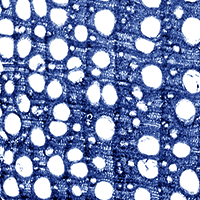
Anatomical and genetic aspects of ash dieback: a look at the wood structure
iForest - Biogeosciences and Forestry, Volume 10, Issue 2, Pages 522-528 (2017)
doi: https://doi.org/10.3832/ifor2080-010
Published: Apr 20, 2017 - Copyright © 2017 SISEF
Research Articles
Abstract
Pathogen diseases are increasingly threatening forest trees under the current climate change, causing a remarkable decrease in the stability of forest ecosystems. Ash (Fraxinus excelsior L.) dieback due to Hymenoscyphus fraxineus has been noted in Poland since 1992 and has spread over many European countries. The aim of this study was to test the hypothesis that ash trees affected by dieback could exhibit a reduced vessel size and density along the trunk, as well as a lowered width of annual wood rings, leading to the weakening of water transport towards the crown. Dead and dying ash trees were sampled in a forest district severely affected by ash decline in southern Poland. Wood samples were collected at different height along the trunk and several wood anatomical characteristics of annual tree rings over the period 2002-2011 were examined. Dead trees showed a stronger reduction in radial growth than dying trees over the period considered. Moreover, the diameter of vessels increased from the crown to the base in both dead and dying trees, while the density decreased. Significant differences between dead and dying trees were detected in size and density of vessels in the period analyzed, as well as in the width of annual rings. DNA extracted from wood samples was analyzed using SSR markers and the main genetic parameters of dead and dying trees were estimated, finding similar levels of polymorphism and only slight non-significant differences between the two health groups. Our results are consistent with the hypothesis that pathogens could disrupt the hormonal control of wood formation by interfering with the polar auxin transport, progressively leading to the death of ash trees.
Keywords
Ash Trees, Microsatellites Markers, Tree Decline, Wood, Vessel Size and Density
Introduction
European ash (Fraxinus excelsior L.) is spread almost throughout Europe, with the exception of central and southern Spain, northern part of the Scandinavian peninsula, Iceland and northern regions of the British Isles ([26]). It is most common in lowland forests, but in the submontane and lower montane layers it usually occurs in the alder-ash or elm-ash forests from the alliance Alno-Ulmion. The ecological significance of ash has recently increased because of the Dutch elm disease ([4]) as well as alder dieback caused by microorganisms belonging to Phytophtora genus ([5]). However, the first symptoms of ash decline were noticed in Poland since 1992, especially in the north-east region ([32]), and rapidly spread across Poland ([20]) over an area of more than 10.000 hectares ([11]). Today, the ash decline has become chronic and was observed not only in most European countries ([29]) but also in Asia and North America ([6], [39]). Currently, it is assumed that ash dieback is due to Hymenoscyphus fraxineus Baral, Queloz & Hosoya ([3]) (= anamorphe Chalara fraxinea T. [20]), a novel species alien to Poland ([19]). A broad range of symptoms resulting from ash dieback disease involves all parts of the tree, including necrotic lesions and cankers along the bark of branches and stem, wilt and discoloration of foliage, dieback of shoots, twigs or trunk, as well as epicormic branching or excessive side shoots along the trunk ([2], [18]). Discoloration of the bark was also observed ([34]).
Ash tree has a ring-porous wood and vessel formation is controlled by a mechanism associated with the polar transport of auxin ([17], [30], [22]), which is conditioned by the asymmetrical distribution of auxin carriers on the cell membrane ([24]). Auxin is thought to be produced by developing buds and young growing leaves and its effect on vessel differentiation can be altered by providing exogenous auxin ([8], [42]). Aloni & Zimmermann ([1]) observed that vessel size in wood increases from leaves to roots, while their density decreases, and ascribed this pattern to a decreasing gradient of auxin concentration downwards. Based on the above hypothesis, high auxin levels close to young leaves induce a rapid differentiation and the formation of small vessels. Conversely, low concentrations of auxin result in a slow differentiation and the formation of wider vessels. Wolpert ([40]) proposed that concentration gradients of morphogens are important for the regulation of developmental events in animals. Under this assumption, cells develop in relation to their position along a concentration gradient of a morphogen. This assumption has also been adopted for plants as a mechanism able to coordinate the development of plant cells. It stipulates that auxin, whose concentration decreases in basipetal direction, could act as a positional signal for the differentiation of cambial derivatives as new vessels ([37]).
