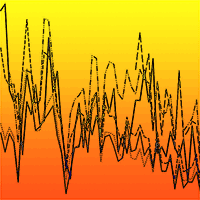
Climate effects on growth differ according to height and diameter along the stem in Pinus pinaster Ait.
iForest - Biogeosciences and Forestry, Volume 11, Issue 2, Pages 237-242 (2018)
doi: https://doi.org/10.3832/ifor2318-011
Published: Mar 12, 2018 - Copyright © 2018 SISEF
Short Communications
Abstract
Climate-growth relationships in forest trees are increasingly the focus of research aimed at understanding and assessing responses to climate change. Many studies have been confined to annual radial growth at breast height as an easy-to-measure dendrological standard variable, although its validity as a proxy for overall annual growth patterns in trees has scarcely been addressed. In this study, we test this hypothesis by exploring additional information on climate-growth relationships as well as analyzing both the radial growth at different stem heights and the height increment. For this purpose, past annual radial growth and shoot elongation were measured in 10 dominant Pinus pinaster Ait. trees in a 130-year-old stand. Radial increments were measured on disks taken from five different trunk heights up to 15 meters. Height increments were obtained by measuring the distance between consecutive branch whorls, which appear as knots after sawing a longitudinal section of the stem. The relationships between climate and both radial growth and height increment were analyzed through Pearson’s correlations and the response to extreme climatic episodes was analyzed using resilience indices. Results revealed that the climatic variables affecting growth were different for height and stem diameter. Additionally, in the case of stem diameter, the climatic variables affecting growth also depended on the height at which the sample was taken. Precipitation prior to bud break, both in the year in which the studied shoot elongation takes place and in the previous year, has a positive effect on height increment. Radial growth in the upper part of the stem was mainly influenced by spring temperatures and precipitation, whereas in the case of basal radial growth it was the autumn and winter temperatures and precipitation of the previous year to growth which had the greatest influence. Similarly, severe droughts cause greater decline in height increment, while the decline in radial growth of the upper part of stem is smaller than that of radial growth at breast height. In conclusion, the analysis of height increment and upper radial growth provides important information to complement the dendroclimatology data for radial growth at breast height, thus improving our understanding of the impact of climate change on tree growth.
Keywords
Dendrochronology, Climate Growth Response, Growth Allocation, Stem Analysis, Climate Sensitivity, Resilience
Introduction
Climate change projections for the Mediterranean region, based on global and regional climate model simulations, provide an overall picture of substantial drying and warming of the region, especially in the summer season. In a scenario with intermediate CO2 emissions, it is estimated that the decrease in precipitation will exceed 15% and that warming will reach 3.6 °C ([13]). Since the middle of the 20th century, the increasing impact of climate change on forest productivity due to changes in water availability and/or temperature is evident ([22]). Therefore, studying climate-growth relationships can provide valuable information to assess the vulnerability of forests to climate change and possible changes in productivity. Furthermore, such studies allow adaptive management measures to be developed in order to mitigate the effects of climate change ([27]).
Resampling diameters at breast height is considered an easy-to-measure proxy for stem growth. However, several studies have shown that radial growth at breast height may not be representative of radial growth patterns along the stem ([4], [5], [14], [27], [15]). Differing rates of growth along the stem can be caused by vertical gradients in hydraulic constraints and priorities for carbon allocation. Due to gravitational effects on xylem tensions, the sensitivity of radial growth to climate increases consistently with height along the stem ([14]).
Although diameter growth is influenced by age, stand density, and natural or anthropogenic disturbances, methods to remove these effects have been developed for the purpose of comparing different stands ([28]). However, the site factors that determine productivity are more correlated with stand height than stand diameter, since the latter depends largely on the stand density and therefore on forest management. Dominant height growth in even-aged stands is scarcely modified by silviculture and is frequently used as a site productivity indicator ([26]). Therefore, given the dependence of dominant tree height growth on climatic conditions, this relationship may be of great interest when analyzing the possible impact of climate change on forest productivity ([31]) at specific sites.
Height increment in monocyclic pines is mainly predetermined by the climatic conditions during the year previous to the growth ([23]), whereas the climate during the growth period itself ([12]) also influences polycyclic pines. As with radial growth series, height increment series can be synchronized and standardized, and the response to climatic variables can be explored following the standard methods of dendrochronology ([23]). However, it is more difficult to measure height increment series than it is to measure tree rings in a radial core for radial growth. Moreover, it frequently requires destructive sampling ([23]).
