width (expressed in number of cells) and maximum rate of cell production. (T): temperature (°C); (P): precipitation (mm); (*): p < 0.05 (2-tailed).
Weather conditions | CP | ECP | Duration of CP |
No of cells |
Average rate of cells |
---|---|---|---|---|---|
Tmean April | -0.692* | - | - | - | - |
Tmean August | - | - | - | - | 0.672* |
Tmean period of duration of CP | - | - | -0.671* | - | - |
Tmin Jan - Apr | - | - | - | - | 0.695* |
Tmin Jan - Aug | - | - | - | - | 0.699* |
Tmin water years (WY) | - | - | - | 0.694* | 0.704* |
Tmax Jun | - | - | - | -0.765* | -0.677* |
Tmax period of duration of CP | - | - | - | - | 0.694* |
P period of duration of CP | - | 0.669* | 0.735* | - | - |
P water years (WY) | - | - | - | -0.709* | - |
P September-September | - | - | 0.692* | - | - |
GDD | 0.654* | -0.695* | - | 0.767* | 0.687* |
Discussion
Impact of temperature on CCP onset
The quantity and quality of the produced wood, as well as the environmental adaptivity of trees are largely determined by the timing of cambial reactivation ([4]). Temperature has been identified as the dominant environmental signal that triggers tree phenology ([27]). Rossi et al. ([50]) demonstrated that a threshold temperature plays a decisive role for xylogenesis in conifers growing under cold conditions. A major impact of temperature on the onset of CCP was also clearly demonstrated in experiments of local heating and cooling of spruce stems, causing earlier initiation and higher rates of CCP in the case of heating and a delay in cambial reactivation and lower rates of cell production in the case of cooling ([19]). In this study, we found that the timing of CCP greatly varied among the studied years. The onset of CCP occurred at the beginning of May and strongly correlated with the mean temperature of April. This is in line with the findings of Gričar et al. ([20]) in Norway spruce. In this study, the annual mean temperature was in general inversely proportional to the annual rainfall; consequently GDD values were highest in 1989 and 1983 (195.10 and 172.6 °D, respectively). However, we observed a fairly large variation in the GDD values required for the onset of CCP among the years, indicating that the temperature is not regularly limiting for the growth of spruce at this temperate forest site.
The observation that high winter or early spring temperatures generally promote growth is consistent with the fact that cambial reactivation of evergreen conifers is strictly triggered by a rise of temperature ([19], [4]). Since photosynthesis in conifers is possible in winter ([16]), high temperatures during the winter period could play a positive role in improving carbohydrate storage and growth in the following year. Additionally, warmer winters might decrease embolism ([8]) and accelerate snowmelt, favoring soil warming and promoting mycorrhizal root growth. Such conditions promote bud formation as well as needle and shoot maturation, thus increasing the growing season of the following year. Finally, these factors, in combination with a suitable water supply, promote early wood formation during spring ([28]).
Impact of climatic conditions on duration and rate of CCP
CCP in the Norway spruce ceased by the end of August, which is in accordance with observations by other studies on this species ([23], [20]). The amount of precipitation proved to be the main factor that positively influenced the duration of CCP, whereas the average rate of cell production positively correlated with the monthly minimum temperature in January-April, as well as with the monthly maximum temperature during the growing period. The timing of the cessation of CCP was more variable among trees and years as compared with its onset. The temperature at the end of cell production was still high and favorable for radial growth. In previous studies, this has been explained by the need to ensure enough time for the development of the latest formed tracheids before winter, which in wide xylem rings can last up to two months after the cessation of cell production ([18], [50]).
The minimum temperature during WY strongly correlated with the production of cells and daily tree growth. The years 1983 and 1989 were found to be dry according to the SPIannual. This is in line with SPEI results from a recent study conducted in the Czech Republic ([43]). Although the years 1983 and 1989 were identified as dry years according to the SPIannual, they were characterized by the fastest production of cells. However, it has to be stressed again that drought occurred before the growing season (SPIJan-Apr), while conditions changed to normal during the growing season (SPIMay-Aug). In the case of 1983, CCP started earlier, reaching the maximum rate of cell production (1.02 cells day-1) also earlier. In 1989, an even higher maximum rate of cell production (1.4 cells day-1) was reached in a short period of almost 20 days. Recent study on spruce growing in temperate forests resulted in 0.44 ± 0.03 cells day-1 ([9]). The maximum rate of cell produced during the summer drought in 1984 was in line with Cuny et al. ([9]), presumably due to moderately wet conditions prevailing before the growing season. Nevertheless, we did not find any strong correlation between the duration of cell production and the timing of maximum cell production or the rate of cell production.