A well-developed set of vessels ensures the proper transport of water to all tree organs and allows for their survival in unfavorable environmental conditions. In ring-porous species like ash the vessels involved in the transport of water are those located in the earlywood of the current year ([9]) which loose their functionality by the end of each winter ([43]). The contribution of earlywood vessels to water transport in ring-porous species is approx. 90% ([7]).
The foliage of ash trees subjected to decline is strongly affected by Hymenoscyphus fraxineus and other secondary harmful agents, though varying levels of the disease symptoms could be observed. Such variation in the susceptibility of trees to decline may be hypothesized to reflect changes in water transport along the trunk and/or to genetically-encoded resistance to the fungus.
In this study we aimed to test the following working hypotheses: (1) ash trees affected by decline have a reduced radial increment, with decreasing annual tree ring width and variation along the trunk of the size and density of tracheary elements, according to Aloni & Zimmermann ([1]); (2) different disease symptoms may be due to differences in the genetic make-up of ash trees. To test the above hypotheses, we focused on the following traits: (i) width of annual tree rings; (ii) number of earlywood vessels along the boundary of annual growth ring; (iii) diameter, density and surface area of earlywood vessels; (vi) ratio of the surface area of earlywood vessels to the width of annual tree ring. To better characterize the differences among trees, DNA analysis of the individuals sampled for wood analysis was also carried out.
Material and methods
The survey was performed on ash trees (Fraxinus excelsior L.) growing in a natural forest stands of the Gidle Forest District, southern Poland (50° 54′ 39.3″ N, 19° 44′ 47.5″ E). This area is severely affected by ash decline (Forest Management Plan) and ash trees with no symptoms have not been observed. The trees selected for sampling were all co-dominant in the canopy layer (II class according to Kraft’s biosocial classification) and were cut in May 2012. Wood disks were taken at four different height along the trunk: (i) breast height (1.3 m above the ground, referred to as H1); (ii) 10 m (H2); (iii) 2 m below the crown base (H3); and (iv) close to the crown base (H4). Sampled trees were classified in two health classes based on the presence of cambium necrosis and their defoliation level: dying trees (control group) with clear symptoms of dieback, and dead trees. We sampled 3 individuals for each health class, totaling 24 wood disk samples (Tab. 1).
Tab. 1 - Mean biometric traits (± standard deviation) of the European ash trees sampled for anatomic and genetic analyses, grouped by health status of the trees. (ABH): Age at the breast height (years); (DBH): diameter at breast height (cm); (HCB): height of the crown base (m).
Health class | Symptoms of dieback | ABH (yrs) |
DBH (cm) |
HCB (m) |
---|---|---|---|---|
dying | defoliating - 70%, top dry or foliage wilting | 60 ± 6.93 | 26 ± 2.08 | 20 ± 3.47 |
dead | cambium necrosis, withered branches without leaves | 75 ± 0.58 | 28 ± 0.58 | 21 ± 2.31 |
Wood samples processing and variable measures
Small samples of wood including the annual tree rings were cut from the disks along a radial direction. Wood sections were then prepared using standard protocols ([12]). After the softening process, 30 µm thick cross sections were cut with the use of a sliding microtome (HM 440E®, Carl Zeiss, Germany). The sections were transferred onto slides, mounted with glycerol and then analyzed under a light microscope (Olympus BX61®, Olympus Corp., Center Valley, PA, USA), equipped with a motorized table, and a digital microscope camera (Olympus DP 70®). Image acquisition and measurements were carried out using the software Olympus Cell P®. For each disk and each tree, the last 10 annual rings were considered. We measured the width of annual tree rings, and the diameter and density of earlywood vessels in the rings. Measures were averaged over each health class for the following variables: (i) the width of annual tree rings; (ii) the number of earlywood vessels along the boundary of annual tree rings (amounting to 6.65 mm for each ring); (iii) the diameter of earlywood vessels (overall 4800 vessels); (iv) the density of earlywood vessels (number of vessels per 1 mm2 of annual increment; (v) the surface area of earlywood vessels; (vi) the ratio of the surface area of earlywood vessels to the width of annual tree ring (expressed in %). The annual tree rings formed during the course of ash dieback were mainly composed of earlywood, as latewood zones were diminished or absent; therefore only the diameter of earlywood vessels was examined.