Extreme episodes such as drought, heavy rains and extreme heat or cold events can lead not only to abrupt declines in growth but can also affect the growth and production of the forest for one year or even several successive years. Resilience of an individual, broadly defined, is its capacity to recover after disturbance ([16]). Most resilience studies focus on tree dieback and forest vulnerability, and generally use growth samples taken at breast height ([20], [29]). Although a few studies addressing resilience have used height increment series ([21]), to our knowledge none have used tree-ring width series from different stem heights.
The main objective of this study was to determine whether height increment and radial growth series sampled at different stem heights can contribute to improve our understanding of the effects of climatic conditions on the growth of Mediterranean Maritime pine (Pinus pinaster Ait.). The specific questions addressed in our study are: (i) how does sensitivity to climate variability differ between height increment and tree-ring width along the length of the stem? (ii) How do height increment and diameter increments along the stem respond to extreme climatic events? (iii) Which climate variables influence tree height increment and tree-ring width at the study site? Our main hypothesis was that height and diameter growth along the stem respond differently to climatic conditions and therefore provide valuable information for evaluating the effects of climate change on productivity, as well as tree vulnerability to extreme climatic events.
Material and methods
Study site and field data
Data was collected from a regeneration felling in a permanent plot belonging to INIA-CIFOR (plot ID: Soria-44) in winter 2009. The plot is situated in an artificially regenerated, even-aged, 130-year-old stand of Pinus pinaster Ait., which is a polycyclic species. The plot, located near Bayubas de Arriba, Soria, Spain (41° 33′ N, 02° 55′ W) at an elevation of 1000 m a.s.l., is gently sloping (5%) and has sandy soils.
The climatic data used in this work is taken from the El Burgo de Osma weather station, which is located at approximately 12 km from the stand (AEMET©, 41° 35′ N, 03° 04′ W, 895 m a.s.l.) and provides records for the period from 1932 to 2008. Missing data were estimated by linear regression, which relates climatic variables from this station and from the Bayubas de Abajo weather station (AEMET©, 41° 32′ N, 02° 55′ W, 1000 m a.s.l.), the latter providing records for the period 1955-2002 (see Fig. S1 in Supplementary material for linear regressions relating both weather stations).
The site has a Mediterranean climate. In the period 1932-2008, mean annual precipitation was 520 mm, mean annual temperature was 10.4 °C, highest temperature was 40.0 °C, lowest temperature was -22.0 °C, mean maximum temperature of the hottest month was 28.6 °C, and mean minimum temperature of the coldest month was -2.0 °C. The average drought length was two months (July-August).
Ten dominant trees, free of visible damage and which had never been pruned along the stem, were felled in the experimental plot to enable sampling. The mean DBH of these trees (± standard deviation, SD) was 48.4 ± 1.8 cm, mean height 17.7 ± 0.9 m and mean height of the first green branch 12.2 ± 0.8 m. Previous studies have proven that a sample of ten trees from a given stand is sufficient to describe the development of height increment and radial growth along the stem ([4], [23], [15]).
Once a tree had been felled, its stem was sawn longitudinally to measure the annual height increments according to the location of the branch whorls along the tree trunk. Height increments were measured to the nearest centimeter and dated in the field. In addition, we sampled five disks per tree from different stem heights: basal (0.3 m), breast height (1.3 m) and at heights of about 8, 12 and 15 m. The last three heights varied depending on total tree height and branch distribution. The disks were taken to the laboratory and sanded with progressively finer grades of sandpaper (ANSI 80, 120, 220, 320 and 400 grit). Two or more radii were selected on each disk according to the eccentricity of each one, avoiding the vicinity of reaction wood. The rings were measured to the nearest 0.01 mm using the Lintab® device and the TSAP-Win® software (Rinntech, Heidelberg, Germany).
Chronology statistics
Annual tree radii increments were cross-dated, listing the characteristics rings. All the tree radii were then compared to identify shared characteristics rings. Annual height increment series were also cross-dated, listing any increments that were especially large or small and the years with multiple shoot growths. The visual cross-dating of tree-ring series was further verified using the COFECHA program ([11]) with a rigidity of spline of 32 years. This rigidity is considered the optimum for error detection ([10]). As regards the height chronologies, only the growths from 1930 to 2008 were used because cross-dating revealed that measurements of growth prior to this period may not be sufficiently reliable, due to the fact that young trees often present multiple shoots as well as greater variability in height growth among individuals, thus complicating annual growth reconstruction ([8]).