Impact of temperature and precipitation on xylem increments
In numerous studies on radial growth of tree-rings conducted in central and northern Europe, temperature was identified as the primary growth factor at higher altitudes ([61], [33], [39], [50]), though precipitation also controls radial growth especially at low altitudes ([28], [6]). We found a positive relationship between the final width of the annual xylem increments and GDD
values, but negative with maximum temperature in June. In northern Europe, June temperature is usually the most important factor ([34], [2]). At high elevation sites, the response of Norway spruce to the mean temperature in summer months is positive, while it is negative at lower elevation sites ([29]). An increase in mean summer temperature is usually associated with a decrease in precipitation and represents the limiting factor for spruce at lower altitudes ([6]). In our case, precipitation did not appear to play an important role on ring width, as had been found for temperature.
In general, ring widths depend on the duration and rate of CCP ([54]). In this study, xylem widths were predominantly related to the rate of cell production. The maximum rate of cell production was reached in the period from 8 June until 6 July, which is in line with the observation of Rossi et al. ([48]). These authors reported that maximum daily growth rates in conifers at colder sites in the northern hemisphere occur approximately at the summer solstice. This photoperiodic growth constraint is thus an adaptation of trees to native climatic conditions, allowing them to complete differentiation of terminal latewood cells before winter ([48]). Unlike temperature, the photoperiod is the same at a given date and locality each year. The cessation of growth in response to declining photoperiods is thus an indirect adaptation to the temperature regime ([14]).
Conclusions
Xylem formation studies are usually conducted on trees growing near either timberline or xeric habitats, where primarily temperature or water availability are expected to play a key role on growth ([13], [42], [59]). Similar studies in temperate regions are more complicated, since the growth may be also driven by many biotic and abiotic factors ([7], [15]). Local site conditions, tree age, tree competition, tree vitality, as well as different forest management practices, can be more important drivers of local-scale growth trends than the regional climate signal ([29], [35]). Our results showed that the timing of CCP and the widths of xylem cells were influenced by temperature and precipitation. However, the weather-xylem growth relations of Norway spruce growing under average climatic conditions in temperate forests are not explicit. Since trees respond less drastically to normal conditions ([34]), further investigations are needed to elucidate the relationship between weather conditions and tree growth.
Acknowledgements
KG together with JG developed the concept of the paper, wrote the paper, prepared the figures and tables and performed the wood-anatomical analysis; VG provided the cross-sections and performed the wood-anatomical measurements and helped to develop the concept of the paper; HV helped to prepare the figures and measurements, wrote some parts of the results and discussion.
The authors gratefully acknowledge the help of the xylogenesis research team from the 1980s: A. Matovič, J. Šlezingerová, L. Gandelová, V. Štepánek and Z. Fouskova for their work in the field and in the laboratory. The work was supported by the Post-doc Project CZ.1.07/2.3.00/30.0031 at MENDELU and the state budget of the Czech Republic, Project Indicators of Trees Vitality Reg. No. CZ.1.07/2.3.00/20.0265. The cooperation among the international partners was supported by COST Action FP1106 “STReESS”. We thank Martin Cregeen for language editing. Our special thanks to the Czech Hydrometeorological Institute for providing us with the long-term weather data.
List of abbreviations
The following abbreviations have been used throughout the text:
- CCP: Cambial Cell Production;
- SPI: Standardized Precipitation Index;
- SPIannual: Annual Standardized Precipitation Index;
- SPIJan-Apr: Annual Standardized Precipitation Index from January to April;
- SPIMay-Aug: Annual Standardized Precipitation Index from May to August;
- WY: Water Years;
- GDD: Growing Degree Days;
- DOY: Day of the Year .