The mean value of each variable was calculated for each annual tree ring over the calendar years 2002-2011 and for all rings at the selected heights (H1, H2, H3, H4). Differences between health classes for each variable were tested using an independent samples t-test, with p ≤ 0.005 as threshold for the significance of differences.
Genetic analyses
A preliminary analysis of genetic diversity based on neutral microsatellite markers was carried out on 6 trees representing the two health classes (3 dead trees and 3 dying trees). DNA was extracted from wood samples using the NucleoSpin® Plant II kit (Macherey-Nagel, Düren, Germany), following the manufacturer’s protocol with small modifications (i.e., the volume of the three buffers was changed: 600 µl of PL2, 150 µl of PL3 and 900 µl of PC buffer). Six nuclear microsatellite and four chloroplast loci were amplified in two multiplexes, using the Multiplex PCR® Kit (Qiagen, Hilden, Germany). In multiplex A the amplified loci were: Femsatl-4, Femsatl-8, Femsatl-19, ccmp3, ccmp6; in multiplex B were Femsatl-11, Femsatl-16, M2-30, ccmp7, and ccmp10 (for details on primer sequences, see [33]). PCR reactions were carried out in 10 µl reaction volumes with 5-50 ng DNA, 10 pmol of each primer, 5 µl Multiplex PCR Master Mix® (Qiagen). Each forward primer was labelled with fluorescent WellRED® dyes (Beckman Coulter Inc., Brea, CA, USA). The PCR thermal profile followed the protocols used by Sutherland et al. ([33]) for both multiplexes. Allele lengths were scored on the CEQ 8000® sequencer (Beckman Coulter, Inc.). Parameters of genetic variation were computed for each health class (dead and dying trees) using the software GenAlEx v.6.502 ([27]). Differences in genetic parameters between health classes and among trees were tested by the analysis of molecular variance (AMOVA) using GenAlEx v.6.502 (999 permutations, p = 0.01).
Results
Morpho-anatomical analysis of wood variation
In both health classes, the annual tree rings formed during the progress of ash dieback comprised a well-developed zone of earlywood, whereas the zone of latewood was diminished (Fig. 1). Also, a decrease in the width of annual tree rings in acropetal direction (from the base of the trunk toward the crown) was observed (Fig. 2a). This trend was significantly more pronounced in dead trees, in which the disease process was more advanced. In the last ten years, a reduction in the width of annual tree rings (expressed as the mean over the trunk) was observed, decreasing from 1850 μm in 2002 to 700 μm in 2011 and from 1100 μm to 400 μm in dying and dead trees, respectively (Fig. 2b).
Fig. 1 - Cross section of wood of a dying ash tree taken at 1.3 m above the ground. A reduction in the extension of latewood can be noticed for more recent annual rings (on the left).
Fig. 2 - Variation in width of annual tree rings in the health classes of ash trees affected by dieback (white bars: dying trees; grey bars: dead trees). (a): different heights along the trunk (H1: 1.3 m above the ground; H2: 10 m above the ground; H3: 2 m below the crown base; H4: close to the crown base); (b): different years over the period 2002-2011.
The number of vessels along the boundary of the tree rings increased from the base of the trunk towards the crown (Fig. 3a). This increase was significantly lower in dead trees compared to dying ones (Tab. 2). The number of vessels in the annual tree ring (averaged over the trunk) increased in the last 10 years, from over twenty to more than thirty in dying trees, and from a dozen to more than twenty in dead trees (Fig. 3b).