The ARSTAN software ([11]) was employed to create residual chronologies of tree-ring width for each sampling height (basal, 1.3, 8, 12 and 15 m) as well as for height increment using a double detrending procedure: a negative exponential curve to remove age trend and a cubic smoothing spline using a rigidity of 32 years. This removes the low-to-medium frequency disturbance components from the data. In order to remove persistence that may remain after the spline, an autoregressive (AR1) model was fitted to the measurement series.
The qualities of the chronologies were assessed using the following statistical parameters: mean correlation coefficient among tree-growth series and master reference chronology for each group of chronologies (basal, 1.3, 8, 12 and 15 m tree-ring width series and height increment series); standard deviation; autocorrelation ([10]); mean sensitivity (MS), which expresses the degree by which one or more causal factors are reflected by a tree-ring series ([24], [10]); signal-to-noise ratio, the proportion of the variability explained by climate or other causal factors divided by the residual or unexplained variability ([7], [11]). These parameters were computed both for their entire length and for the period common to all chronologies using COFECHA, except the signal-to-noise ratio, which was computed using ARSTAN.
The dependence between radial growth and height growth was studied by cross-correlation analysis. These analyses were based on the residual chronologies and were calculated both with and without a time lag of one year.
Response to extreme climatic events
To study the growth response to extreme climatic episodes we analyzed the most important reductions in growth. Negative pointer years were determined as those years in which at least 75% of the growth series for each group of chronologies showed a growth decrease of at least 40% relative to the average growth in the 4 preceding years ([25]). This allowed the characterization of the following negative pointer years: 1896, 1945 and 2005 in the basal tree-ring series; 1896 and 2005 in the 1.3 m tree-ring series; 1918, 1945 and 2005 in the 8 m series; 1945 and 2005 in the 12 m series; only 2005 in the 15 m series and 2006 in the height increment series.
The growth response to the pointer years 1896, 1918, 1945 and 2005 and the ability to recover pre-disturbance growth levels after disturbance were estimated for the different groups of increment series through the resistance (Rt) and resilience (Rs) indices ([16] - eqn. 1, eqn. 2):
where Gi is the growth of year i and i-4 and i+4 are 4-year-long pre- and post-disturbance periods based on previous studies ([9]). For the pointer year 2005 we only used a 3-year-long post-disturbance period as we only had growth data up to 2008. As shoot length is heavily influenced by bud formation in the previous year of growth, instead of calculating the Rt of the pointer year 2006 in the height increment series, we calculated the Rt of 1945 and 2005 with a time lag of one year (t+1), shifting the numerator for growth of one year after the disturbance but maintaining a constant denominator. In addition, the Rs index of the height increment series was calculated without the first post-disturbance year, that is, with a period of only 3 years (1947-1949) after the pointer year of 1945 and 2 years (2007-2008) after the pointer year of 2005. The pointRes package ([30]) was used to calculate pointer years and resilience indices. We compared index values among groups of chronologies using the t-test for independent samples with different variances.
Climate-growth relationships
Relationships between growth and climate were analyzed through the software DendroClim 2002 ([1]) using Pearson’s correlation coefficients between the residual chronologies and the monthly precipitation / monthly mean temperature. As regards radial growth, correlation coefficients were calculated from September of the previous year to November of the year of growth analyzed, as the climate in autumn can influence the growth of the following year. Furthermore, in favorable years there may be a second vegetative stage between the end of summer and the middle of autumn ([6]). With respect to the height increment series, this interval was extended to the entire previous year for shoot elongation, because the corresponding terminal buds in conifers are pre-formed the year before and strongly influence final shoot length ([23], [12]).
Results and discussion
Height and diameter increment chronologies
Average correlations of the growth series with their master reference series ranged between 0.79 and 0.89 for tree-ring width series, and 0.61 in the case of height increment series with p<0.001 (Tab. 1), reflecting good to excellent inner coherence of the data set ([10], [2], [23]). The relatively high MS values suggest that the tree-ring widths accurately reflect causal factors (climate) and signal-to-noise ratio values suggest that the proportion of explainable variation due to causal factors is sufficient ([2], [28]). Therefore, all growth series were appropriate for studying their relationship with climate.