References
Gscholar
CrossRef | Gscholar
CrossRef | Gscholar
Online | Gscholar
Gscholar
Online | Gscholar
Gscholar
Gscholar
Gscholar
CrossRef | Gscholar
Gscholar
Authors’ Info
Authors’ Affiliation
Hanuš Vavrčík
Vladimír Gryc
Mendel University in Brno, Faculty of Forestry and Wood Technology, Department of Wood Science, Zemedelska 3, 613 00 Brno (Czech Republic)
Slovenian Forestry Institute, Vecna pot 2, SI-1000 Ljubljana (Slovenia)
Corresponding author
Paper Info
Citation
Giagli K, Gričar J, Vavrčík H, Gryc V (2016). Nine-year monitoring of cambial seasonality and cell production in Norway spruce. iForest 9: 375-382. - doi: 10.3832/ifor1771-008
Academic Editor
Paolo Cherubini
Paper history
Received: Jul 15, 2015
Accepted: Oct 05, 2015
First online: Jan 16, 2016
Publication Date: Jun 01, 2016
Publication Time: 3.43 months
Copyright Information
© SISEF - The Italian Society of Silviculture and Forest Ecology 2016
Open Access
This article is distributed under the terms of the Creative Commons Attribution-Non Commercial 4.0 International (https://creativecommons.org/licenses/by-nc/4.0/), which permits unrestricted use, distribution, and reproduction in any medium, provided you give appropriate credit to the original author(s) and the source, provide a link to the Creative Commons license, and indicate if changes were made.
Web Metrics
Breakdown by View Type
Article Usage
Total Article Views: 41609
(from publication date up to now)
Breakdown by View Type
HTML Page Views: 35799
Abstract Page Views: 1857
PDF Downloads: 3002
Citation/Reference Downloads: 32
XML Downloads: 919
Web Metrics
Days since publication: 3115
Overall contacts: 41609
Avg. contacts per week: 93.50
Article Citations
Article citations are based on data periodically collected from the Clarivate Web of Science web site
(last update: Feb 2023)
Total number of cites (since 2016): 10
Average cites per year: 1.25
Publication Metrics
by Dimensions ©
Articles citing this article
List of the papers citing this article based on CrossRef Cited-by.
Related Contents
iForest Similar Articles
Research Articles
Wind contribution to yearly silver fir (Abies alba Mill.) compression wood development in the Romanian Carpathians
vol. 9, pp. 927-936 (online: 02 October 2016)
Short Communications
Culturable fungi associated with wood decay of Picea abies in subalpine forest soils: a field-mesocosm case study
vol. 11, pp. 781-785 (online: 28 November 2018)
Research Articles
The effect of provenance of historical timber on tree-ring based temperature reconstructions in the Western Central Alps
vol. 13, pp. 351-359 (online: 25 August 2020)
Research Articles
Impact of climate change on radial growth of Siberian spruce and Scots pine in North-western Russia
vol. 1, pp. 13-21 (online: 28 February 2008)
Short Communications
Climate effects on growth differ according to height and diameter along the stem in Pinus pinaster Ait.
vol. 11, pp. 237-242 (online: 12 March 2018)
Research Articles
Auxin (IAA) and soluble carbohydrate seasonal dynamics monitored during xylogenesis and phloemogenesis in Scots pine
vol. 11, pp. 553-562 (online: 01 September 2018)
Research Articles
Response to climate and influence of ocean-atmosphere phenomena on annual radial increments of Pinus oocarpa Schiede ex Schltdl. & Cham in the Lagunas de Montebello National Park, Chiapas, Mexico
vol. 16, pp. 174-181 (online: 30 June 2023)
Research Articles
Improving dimensional stability of Populus cathayana wood by suberin monomers with heat treatment
vol. 14, pp. 313-319 (online: 01 July 2021)
Research Articles
Anatomical and genetic aspects of ash dieback: a look at the wood structure
vol. 10, pp. 522-528 (online: 20 April 2017)
Research Articles
Scots pine’s capacity to adapt to climate change in hemi-boreal forests in relation to dominating tree increment and site condition
vol. 14, pp. 473-482 (online: 18 October 2021)
iForest Database Search
Search By Author
Search By Keyword
Google Scholar Search
Citing Articles
Search By Author
Search By Keywords
PubMed Search
Search By Author
Search By Keyword