Fig. 3 - Number of vessels of earlywood along the boundary of annual growth (a, b) and vessel density of earlywood within annual increments (c, d) in the health classes of ash trees affected by dieback (white bars: dying trees; grey bars: dead trees). (a, c): different heights along the trunk (H1: 1.3 m above the ground; H2: 10 m above the ground; H3: 2 m below the crown base; H4: close to the crown base); (b, d): different years over the period 2002-2011.
Tab. 2 - Differences in wood characteristics between the two health classes (dead and dying) of ash trees affected by dieback after the Student’s t-test. (*): p<0.05.
Wood characteristics | Health class |
Mean | Difference | t-statistics |
---|---|---|---|---|
Mean width of the annual tree rings (mm) |
Dead trees | 670.92 | 388.08 | 6.799* |
Dying trees | 1059.00 | |||
Number of vessels (n) | Dead trees | 21.83 | 4.96 | 5.098* |
Dying trees | 26.79 | |||
Diameter of vessels (mm) | Dead trees | 185.81 | 7.36 | 2.010* |
Dying trees | 193.17 | |||
Density of vessels (n mm-2) | Dead trees | 6.64 | 1.92 | 3.941* |
Dying trees | 4.72 | |||
Sectional area of earlywood vessels (mm2) |
Dead trees | 0.63 | 0.16 | 5.249* |
Dying trees | 0.79 | |||
Ratio of surface area of earlywood vessels to the width of the annual tree ring (%) | Dead trees | 19.20 | 5.79 | 4.252* |
Dying trees | 13.41 |
The earlywood vessel density (the number of vessels per 1 mm2 of annual tree ring) increased from the base of the trunk towards the crown. There was a significantly higher vessel density in dead trees compared to dying trees, from 4.8 to 8.7 and from 3.7 to 6.3 per mm2, respectively (Fig. 3c, Tab. 2). In the last 10 years the mean vessel density in annual tree rings (averaged over the trunk) increased steadily, reaching in the last year (2011) a value of 13.1 per mm2 in dead trees and 8 per mm2 in dying ashes (Fig. 3d).
The diameter of the vessels along the trunk decreased in dying trees from 221 μm at breast height to 150 μm at the base of the crown. In dead trees this trend was similar, with the largest diameters (206 μm) observed 10 m below the crown (Fig. 4a). However, the vessel diameter under the crown was higher in dead trees and reached a mean value of 167 μm. In the last 10 years the mean vessel diameters (expressed as a mean for the trunk) fluctuated in both dying and dead trees, ranging from 202 to 179 μm and from 196 to 168 μm in dying and dead trees, respectively (Fig. 4b).
Fig. 4 - Diameter of earlywood vessels (a, b), cross sectional area of earlywood vessels (c, d) and the ratio of surface area of earlywood vessels to the width of the annual tree ring (e, f) in the health classes of ash trees affected by dieback (white bars: dying trees; grey bars: dead trees). (a, c, e): different heights along the trunk (H1: 1.3 m above the ground; H2: 10 m above the ground; H3: 2 m below the crown base; H4: close to the crown base); (b, d, f): different years in the period 2002-2011.
The sectional area of earlywood vessels along the trunk in annual tree rings tends to reduce moving upwards toward the crown, with the highest values found at the height of 10 m below the crown (0.96 mm2 for dying trees and 0.75 mm2 for dead ashes, respectively - Fig. 4c, Tab. 2). In the last 10 years a higher cross-sectional area (mean over the trunk) was found in dying trees as compared with dead ashes. However, significant differences occurred in 2002-2004, 2006 and 2008 only (Fig. 4d).