Tab. 1 - Statistical parameters of the chronology series. Signal-to-noise ratio of the 15 meter tree-ring chronology is missing because of the short time span.
Chronology | Type | Tree-ring width series | Height increment series |
||||
---|---|---|---|---|---|---|---|
Height (m) | Basal | 1.3 | 8 | 12 | 15 | ||
First year | 1871-81 | 1878-90 | 1909-20 | 1932-55 | 1961-91 | 1930 | |
Correlation with master | 0.79 | 0.79 | 0.84 | 0.88 | 0.89 | 0.61 | |
Standard deviation | 0.42 | 0.43 | 0.42 | 0.46 | 0.53 | 0.57 | |
Autocorrelation | 0.85 | 0.85 | 0.83 | 0.58 | 0.23 | 0.27 | |
Mean sensitivity | 0.27 | 0.27 | 0.25 | 0.26 | 0.31 | 0.36 | |
Signal-to-noise ratio | 11.34 | 14.4 | 16.79 | 32.14 | - | 9.76 | |
First year | 1961 | 1961 | 1961 | 1961 | 1961-91 | 1961 | |
Correlation with master | 0.84 | 0.84 | 0.85 | 0.9 | 0.89 | 0.57 | |
Standard deviation | 0.49 | 0.5 | 0.47 | 0.49 | 0.53 | 0.59 | |
Autocorrelation | 0.53 | 0.48 | 0.58 | 0.52 | 0.23 | 0.19 | |
Mean sensitivity | 0.28 | 0.29 | 0.28 | 0.27 | 0.31 | 0.38 |
The raw chronologies of tree-ring width and height increment showed a time lag of one year between maximum and minimum values (Fig. 1). Equally, the higher correlations between radial and height increment series were produced with the same time lag (Tab. 2).
Tab. 2 - Correlation coefficients between residual chronologies of basal and 15 m tree-ring width and height increment series. The level of significance (p-value) is in parentheses. t+1 has been calculated with a time lag of one year. (*): p < 0.05; (**) : p < 0.01; (***): p < 0.001.
- | Basal | 15 m | Height | Height t+1 |
---|---|---|---|---|
Basal | 1 | - | - | - |
15 m | 0.850*** (0.000) |
1 | - | - |
Height | 0.051 (0.740) |
0.252 (0.095) |
1 | - |
Height t+1 | 0.480** (0.001) |
0.514*** (0.000) |
0.095 (0.537) |
1 |
Height increment series presented higher values for sensitivity and standard deviations and scarce autocorrelation, which is concordant with previous studies ([23]). However, they also showed lower correlations with their master reference series, suggesting that their high variability may be due to genetic or microsite differences rather than to a better response to climatic variations.
The 15 m tree-ring series showed the highest values of MS and correlation with the master reference series and the lowest autocorrelation value (Tab. 1). Likewise, the signal-noise ratio increased with sample height of the tree-ring series. Therefore, the response of radial growth to climatic variations increased with height along the stem, as previously reported by other studies ([4], [14], [15]).
Resistance and resilience to severe droughts
In this regard we only have climate data for the pointer years 1945 and 2005. Like 2005, 1945 was one of the driest of the climate series with total precipitation of 179 mm between February and September. The growth response to the pointer years was similar in all tree-ring series (Fig. 2) except in the 15 m series, which had significantly higher Rt values for the pointer year 2005 (p = 0.002). Bouriaud et al. ([3]) also highlighted the lesser effect of drought on the upper part of the stem. Similarly, height increment series showed significantly higher Rt values for the pointer year 2005 (p < 0.001). The Rt values of the height increment series for the pointer years 1945 (t+1) and 2005 (t+1), both with a time lag of one year, also presented significant differences with regard to the tree-ring series with a time lag of one year (p < 0.001 in both), but showed no differences with regard to Rt values of the tree-ring series for 1945 and 2005 without one year time lag. In contrast to other species ([21]) the declines in height increment series were found to be no greater than those of tree-ring series. Therefore, our results do not support the hypothesis that the tree may change its allocation pattern from crown expansion and carbon assimilation to root extension in order to capture additional water ([19]).