The ratio of surface area of earlywood vessels to the width of the annual tree ring along the trunk fluctuated in the period considered (Fig. 4e). However, in dying trees it was significantly lower than in dead trees (Tab. 2). It was found to be the lowest under the canopy in dying trees, while in dead trees it was the lowest at breast height. During the last 10 years, the mean ratio of surface area of earlywood vessels to the width of the annual tree ring (mean for the trunk) increased more in dead trees than in dying ashes, and this trend was especially noticeable in the last 5 years of their lifespan (Fig. 4f).
Genetic analysis of wood
Based on the results of microsatellites analysis, we did not observe any polymorphism in the chloroplast loci. However, single private alleles have been detected for nuclear SSR loci, depending on the analyzed group of individuals (Fig. 5). The damaged trees had lower observed and expected number of alleles per locus (Tab. 3). Also, the number of private alleles was slightly higher in dead trees compared to the damaged trees (Ap = 1.883 and 1.5, respectively). The observed heterozygosity in the two health classes was the same (Ho = 0.889), but the expected heterozygosity was higher in dead than in damaged trees (He = 0.685 and 0.639, respectively). The results of the AMOVA revealed that the two health classes of trees were not significantly divergent in terms of their genetic make-up (FST = 0.066; p>0.05 - data not shown).
Fig. 5 - Alleles frequency for 6 nuclear microsatellite loci examined in the health classes of ash trees affected by dieback (white bars: dying trees; grey bars: dead trees).
Tab. 3 - Estimates of genetic diversity parameters for dead and dying ash trees affected by dieback. (na, ne): observed and expected allele number; (Ap): private alleles; (I): information index; (Ho, He): observed and expected heterozygosity.
Health class | n a | n e | A p | I | H o | H e |
---|---|---|---|---|---|---|
Dead trees | 4.000 | 3.500 | 1.833 | 1.277 | 0.889 | 0.685 |
Dying trees | 3.667 | 2.979 | 1.500 | 1.156 | 0.889 | 0.639 |
Discussion
In this study, a marked modification of wood biometric traits was observed in ash trees affected by decline over the last 10 years of their lifespan. As expected, such changes were more pronounced in trees showing a more advanced disease process like the dead ash trees. The analysis of the width of annual tree rings along the trunk showed that the decline in radial growth is more prominent in the upper part of the trunk (below the crown) and gradually decreases moving toward the base. Further, we observed a more significant progression of the disease through time in dead ash trees as compared with dying trees. The above evidence might suggest that the weakening of water transport along the trunk could be involved in the dying of ash trees in the investigated area. Indeed, ash has ring-porous wood with large vessels in the earlywood and a very efficient conductive system; according to Hagen-Poisseuille’s law, the amount of transported water is proportional to the fourth power of the vessel diameter ([36], [35]). On the other hand, their large vessels are exposed to embolism and cavitation which take place during the winter ([13], [21]).
The formation of a suitable network of vessels during the annual production of wood rings is a necessary condition for an efficient water transport along the trunk. Our results suggests that dying trees produced smaller annual rings with reduced vessel size (Fig. 4a, Fig. 4b) and increased density (Fig. 3c, Fig. 3d). This results in the deterioration of water supply to the crown, thus weakening the growth of apical meristems and leaves. Moreover, our results are consistent with the hypothesis that the observed reduction in vessel size and annual rings could be due to a disruption of the hormonal control of wood formation (in terms of interference in the polar auxin transport), progressively leading to the death of trees. Indeed, auxin is produced in apical meristems and young leaves and transported in basipetal direction to roots, promoting wood formation and controlling the density and size of the conductive elements in wood ([1], [10]). Further, auxin strongly promotes the growth of roots ([41]), which in turn produce cytokinins that move in the acropetal direction and stimulate the crown development ([38]), with a mechanism of positive feedback.