Fig. 2 - Resistance and resilience recovery indices for all groups of chronologies considering the selected negative pointer years (1896, 1918, 1945 and 2005). Resistance is also shown with a time lag of one year (t+1) for the 1945 and 2005 pointer years.
No significant differences were found in Rs between series. Like previous studies, Rs values gradually rise over time, with better growth recoveries as age increases ([9]). In general, complete tree growth recovery is not reached within the four post-disturbance years. This is usually the case with conifers growing in mesic sites ([18]) as opposed to conifers adapted to xeric sites, which recover pre-drought growth more rapidly ([17], [9]). According to our results, extrapolating a given decline in increment at breast height to the whole stem can result in errors; on the one hand underestimating the total height increment decline (which occurs over two consecutive years following drought) and on the other, overestimating the decline in growth of the tree-ring series at 15 m.
Climatic drivers of height and diameter increments
Correlation with climatic variables differed for height increment and tree-ring width. In the case of the latter, this correlation also differed depending on the height at which the sample was taken (Tab. 3). We found significant (p < 0.05) positive responses of height increment to rainfall just before the start of the growing season, both in the previous year (bud formation) and in the year in which the studied shoot elongation takes place. Rainfall in September of the previous year was also significantly correlated, possibly because it influences bud formation which finishes during autumn ([12]). Tree-ring width seems to be mainly related to the amount of precipitation from November to July, which replenishes the soil water reserves up to the summer, as reported in similar studies conducted under Mediterranean climatic conditions ([2], [6]). The correlation with tree-ring width was positive for each month from November to July, although there were some differences in the significance between chronologies in the months from February onwards, depending on the sample height. The correlations with precipitation in the case of the upper stem begin to be significant (p < 0.05) in spring, whereas for basal radial growth, this occurs in winter. During the period of crown activity, which coincides with spring and summer in the Mediterranean climate ([12]), it is possible that most of the nutrients are used by the crown and the part of the stem which is closest to the crown, while the lower stem benefits from stored carbon ([8]). The low correlation between ring width at the stem base and precipitation during the active crown period, as well as the high correlation with the precipitation over the previous months may be due to a dependency of the cambium on carbon reserves collected during these months ([8]).
Tab. 3 - Pearson’s correlation coefficients of the residual chronologies with monthly precipitations (P) and monthly mean temperatures (T). Asterisks mark the statistically significant (p < 0.05) correlation coefficients with a 95% confidence interval. Residual chronologies: Height is the height increment chronology; Basal, 1.30 m, 8 m, 12 m, 15 m are the tree-ring width chronologies.
Year | Month | Residual Chronology vs. P | Residual Chronology vs. T | ||||||||||
---|---|---|---|---|---|---|---|---|---|---|---|---|---|
Height | 15 m | 12 m | 8 m | 1.30 m | Basal | Height | 15 m | 12 m | 8 m | 1.30 m | Basal | ||
Previous year | Jan | 0.11 | - | - | - | - | - | 0.02 | - | - | - | - | - |
Feb | 0.35* | - | - | - | - | - | 0.18 | - | - | - | - | - | |
Mar | 0.15 | - | - | - | - | - | 0.13 | - | - | - | - | - | |
Apr | 0.13 | - | - | - | - | - | 0 | - | - | - | - | - | |
May | 0.07 | - | - | - | - | - | -0.03 | - | - | - | - | - | |
Jun | -0.01 | - | - | - | - | - | -0.03 | - | - | - | - | - | |
Jul | 0.17 | - | - | - | - | - | 0.03 | - | - | - | - | - | |