Genetic studies performed on plus-tree clones of F. excelsior in Sweden demonstrated a considerable genotypic variation among individuals. None of the clones were totally resistant to Hymenoscyphus fraxineus, but some trees exhibited a reduced susceptibility and still showed some resistance after 6 years of heavy infection pressure ([31]). A study on the genetic variation in dieback resistance of Fraxinus excelsior carried out in Denmark using microsatellite markers ([23]) proved no correlation between the inheritance of alleles at the Femsatl loci and tree susceptibility in terms of necrosis developed after inoculation with Chalara fraxinea. In this study, the two health groups of ash trees affected by dieback were screened at the Femsatl loci, finding no differences in their allele frequencies. We obtained similar results from the analysis of 55 samples of the same species showing different signs of dieback at various stages of infection (unpublished data). Only two alleles, one from locus Femsatl-11 (allele 208) and the second from locus Femsatl-19 (allele 190), were found only in a group of more resistant individuals. However, based on the results of this investigation, microsatellite markers did not show any correlation with the level of resistance to ash dieback, consistently with the neutral nature of this class of molecular markers. Other kinds of DNA-based markers like SNPs seems more suitable to differentiate tolerant versus susceptible ash trees, as recently demonstrated by Harper et al. ([15]).
The interaction between European ash genotype and environmental conditions was previously assessed using RAPD markers in a Lithuanian population trial by Pliura & Baliuckas ([28]), who reported a high genetic variation within populations affecting the adaptive potential and competitive ability of the studied trees. High levels of genetic variation allows the ash population to maintain sufficiently high plasticity and adaptability to the changing environmental conditions. In this study, we found a fairly high (though not significant) levels of genetic divergence (FST = 0.066) between the two health groups of trees, even though they belong to the same population. Previous studies based on nuclear and chloroplast DNA markers showed a level of genetic divergence among Polish common ash populations higher to that found for Angiosperms (GST = 0.198 vs. 0.110, respectively - [14], [25]). Indeed, a transition zone between chloroplast microsatellite DNA haplotypes has been reported in Poland, which may suggest genotypic richness of common ash populations ([16]).
Further studies based on DNA polymorphism performed on larger groups of trees may help to understand the genetic basis of the pathogen tolerance developed by some common ash trees.
Conclusions
In this study, we investigated the variation of the wood structure along the trunk of ash trees affected by ash dieback. Our results revealed that: (i) the reduction in width of annual tree rings in dead trees is much more conspicuous in the upper part of the trunk; (ii) the number of earlywood vessels in the upper part of the trunk is significantly different between dead and dying trees. Moreover, our results are in agreement with the hypothesis proposed by Aloni & Zimmermann ([1]). The above evidences suggest that the ash dying process could start at the top of the trunk, perhaps in the crown, then moving towards its base. We hypothesized that this could be the consequence of the disruption of the hormonal control of wood formation due to the pathogen, leading to a restricted water supply to the crown and thus weakening the growth of apical meristems and leaves. However, further study of the root system of diseased trees are needed to better understanding such mechanism.
The genetic analysis of wood of dying and dead ash trees using both chloroplast and microsatellite markers did not reveal any correlation between tree genotype and phenotype. However, the high variability detected and the presence of private alleles suggests a high genetic variation possibly associated with a higher adaptive potential for ash trees in unfavorable environmental conditions.
Acknowledgments
MT conceived the study, carried out the anatomical analyses and helped to draft the manuscript; JZ carried out the field study and helped to draft the manuscript; JA performed the statistical analysis and helped to draft the manuscript; AT and JN carried out the genetic surveys and helped to draft the manuscript; BY helped to draft the manuscript.
The authors thank Ms. Aissa Zakrzewska for language revision. We acknowledge Mr. Jan Wisniewski and Mr. Mariusz Kielek’s assistance during wood samples collection.
Disclosure statement
No potential conflict of interest was reported by the authors.
References
Gscholar
Gscholar
CrossRef | Gscholar
Gscholar
Gscholar
Gscholar
Gscholar
Gscholar
Online | Gscholar
Gscholar
Gscholar
Authors’ Info
Authors’ Affiliation
Jacek Zakrzewski
Department of Forest Botany, Warsaw University of Life Sciences (SGGW-WULS), 159 Nowoursynowska St., 02-776 Warsaw (Poland)
Institute of Forest Science of Lodz University, 65/67 Konstytucji 3 Maja St. 97-200 Tomaszów Mazowiecki (Poland)
Justyna A Nowakowska
Laboratory of Molecular Biology, Forest Research Institute, 3 Braci Lesnej St., Sekocin Stary, 05-090 Raszyn (Poland)
Wood Anatomy and Dendrochronology Laboratory, Bartin University, Bartin (Turkey)
Corresponding author
Paper Info
Citation
Tulik M, Zakrzewski J, Adamczyk J, Tereba A, Yaman B, Nowakowska JA (2017). Anatomical and genetic aspects of ash dieback: a look at the wood structure. iForest 10: 522-528. - doi: 10.3832/ifor2080-010
Academic Editor
Vicente Rozas
Paper history
Received: Apr 08, 2016
Accepted: Jan 21, 2017
First online: Apr 20, 2017
Publication Date: Apr 30, 2017
Publication Time: 2.97 months
Copyright Information
© SISEF - The Italian Society of Silviculture and Forest Ecology 2017
Open Access
This article is distributed under the terms of the Creative Commons Attribution-Non Commercial 4.0 International (https://creativecommons.org/licenses/by-nc/4.0/), which permits unrestricted use, distribution, and reproduction in any medium, provided you give appropriate credit to the original author(s) and the source, provide a link to the Creative Commons license, and indicate if changes were made.
Web Metrics
Breakdown by View Type
Article Usage
Total Article Views: 50663
(from publication date up to now)
Breakdown by View Type
HTML Page Views: 42507
Abstract Page Views: 2851
PDF Downloads: 3976
Citation/Reference Downloads: 46
XML Downloads: 1283
Web Metrics
Days since publication: 2995
Overall contacts: 50663
Avg. contacts per week: 118.41
Article Citations
Article citations are based on data periodically collected from the Clarivate Web of Science web site
(last update: Mar 2025)
Total number of cites (since 2017): 8
Average cites per year: 0.89
Publication Metrics
by Dimensions ©
Articles citing this article
List of the papers citing this article based on CrossRef Cited-by.
Related Contents
iForest Similar Articles
Research Articles
Earlywood vessel features in Quercus faginea: relationship between ring width and wood density at two sites in Portugal
vol. 8, pp. 866-873 (online: 27 April 2015)
Research Articles
Variation of wood and bark density and production in coppiced Eucalyptus globulus trees in a second rotation
vol. 9, pp. 270-275 (online: 08 September 2015)
Short Communications
Effect of intensive planting density on tree growth, wood density and fiber properties of maple (Acer velutinum Boiss.)
vol. 9, pp. 325-329 (online: 22 October 2015)
Research Articles
Chloroplast microsatellites as a tool for phylogeographic studies: the case of white oaks in Poland
vol. 8, pp. 765-771 (online: 19 July 2015)
Research Articles
Individual-based approach as a useful tool to disentangle the relative importance of tree age, size and inter-tree competition in dendroclimatic studies
vol. 8, pp. 187-194 (online: 21 August 2014)
Research Articles
The effect of provenance of historical timber on tree-ring based temperature reconstructions in the Western Central Alps
vol. 13, pp. 351-359 (online: 25 August 2020)
Research Articles
Influence of tree density on climate-growth relationships in a Pinus pinaster Ait. forest in the northern mountains of Sardinia (Italy)
vol. 8, pp. 456-463 (online: 19 October 2014)
Research Articles
Tree growth, wood and bark water content of 28 Amazonian tree species in response to variations in rainfall and wood density
vol. 9, pp. 445-451 (online: 16 January 2016)
Research Articles
Physical, chemical and mechanical properties of Pinus sylvestris wood at five sites in Portugal
vol. 10, pp. 669-679 (online: 11 July 2017)
Research Articles
Wind contribution to yearly silver fir (Abies alba Mill.) compression wood development in the Romanian Carpathians
vol. 9, pp. 927-936 (online: 02 October 2016)
iForest Database Search
Search By Author
Search By Keyword
Google Scholar Search
Citing Articles
Search By Author
Search By Keywords
PubMed Search
Search By Author
Search By Keyword