Aug | -0.02 | - | - | - | - | - | 0.17* | - | - | - | - | - | |
Sep | 0.24* | 0.07 | 0 | 0.05 | -0.08 | -0.08 | 0.21* | 0 | 0.02 | 0.06 | -0.01 | -0.03 | |
Oct | 0.03 | 0.03 | -0.05 | 0 | -0.05 | -0.08 | 0.01 | -0.01 | -0.07 | -0.13 | -0.1 | -0.09 | |
Nov | -0.11 | 0.31* | 0.24* | 0.25* | 0.28* | 0.34* | -0.1 | 0.24 | 0.19 | 0.16 | 0.27* | 0.30* | |
Dec | -0.1 | 0.25* | 0.19* | 0.21* | 0.23* | 0.27* | -0.09 | 0.2 | 0.28* | 0.30* | 0.37* | 0.39* | |
Growth year | Jan | 0.19 | 0.39* | 0.37* | 0.41* | 0.37* | 0.37* | 0.11 | 0.31* | 0.29* | 0.30* | 0.32* | 0.35* |
Feb | 0.07 | 0.1 | 0.18 | 0.21* | 0.21* | 0.23* | -0.02 | 0.43* | 0.35* | 0.32* | 0.33* | 0.35* | |
Mar | 0.22* | 0.15 | 0.25* | 0.26* | 0.25* | 0.27* | 0.05 | -0.01 | 0.12 | 0.08 | 0.05 | 0.07 | |
Apr | 0.16 | 0.34* | 0.25* | 0.25* | 0.16 | 0.12 | -0.07 | 0.32* | 0.05 | 0.06 | 0.16 | 0.17 | |
May | 0.05 | 0.35* | 0.34* | 0.31* | 0.34* | 0.30* | 0.03 | -0.28* | -0.38* | -0.38* | -0.38* | -0.32* | |
Jun | -0.02 | 0.29* | 0.14 | 0.17 | 0.17 | 0.17 | -0.01 | -0.22 | -0.14 | -0.21 | -0.17 | -0.15 | |
Jul | 0.03 | 0.25 | 0.28* | 0.25* | 0.27* | 0.30* | -0.25* | -0.35* | -0.12 | -0.21* | -0.1 | -0.05 | |
Aug | -0.1 | -0.17 | -0.1 | -0.13 | -0.09 | -0.05 | -0.07 | 0.11 | 0.09 | 0.02 | 0.04 | 0.08 | |
Sep | 0.08 | -0.09 | 0.13 | 0.08 | 0.13 | 0.21 | -0.13 | 0.42* | 0.26* | 0.23* | 0.27* | 0.26* | |
Oct | -0.12 | -0.1 | -0.12 | -0.06 | -0.09 | -0.04 | -0.03 | 0.04 | -0.06 | -0.09 | 0 | -0.02 | |
Nov | 0.05 | -0.17 | -0.1 | -0.09 | -0.15 | -0.12 | -0.13 | -0.27* | -0.28* | -0.24* | -0.21 | -0.17 |
Height increment was directly correlated with late summer temperature in the previous year of growth and low July temperature of the current year. Tree-ring width was positively correlated with winter and late summer temperatures and negatively correlated with May and July temperatures (Tab. 3). These results highlight the importance of late summer temperature for the growth of P. pinaster at the study site, which may present a bimodal pattern of growth with reactivation in late summer ([12]). Temperature seems to be more important for growth than reported in other studies on the same species at nearby sites ([2]), possibly because climate trends at local level can differ from regional trends, due to the influence of the topography, slope, winds or other local factors that add local variability to climatic trends. The relationships with temperature were similar for all ring-width samples with the exception of the 15 meter series, which showed higher correlations in the active crown period during spring and summer ([12]), and also with the exception of the previous autumn, as there was a higher correlation with stem base growth, which may be due to its dependency on the carbon reserves collected over these months.
Conclusions
Our results reveal that height increment and radial growth responses to climatic variability in the middle and especially upper stem differ from the radial growth response at breast height, the latter being less sensitive to climate. If we only consider growth at breast height, the influence of late summer climatic conditions or disturbances such as droughts on tree growth may be underestimated, leading to lower accuracy when estimating the impact of climate change on tree productivity, carbon dioxide capture and adaptation of tree species. Furthermore, these results could contribute to the development of appropriate forest management practices aimed at adapting to and mitigating the effects of climate change.
The results of this study are of local value for Pinus pinaster. Similar studies with other species and/or sites need to be conducted to obtain more generally applicable results.
Acknowledgments
The study was partially supported by the projects AGL2011-29701-C02.01 and AT10-007. We also acknowledge the Spanish State Meteorological Agency (AEMET), Ministry of Agriculture, Food and Environment, for granting access to the meteorological data.
References
Gscholar
CrossRef | Gscholar
CrossRef | Gscholar
Authors’ Info
Authors’ Affiliation
Departamento de Sistemas y Recursos Naturales, Escuela Técnica Superior de Ingeniería de Montes, Forestal y del Medio Natural, Universidad Politécnica de Madrid, Ciudad Universitaria s/n, E-28040 Madrid (Spain)
Sven Mutke
Miren Del Río
INIA, Forest Research Centre, Department of Silviculture and Forest Management, Crta. La Coruña km 7.5, E-28040 Madrid (Spain)
Sven Mutke
Miren Del Río
iuFOR, Sustainable Forest Management Research Institute, University of Valladolid & INIA (Spain)
Department of Biogeography and Global Change, National Museum of Natural Sciences, Spanish National Research Council (MNCN, CSIC), Madrid (Spain)
Corresponding author
Paper Info
Citation
Rubio-Cuadrado Á, Bravo-Oviedo A, Mutke S, Del Río M (2018). Climate effects on growth differ according to height and diameter along the stem in Pinus pinaster Ait.. iForest 11: 237-242. - doi: 10.3832/ifor2318-011
Academic Editor
Francesco Ripullone
Paper history
Received: Dec 15, 2016
Accepted: Jan 15, 2018
First online: Mar 12, 2018
Publication Date: Apr 30, 2018
Publication Time: 1.87 months
Copyright Information
© SISEF - The Italian Society of Silviculture and Forest Ecology 2018
Open Access
This article is distributed under the terms of the Creative Commons Attribution-Non Commercial 4.0 International (https://creativecommons.org/licenses/by-nc/4.0/), which permits unrestricted use, distribution, and reproduction in any medium, provided you give appropriate credit to the original author(s) and the source, provide a link to the Creative Commons license, and indicate if changes were made.
Web Metrics
Breakdown by View Type
Article Usage
Total Article Views: 47065
(from publication date up to now)
Breakdown by View Type
HTML Page Views: 39967
Abstract Page Views: 3029
PDF Downloads: 3175
Citation/Reference Downloads: 19
XML Downloads: 875
Web Metrics
Days since publication: 2576
Overall contacts: 47065
Avg. contacts per week: 127.89
Article Citations
Article citations are based on data periodically collected from the Clarivate Web of Science web site
(last update: Mar 2025)
Total number of cites (since 2018): 16
Average cites per year: 2.00
Publication Metrics
by Dimensions ©
Articles citing this article
List of the papers citing this article based on CrossRef Cited-by.
Related Contents
iForest Similar Articles
Research Articles
The effect of provenance of historical timber on tree-ring based temperature reconstructions in the Western Central Alps
vol. 13, pp. 351-359 (online: 25 August 2020)
Research Articles
Response to climate and influence of ocean-atmosphere phenomena on annual radial increments of Pinus oocarpa Schiede ex Schltdl. & Cham in the Lagunas de Montebello National Park, Chiapas, Mexico
vol. 16, pp. 174-181 (online: 30 June 2023)
Research Articles
Impact of climate change on radial growth of Siberian spruce and Scots pine in North-western Russia
vol. 1, pp. 13-21 (online: 28 February 2008)
Research Articles
Influence of tree density on climate-growth relationships in a Pinus pinaster Ait. forest in the northern mountains of Sardinia (Italy)
vol. 8, pp. 456-463 (online: 19 October 2014)
Research Articles
Individual-based approach as a useful tool to disentangle the relative importance of tree age, size and inter-tree competition in dendroclimatic studies
vol. 8, pp. 187-194 (online: 21 August 2014)
Research Articles
Growth-climate relations and the enhancement of drought signals in pedunculate oak (Quercus robur L.) tree-ring chronology in Eastern Hungary
vol. 11, pp. 267-274 (online: 29 March 2018)
Research Articles
Role of photosynthesis and stomatal conductance on the long-term rising of intrinsic water use efficiency in dominant trees in three old-growth forests in Bosnia-Herzegovina and Montenegro
vol. 14, pp. 53-60 (online: 28 January 2021)
Research Articles
Contrasting resin-tapped and untapped Pinus pinaster Ait. trees of central Spain in a dendroclimatic research
vol. 17, pp. 148-155 (online: 30 May 2024)
Review Papers
Increasing resistance and resilience of forests, a case study of Great Britain
vol. 17, pp. 69-79 (online: 21 March 2024)
Research Articles
Species-specific climate response of oaks (Quercus spp.) under identical environmental conditions
vol. 7, pp. 61-69 (online: 18 November 2013)
iForest Database Search
Search By Author
Search By Keyword
Google Scholar Search
Citing Articles
Search By Author
Search By Keywords
PubMed Search
Search By Author
Search By Keyword